Fig. 51.1
Mechanics of dynamic hyperinflation in acute severe asthma (Rotta and Steinhorn 2007). (a) A flow-time curve shows that inspiration begins before complete exhalation of the previous breath, leading to gas trapping (shaded area). (b) Gas trapping over the course of several breaths leads to an increased end-expiratory lung volume. The trapped volume over functional residual capacity is indicated by the double-headed arrow. (c) Increase in expiratory time and decrease in respiratory rate allow for complete exhalation prior to initiation of the next breath. (d) Increase in expiratory time and decrease in respiratory rate result in no air trapping and absence of dynamic hyperinflation
Additionally, during severe exacerbations, the normally passive process of exhalation becomes active in an attempt by the patient to force the inspired gas out of the lungs. High airway resistance and hyperinflation also increases the inspiratory work during inhalation. This sustained functioning of the respiratory muscles leads to increased metabolic demand and can lead to respiratory muscle fatigue over time (Oddo et al. 2006; Stather and Stewart 2005; Rotta and Steinhorn 2007). Respiratory failure can occur if ventilatory demand exceeds the work output of the respiratory muscles.
51.2.2 Assessment of Children with Acute Asthma
Accurately assessing a child’s degree of respiratory impairment is often difficult during acute exacerbations (Werner 2001; Phipps and Garrard 2003; Papiris et al. 2002; van der Windt et al. 1994). Due to their age and developmental level, acutely ill children are frequently unable to reliably and reproducibly perform tests that are used to assess severity of illness in adult patients with asthma (van der Windt et al. 1994). Spirometry and peak flow testing are generally not useful in most critically ill children (van der Windt et al. 1994; National Heart, Lung and Blood Institute. Asthma Education and Prevention Program 2007). In this population, a combination of subjective and objective parameters is best used to qualify the degree of distress (National Heart, Lung and Blood Institute. Asthma Education and Prevention Program 2007). Symptoms such as breathlessness, level of alertness, and ability to speak and physical signs such as respiratory rate, use of accessory muscles, degree of wheezing, and heart rate can provide important indicators as to the severity of a child’s exacerbation. Locally, many institutions use a clinical asthma score to quantify these objective and subjective findings (Keogh et al. 2001). These scores are used to facilitate communication between providers and to guide therapeutic interventions.
Blood gas measurements are also frequently used to assess respiratory function in this population; however, blood gas analysis has been shown not to correlate with acute asthma severity or to predict respiratory failure (Gentile et al. 2003). If the child is responding to therapy, hypercarbia alone is not an indication for intubation. Clinical judgment is crucial, since modest degrees of hypercarbia are generally well tolerated in non-intubated children with acute asthma and many children presenting with hypercarbia do not require intubation (Roberts et al. 2002). Conversely, however, a normal or rising carbon dioxide level can indicate impending respiratory failure in a child with significantly increased work of breathing and airflow obstruction.
Assessment of oxygen saturation should be performed on all children with an acute exacerbation. Compared to adults with acute asthma, children are significantly more likely to require oxygen therapy during an exacerbation. In addition, hypoxemia is an important indicator of impending respiratory failure in children with asthma (Werner 2001).
Determining which child will benefit from intubation can be challenging. The clinician must weigh the probability of improvement with therapy, the potential for respiratory arrest, and the potential complications of intubation and mechanical ventilation. However, there are some absolute indications for the intubation of a child with acute asthma. These include respiratory or cardiac arrest, severe hypoxia, or a rapid deterioration of mental status (Schramm and Carroll 2009; Biarent 2001; Werner 2001). Progressive exhaustion despite aggressive treatment is another indication for intubation; however, this finding is subjective and can be difficult to judge in young children. Other potential indications for intubation can be found in Table 51.1.
