Acute respiratory distress syndrome (ARDS) represents the final common pathway and clinical presentation of the most severe acute lung injury (ALI), which may be precipitated by various pulmonary (direct) or nonpulmonary (indirect) insults. Infections are an important cause of ARDS in children, with pneumonia and sepsis being most common. ARDS is an important consideration in the setting of pediatric infectious diseases, given the wide range of infections that may lead to this complication and its associated morbidity and mortality. Morbidity, mortality, and resource usage related to ARDS also increase during outbreaks of infectious diseases, as seen during the 2009–10 H1N1 influenza pandemic. This chapter will discuss the definition, pathophysiology, presentation, and clinical management of children with ARDS, with an emphasis on aspects that may be of particular interest to pediatric infectious diseases specialists.
Definition
Although first described by Ashbaugh and colleagues in 1967, a formal definition of ARDS was not developed until 1994 when the American-European Consensus Conference (AECC) defined ARDS as follows :
- 1.
Acute onset of respiratory symptoms
- 2.
Frontal chest radiograph with bilateral infiltrates
- 3.
Partial pressure of oxygen (Pa o 2 ) to fraction of inspired oxygen (Fi o 2 ) ratio (P/F ratio) 200 mm Hg or less
- 4.
No clinical evidence of left atrial hypertension as defined by a pulmonary capillary wedge pressure less than 18 mm Hg (if measured)
A subsequent definition that addressed these limitations is commonly referred to as the Berlin Definition of ARDS and was developed in 2011 by the European Society of Intensive Care Medicine in collaboration with the American Thoracic Society. The term ALI was removed in favor of considering ARDS to be a single entity with three subgroups based on the severity of hypoxemia (i.e., mild, moderate, and severe). The Berlin Definition of ARDS takes into account the timing of onset, the degree of respiratory support (positive airway pressure), and the overall fluid status. This new definition also allowed for the use of either computed tomography (CT) of the chest or standard chest radiograph as part of the diagnostic criteria for ARDS.
The Berlin definition, however, failed to consider differences between adults and children in the presentation and management of ARDS. In 2015, after a 2-year process, the Pediatric Acute Lung Injury Consensus Conference (PALICC) published pediatric-specific definitions for ARDS and recommendations regarding management and suggested priorities for future research. PALICC defined pediatric ARDS (PARDS) with the use of the oxygenation index ([Fi o 2 × mean airway pressure × 100]/Pa o 2 ) or the oxygen saturation index ([Fi o 2 × mean airway pressure × 100]/Sp o 2 ) as a means of assessing severity ( Table 65.1 ). Some of the key PALICC recommendations were (1) no age criteria for the definition of PARDS in order that the pathophysiology of PARDS be better studied across the spectrum of age groups in future studies; (2) exclusion of perinatal-related lung injury; (3) inclusion of infants and children requiring noninvasive ventilation, as well as (4) inclusion of those with congenital heart disease and chronic lung disease; and (5) inclusion of patients with unilateral lung disease.