Table 51.1
Indications for endotracheal intubation
Inability to speak or absent breath sounds |
Poor aeration with absent wheezing |
Paradoxical thoracoabdominal movement |
Rising PaCO2 |
Hypoxemia (PaO2 < 60 mmHg) with deteriorating clinical status |
Altered level of consciousness (agitation, confusion, or drowsiness) |
Hemodynamic instability (hypotension or bradycardia) |
Respiratory or cardiac arrest |
In adults with asthma, certain populations have been identified that may be more likely to develop respiratory failure. In the meta-analysis by Alvarez of studies in asthmatic adults (Alvarez et al. 2005), a previous history of hospital admission and ICU admission or previous intubation for asthma was associated with an increased likelihood of mechanical ventilation. In children, the risks are less clear. A “brittle” or acute asphyxial asthma phenotype has been proposed, in which there is a brief duration of symptoms and a rapid progression to respiratory failure (Maffei et al. 2004). Response to therapy tends to be rapid and duration of mechanical ventilation short. However, others have questioned the existence of this phenotype. There are significant regional differences in the incidence of intubation and mechanical ventilation for acute asthma, ranging from 9 to 38 % in some reviews (Bratton et al. 2005) These variations suggest that provider preference and local practice have significant influence on the decision to intubate. In addition, other non-patient-related factors, such as distance from a children’s hospital, may impact whether a child is intubated.
51.3 Noninvasive Respiratory Support
Noninvasive positive pressure ventilation (NPPV) is a promising therapy for the treatment of a variety of respiratory diseases in children, including asthma (Thill et al. 2004; Carroll and Schramm 2006a; Beers et al. 2007; Soroksky et al. 2003). NPPV provides positive airway pressure via a nasal mask or a nasal-oral face mask. The positive pressure is delivered either continuously or in a bi-level mode that varies between higher inspiratory and lower end-expiratory pressures (Meduri et al. 1996; Ram et al. 2005). During acute asthma exacerbations, NPPV works by helping to avoid airway collapse during exhalation and by reducing the change in alveolar pressure needed to initiate inspiration, thereby unloading fatigued respiratory muscles and improving dyspnea. In addition, in bi-level positive airway pressure modes, the inspiratory pressure helps to improve tidal volumes, further supporting a child’s respiratory function and improving gas exchange. NPPV also preserves a child’s natural airway and airway clearance, potentially avoiding some of the complications associated with invasive ventilation and with the sedation needed to provide invasive ventilation.
Although NPPV is used with increasing frequency for the treatment of acute asthma, its utility remains controversial. Recent reviews by the Cochrane Collaboration have concluded that NPPV has not been shown to improve outcomes in adults with asthma (Ram et al. 2005). However, at least in children, early intervention with NPPV in acute severe asthma may improve outcomes and may potentially prevent endotracheal intubation (Thill et al. 2004; Carroll and Schramm 2006a; Beers et al. 2007). Several small pediatric case series have been performed, and although the sample size in each of these studies is small, each found an improvement in gas exchange and respiratory effort in children with acute severe asthma. In addition, the use of NPPV was generally well tolerated in these populations, with children requiring little or no sedation and having few adverse events. However, careful patient selection, staff familiarity with NPPV, and close monitoring of the patient were reported as important for the success of this intervention.
When using NPPV in children, providers need to be concerned about several patient-related factors. These include the challenges of finding an appropriate-sized mask as well as the prevention of gastric distention and skin breakdown. Due to the wide variation in the sizes of children, standard-sized nasal and nasal-oral masks may not fit a particular child. Nasal masks are usually best tolerated but are more prone to air leaks and therefore may not be able to deliver positive pressure reliably in uncooperative children. The use of nasal-oral masks may improve gas exchange but increase the risk of gastric distention, a common effect of NPPV. In children, gastric distention is of particular concern since an immature gastroesophageal sphincter will increase a child’s risk of vomiting and aspiration. Skin abrasions and ulcerations can occur from the pressure required to secure the mask to the child’s face. Close assessment of the patient’s skin for breakdown is important, particularly with long-term use of NPPV. There is anecdotal evidence that skin breakdown may be diminished or prevented by the prophylactic use of protective skin coverings. Whenever possible, short breaks (10–15 min every 4 h) should be taken off NPPV in order to provide relief to the skin, to assess for skin breakdown, and to reposition the mask.
51.4 Invasive Mechanical Ventilation
51.4.1 Strategies for Intubation
Rapid sequence intubation technique is the preferred method of intubation in children with acute asthma. Prior to intubation, the child should be preoxygenated with 100 % oxygen, placed on respiratory and cardiac monitors, suctioned if necessary, and the stomach decompressed. Ketamine is a preferred induction agent due to its bronchodilatory effects. Atropine may also be used as an antisialogogue and to reduce the incidence of vagal-induced bradycardia. Short-acting neuromuscular blocking agents should be considered to reduce some of the large swings in airway pressure following intubation and possibly prevent peri-intubation barotrauma and its resultant complications. The Sellick maneuver (i.e., the application of cricoid pressure during intubation) should be used to reduce the risk of aspiration. Primary (via visualization and auscultation) and secondary (end-tidal CO2 monitoring and chest radiography) methods of confirming endotracheal tube placement are essential.
51.4.2 Strategies for Mechanical Ventilation
The primary goals of mechanical ventilation during acute asthma exacerbations are to achieve adequate oxygenation and ventilation while minimizing iatrogenic hyperinflation and levels of intrathoracic pressure that could negatively impact cardiac output (Bohn and KIssoon 2001). Several strategies have been proposed to achieve these goals. However, most are based on expert reviews or small retrospective cohorts (Koh 2001; Darioli and Perret 1984; Sarnaik et al. 2004; Malmstrom et al. 2001; Shugg et al. 1990). There are no large-scale studies comparing mechanical ventilation strategies in this population. In the end, like most of pediatric critical care, in the absence of evidence-based medicine, the treatment of acute asthma and respiratory failure involves primarily phronesis (practical wisdom): a customized decision for an individual patient (Tobin 2008).
However, there are several key themes in the ventilation of children with acute asthma and respiratory failure that are generally accepted. Controlled hypoventilation with low peak inspiratory pressures, low respiratory rates, and permissive hypercapnia is often used with the perception that this strategy improves outcomes and decreases complications (Darioli and Perret 1984; Sarnaik et al. 2004). Plateau pressures of less than 30 cm H2O and tidal volumes of less than 8 mL/kg are suggested to reduce the potential for barotrauma (Levy et al. 1998). These lower pressures and volumes also may reduce iatrogenic increases in intrathoracic pressure that may significantly impair preload and cardiac output. A strategy of lower respiratory rates may allow adequate expiratory time to accommodate for delayed time constants and also reduce iatrogenic dynamic hyperinflation. However, in acute asthma, a child’s lung has heterogeneous areas with varying degrees of obstruction. As a result, during mechanical ventilation a child may not be able to reach a complete expiratory volume. However, a clinician may be able to provide sufficient emptying to prevent most dynamic hyperinflation while balancing the need to provide some degree of ventilation. A strategy of permissive hypercapnia with buffering of pH (goal >7.2) is used in these cases. When possible, spontaneous respiration should also be used to allow a child to regulate their own respiratory rate and expiratory time. However, practically in children, difficulty with providing sedation may preclude support modes without set respiratory rates.
Both pressure-limited and volume-limited modes have been used to ventilate children with acute asthma and respiratory failure, but neither approach has been demonstrated to be more effective than the other (Sarnaik et al. 2004; Malmstrom et al. 2001). However, there are important advantages and disadvantages of each therapy to consider when the clinician weighs the decision about which mode to use in a particular patient. Pressure-limited modes provide decelerating gas flow with a preset peak inspiratory pressure and an inspiratory tidal volume that will vary based on changes in compliance and resistance. This has the theoretical advantage of limiting hyperinflation in this population; however, high levels of airway resistance may limit effective tidal volumes, and sudden changes in resistance with acute bronchospasm can cause significant variation in delivered tidal volume. Volume-limited modes will provide a more constant tidal volume and may provide for more consistent delivery of aerosolized medications. However, due to the heterogeneous nature of the lung in acute asthma, there may be overdistention and air trapping in areas with less airway obstruction. Volume-limited modes also have the advantage of allowing the clinician to assess for changes in resistance and dynamic and static compliance by assessing changes in peak and plateau pressures over time. Response to therapy can therefore be quantified by examining for any decrease in the difference between peak and plateau pressures that will reflect improving resistance. Newer, pressure-regulated volume control modes can provide a guaranteed tidal volume with a decelerating gas flow and combine some of the advantages of both pressure-limited (preset peak pressure) and volume-limited ventilations (consistent minute ventilation). These modes may be advantageous in children with acute asthma, but clinical trials are needed.