Age | Exclude patients with perinatal related lung disease |
Timing | Within 7 days of known clinical insult |
Origin of Edema | Respiratory failure not fully explained by cardiac failure or fluid overload |
Chest Imaging a | Chest imaging findings of new infiltrate(s) consistent with acute pulmonary parenchymal disease |
Oxygenation | Noninvasive Mechanical Ventilation | Invasive Mechanical Ventilation | ||
Mild | Moderate | Severe | ||
4 ≤OI<8 | 8 ≤OI<16 | OI ≥16 | ||
Full face-mask bi-level ventilation or CPAP ≥5 cm H 2 O, PF ratio ≤300, SF ratio ≤264 | 5 ≤OSI<7.5 | 7.5 ≤OSI<12.3 | OSI ≥12.3 |
SPECIAL POPULATIONS | |
---|---|
Cyanotic Heart Disease | Standard preceding criteria for age, timing, origin of edema, and chest imaging with an acute deterioration in oxygenation not explained by underlying heart disease |
Chronic Lung Disease | Standard preceding criteria for age, timing, and origin of edema with chest imaging consistent with new infiltrate and acute deterioration in oxygenation from baseline that meet preceding oxygenation criteria |
Left Ventricular Dysfunction | Standard preceding criteria for age, timing, and origin of edema with chest imaging consistent with new infiltrate and acute deterioration in oxygenation that meet preceding criteria not explained by left ventricular dysfunction |
Pathology and Pathophysiology
The clinical stages of ARDS coincide with three pathologic stages: exudative stage, proliferative stage, and fibrotic stage. The exudative stage is characterized by accumulation of protein-rich edema fluid in the alveoli following direct or indirect injury to the alveolar-capillary membrane (see Table 65.1 ). Neutrophils play a predominant role in this stage of ARDS. Pulmonary compliance is worsened by the presence of edema, widespread atelectasis, and the inactivation of surfactant that results from the presence of fibrin and other plasma proteins and inflammatory mediators in the alveolar space. Microthrombi develop within the pulmonary vasculature and numerous vasoactive mediators are released from inflammatory cells and the activated endothelium contributing to the development of elevated pulmonary vascular resistance and the ventilation/perfusion abnormalities characteristic of ARDS. The degree of epithelial injury and the subsequent ability to clear edema fluid, along with the reversibility of pulmonary hypertension, are important predictors of outcome in ARDS.
The proliferative stage occurs 1 to 3 weeks after the initiation of injury and is characterized by attempted repair of the disrupted alveolar-capillary membrane. The mechanism of this repair requires not only the close coordination of numerous growth factors but also an intact basement membrane to provide a platform for cell adhesion and migration. The ability of the alveolar epithelium to remove edema fluid depends on the degree of inflammation and injury in the exudative stage. Antiinflammatory cytokines such as interleukin-10 (IL-10) and lipid mediators are particularly important mechanisms aimed at limiting the degree of injury. If lung injury and inflammation persist, the patient may develop severe physiologic abnormalities and may progress to the fibrotic stage of ARDS.
The fibrotic stage may be seen as early as 5 to 7 days after the onset of disease, although this degree of injury may not be clear for several weeks. Histologically the alveolar space becomes filled with mesenchymal cells, and lung tissue is replaced by collagenous tissue. In addition, vascular changes in the fibrotic stage can lead to increased thickness of the pulmonary vasculature and even obliteration of small capillaries. Overall these changes markedly decrease the available surface area for gas exchange and result in decreased exercise tolerance in survivors of ARDS. In some patients, intractable respiratory failure or chronic lung disease result, necessitating prolonged ventilator support.
Etiology
The inflammatory cascade that ultimately results in ARDS can be triggered by a variety of insults that directly or indirectly affect the lung ( Box 65.1 ). Direct pulmonary insults include pneumonia, aspiration, chest trauma, drowning injury, and smoke inhalation. Indirect lung injury may be the result of generalized systemic conditions, such as sepsis, closed head injury, multisystem trauma, transfusion reactions, pancreatitis, and hemorrhagic shock. A study of 146 PARDS cases found that infectious etiologies were the most common causes of ARDS: 35% had bacterial pneumonia, 20% had lung disease due to respiratory syncytial virus (RSV), and 27% had sepsis. ARDS was due to trauma in only four (3%) pediatric cases.
Direct (Pulmonary) Causes
Pneumonia
- •
Bacterial
- •
Viral: influenza, respiratory syncytial virus
- •
Mycobacterial
- •
Fungal
- •
Aspiration
Drowning
Smoke or chemical inhalation
Pulmonary contusion
Indirect (Nonpulmonary) Causes
Severe sepsis and septic shock
Significant trauma
Severe hemorrhage
Severe pancreatitis
Transfusion reaction
Cardiopulmonary bypass
Thermal burn
Bacterial lower respiratory tract infection is the most common inciting event in PARDS, although severe sepsis is often also present. Streptococcus pneumoniae and Pseudomonas aeruginosa are bacteria commonly associated with severe pneumonia potentially leading to sepsis and, subsequently, ARDS. Some bacteria may be associated with a higher risk of ARDS than other pathogens because of the production of cytotoxic and chemotactic toxin production.