The use of external PEEP in patients with acute asthma and respiratory failure has been controversial. External PEEP may further increase air trapping and dynamic hyperinflation, factors that have been associated with increased complications of mechanical ventilation (Tuxen and Lane 1987). For these reasons, some authors continue to advocate no external PEEP (Oddo et al. 2006). Other authors, however, advocate an extrinsic PEEP to match intrinsic auto-PEEP, theorizing that some external PEEP minimizes atelectasis and helps to maintain patent airways to facilitate mucous clearance in this population. In practice, the potential beneficial effects of external PEEP must be balanced with the potential negative effects of external PEEP in a particular patient.
51.5 Use of Inhalational Gases
51.5.1 Heliox
Heliox, a blend of helium and oxygen, reduces airway resistance by converting density-dependent turbulent airflow within the airways to a more laminar flow (Barach 1935). As such, heliox is an attractive therapy for children with severe asthma, potentially reducing work of breathing, increasing nebulized drug delivery, and improving gas exchange to the distal airways. Small studies in children have found a reduction in dyspnea, improved gas exchange, and improved pulmonary function in some patients. However, these beneficial effects are most apparent within the first hour of therapy, after which most conventionally treated children improve similarly. In addition, a recent a systematic review of the literature failed to demonstrate a significant beneficial effect for the treatment of acute asthma in children (Ho et al. 2003). However, helium is a biologically inert gas, and heliox has no known adverse effects. With this risk/benefit profile, a brief trial of heliox seems warranted in select children with severe asthma. If the child does not rapidly improve with this therapy, the heliox should be discontinued and other therapies considered. Clinicians need to be aware, however, that to significantly lower the density of the inhaled gas, helium needs to comprise 60–80 % of the mixture. Significantly hypoxemic children who require greater than 40 % inspired oxygen should therefore not be considered for this therapy.
51.5.2 Inhalational Anesthetics
Volatile inhalational anesthetics are potent bronchodilators and have been used to treat severe refractory exacerbations in both adults and children (Maltais et al. 1994; Shankar et al. 2006). Although their mechanism of action has not been established, some of the proposed mechanisms include direct relaxation of bronchial smooth muscles, β2-adrenergic receptor stimulation, and inhibition of hypercapnic bronchoconstriction (Maltais et al. 1994; Shankar et al. 2006). Because of the novelty of these proposed therapeutic mechanisms, inhalational anesthetics may be particularly useful for the treatment of acute asthma that is refractory to conventional bronchodilators. Halothane, isoflurane, and sevoflurane have each been reported to be beneficial (Maltais et al. 1994; Shankar et al. 2006). As with heliox therapy, the beneficial effects of these inhalation anesthetics typically occur rapidly. But unlike heliox, the effects may be sustained (Shankar et al. 2006).