Viral pneumonia is also an important initiator of ARDS and is frequently not diagnosed due to testing limitations. RSV infection is one of the most common indications for admission to the pediatric intensive care unit (PICU), especially during the winter months. Although most critically ill children present as severe RSV bronchiolitis, a significant proportion (up to 27%) can present with ARDS; those with preexisting conditions are at the highest risk. Influenza virus is another important cause of ARDS. In one study, 80% of children admitted to the PICU with influenza developed ARDS. A single-center study found that as many as 24% of patients with the 2009 pandemic H1N1 strain developed ARDS. A less common cause of viral-induced ARDS is coronavirus, particularly the strain that caused the severe acute respiratory syndrome (SARS) outbreak in 2003, which affected adults more severely than children. Bacterial coinfection increases the risk that viral pneumonia will cause ARDS. For example, in patients with the severe pandemic H1N1 viral infection, bacterial coinfection was shown to increase the need for mechanical ventilation, duration of intensive care unit (ICU) stay, and mortality.
In addition to lower respiratory tract infections, severe sepsis is a leading risk factor for indirect ARDS in children. ARDS has been described following sepsis due to many different organisms and does not seem to be directly related to pathogen-specific factors. Multiorgan dysfunction and other illnesses associated with sepsis increase the risk for ARDS, and this risk is further increased by the presence of underlying comorbidities. In critically ill adults, sepsis is associated with an approximately 40% risk of developing ARDS. Sepsis appears to be a less common cause of ARDS in pediatric patients; one study found that 15% of PARDS cases were attributable to sepsis.
Limited data exist on the impact of the underlying etiology of ARDS on clinical outcomes in children. In a study of 736 adults with ARDS, patients with sepsis-related ARDS were more likely to have diabetes, longer pre-ICU stay, and higher APACHE III (a measure of severity of illness in critically ill adults) scores in contrast to those who had non–sepsis-related ARDS. However, after accounting for differences in clinical characteristics, no difference was found in 60-day mortality between patients with sepsis-related ARDS and non–sepsis-related ARDS. Furthermore no evidence exists to suggest that the source of sepsis is an important determinant of mortality in ARDS. However, genetic differences in the immune regulation underlying responses of the lungs to these precipitating factors do account for some of the differences in the degree of severity in ARDS in individual patients, even when similar risk profiles are present. One such example of genetic polymorphisms is that related to nuclear factor-κB (NF-κB). A deletion polymorphism of the promoter region of this important transcription factor leads to inappropriate upregulation of proinflammatory genes, which has been shown to result in an increase in ARDS severity and mortality.
Clinical Manifestations
The initial clinical course of ARDS begins with direct or indirect acute injury to the pulmonary parenchyma. In the initial stage, the clinical symptoms and physical findings vary depending on the underlying etiology of the ALI. Children with pulmonary etiologies of ARDS will have predominantly respiratory symptoms; those with nonpulmonary etiologies of ARDS will have symptoms related to the initial insult (e.g., acute abdomen for pancreatitis). Early in the course of lung injury, patients may display mild tachypnea and dyspnea but tend to have normal radiographic findings.
After the inciting event and initial phase, a latent period of variable duration follows. During this latent period, the patient may appear to be clinically stable, but early signs of pulmonary insufficiency develop, as manifested by hyperventilation with hypocarbia and respiratory alkalosis. The chest radiograph may begin to demonstrate a fine reticular pattern related to increases in pulmonary interstitial fluid ( Fig. 65.1 ). As the degree of lung injury worsens, acute respiratory failure follows and is characterized by rapid onset of hypoxemia that is often refractory to supplemental oxygen administration. Diffuse pulmonary edema and worsening compliance cause significant atelectasis and intrapulmonary shunting. Clinically patients develop rapid, shallow tachypnea with increased work of breathing. Subcostal and supraclavicular retractions, grunting, and nasal flaring are common. Lung examination usually reveals diffuse crackles. Radiographically bilateral areas of consolidation with air bronchograms reflect alveolar filling and atelectasis ( Fig. 65.2 ). CT may provide additional information. A significant percentage of these children will require endotracheal intubation and mechanical ventilation. However, noninvasive ventilation may be an alternative for a subgroup of patients.


Mortality
Most studies indicate that mortality associated with ARDS is due to nonrespiratory causes. In most cases, early death (i.e., within 72 hours) is caused by the underlying illness or injury, whereas late death (i.e., beyond 72 hours) is caused by infection or multiorgan system failure. Reported mortality rates for children with ARDS vary greatly and range from 8% to 79%. This variation is likely due to the myriad of underlying causes of ARDS, the differing degrees of ARDS severity, and the presence or absence of concomitant organ failures. ARDS secondary to sepsis appears to confer the highest risk of death, likely due to the systemic dysfunction present at the onset of ARDS symptoms. Some of the factors that have been identified as predictors of mortality in PARDS include P/F ratio, oxygenation index, pH, Pediatric Risk of Mortality (PRISM) score (a severity index score commonly used in PICUs), and presence of multiorgan failure. Despite the large reported variation in mortality, it is generally accepted that the overall mortality rate of PARDS is lower than that of the adult population. Additionally ARDS-related mortality has decreased over the past several years, likely due to improvements in supportive care and the widespread adoption of low tidal volume ventilation strategies, the only mechanical ventilation strategy that has ever been shown to decrease mortality in ARDS in a randomized clinical study. This study was conducted in adults and, although the role of low tidal volume ventilation in reducing mortality in pediatric patients in not clear, mortality rates following PARDS have fallen from 80% in the 1980s to approximately 20% in the early 2000s and are potentially under 15% now.
Treatment
Goals of treatment of patients with ARDS include treating the underlying disease (when possible), achieving adequate tissue oxygenation, and minimizing ventilator-induced lung injury (VILI). In patients in whom ARDS is secondary to sepsis or bacterial lower respiratory tract infection, prompt administration of appropriate antibiotics is essential. The antimicrobial approach depends on patient-specific risk factors as well as patterns of drug resistance in the community and within a given hospital. Local rates of cephalosporin-resistant S. pneumoniae and methicillin-resistant Staphylococcus aureus (MRSA) may necessitate broad-spectrum gram-positive coverage (e.g., vancomycin). Patients with neutropenia or other risk factors for sepsis due to gram-negative pathogens may require extended-spectrum penicillins or cephalosporins as part of their empiric therapy. The mainstay of support in the ARDS patient is the provision of supplemental oxygen and mechanical ventilation. In addition, nonpulmonary organ function must be meticulously maintained to optimize the clinical care of children with ARDS.
Pulmonary Management
Modes of Mechanical Ventilation
Multiple modes of mechanical ventilation are currently used in clinical practice to provide respiratory support for patients with ARDS. Regardless of the conventional mechanical ventilation (CMV) mode used, it is paramount that VILI be minimized by paying meticulous attention to prevention of barotrauma and volutrauma. Prospective, randomized multicenter studies comparing a pressure-controlled mode to a volume-control mode in the setting of ARDS are limited and have not demonstrated a single ventilatory mode as being superior.
However, a landmark study of adults with ARDS published in 2000 did show that low tidal volume mechanical ventilation (6 mL/kg) decreased mortality by 22% and increased the number of ventilator-free days in contrast to a more traditional tidal volume (12 mL/kg). This study is the only investigation in either adult or pediatric ARDS that has demonstrated a mortality benefit associated with a specific ventilatory approach. It is important to mention that two pediatric-focused observational studies demonstrated an inverse relationship between delivered tidal volumes and mortality. However, a large retrospective multicenter pediatric study of acute hypoxic respiratory failure did not reveal an association between tidal volume and either mortality or ventilator-free days. One must be careful not to make definitive conclusions from these pediatric-based studies, which demonstrate association rather than cause and effect. Without pediatric-specific definitive data, the low tidal volume approach has generally become standard practice in the PICU environment.