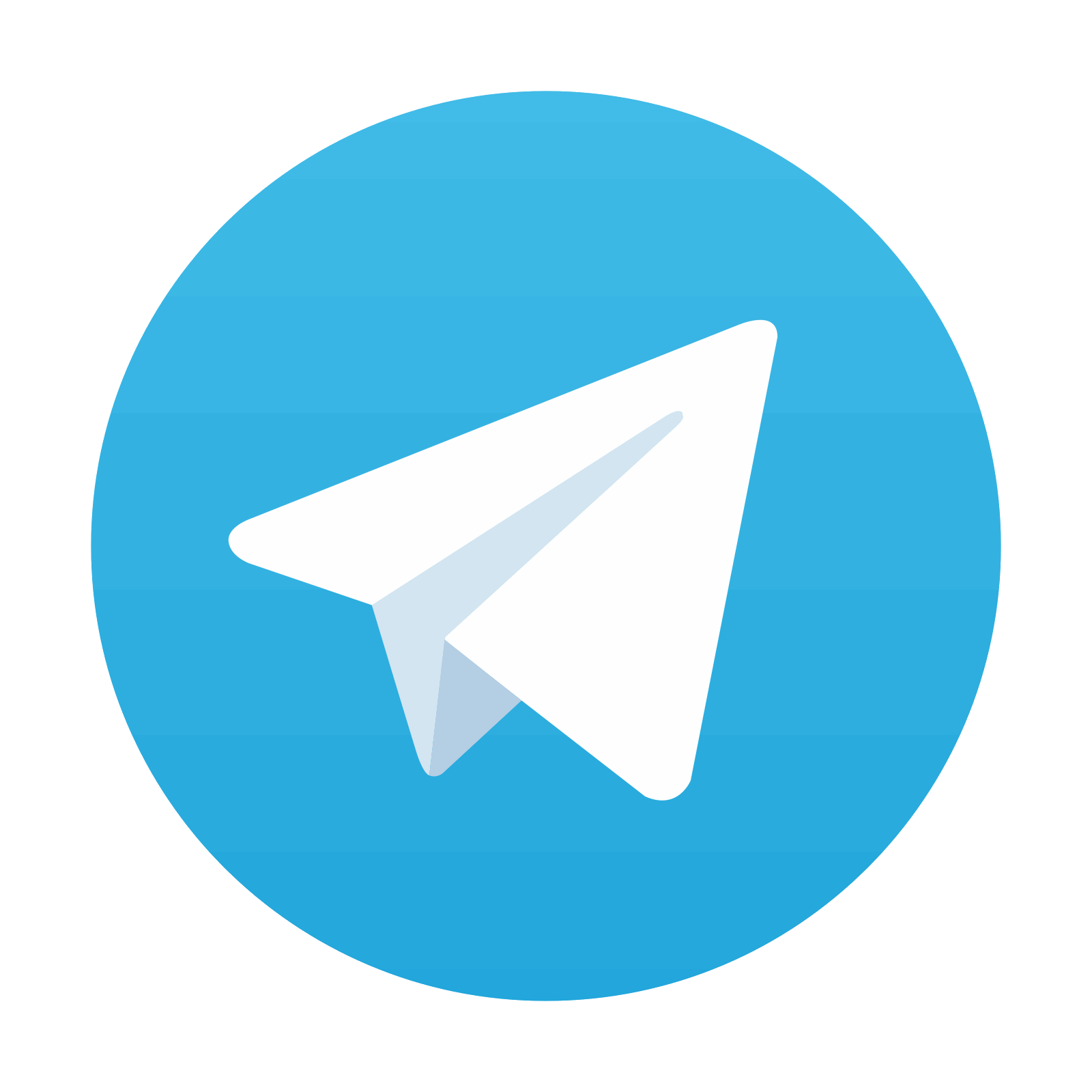
Stay updated, free articles. Join our Telegram channel

Full access? Get Clinical Tree
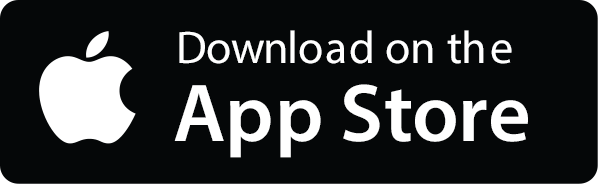
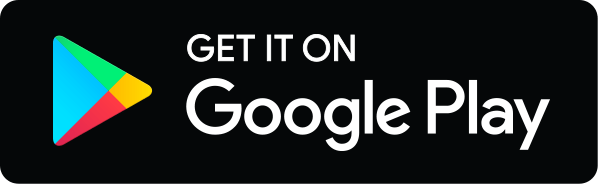