In contrast to CMV, high-frequency oscillatory ventilation (HFOV) is an alternative mode that delivers extremely small-volume “breaths” at high rates (5 to 15 Hz [equivalent to 300 to 900 breaths/min]). Although use of HFOV has become commonplace in pediatrics, the only definitive study of this method in PARDS is a crossover trial conducted more than 20 years ago that showed improved oxygenation and a reduction in the need for supplemental oxygen at 30 days. The adult-based OSCAR and OSCILLATE ARDS trials of HFOV have led to a negative view of this ventilatory modality. OSCAR found no difference in mortality between HFOV and CMV. OSCILLATE was prematurely stopped due to higher mortality with HFOV compared to control ventilation. The RESTORE HFOV study compared the length of mechanical ventilation in pediatric subjects managed with early (within 24 to 48 hours of intubation) HFOV compared to patients receiving CMV or late HFOV by means of a propensity score analysis. After adjusting for risk category, early HFOV was associated with an increased length of ventilation but not increased mortality as compared to patients managed with CMV/late HFOV. However, this study raises an important question of whether the increased length of ventilation with early HFOV is directly related to HFOV or rather to the manner in which this modality was used. Unfortunately, pediatric clinicians are left to determine whether or not to use HFOV based on their interpretation of the available data as well as their clinical experience. In general, despite the lack of definitive evidence in support of HFOV in pediatrics, it has become an often-used technique in the management of PARDS.
Positive End-Expiratory Pressure
Positive end-expiratory pressure (PEEP) is the constant pressure applied to the airways and alveoli during exhalation and helps maintain alveolar patency and restore functional residual capacity. PEEP is typically titrated to a level that allows adequate oxygenation at an acceptable Fi o 2 .
The ARDS Network investigated the optimal PEEP-Fi o 2 approach for adult patients with ARDS and showed that both “lower” and “higher” PEEP strategies produced similar survival rates. The results of this study suggest that as long as adequate PEEP is applied, higher levels are not necessary but are acceptable. Once appropriate PEEP is applied to maintain the lungs at an ideal volume, further increases in PEEP in an attempt to reduce the Fi o 2 do not lead to an improved outcome. A follow-up meta-analysis of almost 2300 patients demonstrated that patients with pure ALI (i.e., recruitable lung) benefited from a higher PEEP strategy, whereas those without recruitable lung did not. Based on these data, a strategy that individualizes PEEP based on the specific clinical circumstances seems warranted. As long as alveolar collapse is minimized, the balance between PEEP and Fi o 2 can be left to the discretion of the clinician.
Permissive Hypoxemia and Permissive Hypercapnia
Every child with ARDS is hypoxemic by definition. Prolonged administration of high concentrations of oxygen can damage lungs due to the formation of oxygen free radicals. Human and animal studies suggest that a prolonged Fi o 2 of greater than 0.60 should be avoided to prevent oxygen-induced pulmonary damage. However, the exact Fi o 2 cutoff for oxygen toxicity in the ARDS patient, especially in children, remains unknown and may be less than 0.60. Increases in the set PEEP may allow for a reduction of the delivered Fi o 2 .
In the setting of ARDS, improved oxygenation has not been associated with improved outcomes. This finding was best demonstrated in the ARDS Network low tidal volume study, in which the control (12 mL/kg) group demonstrated improved oxygenation for the first 72 hours of mechanical ventilation but ultimately had a higher mortality in contrast to the lower tidal volume (6 mL/kg) group. In a pediatric study of 470 children, no correlation was found between oxygenation or ventilation measured during the first 14 days of PICU admission in survivors or nonsurvivors of pediatric ALI. Other studies in PARDS have demonstrated short-term improvements in oxygenation parameters with no difference in mortality. The ideal target for oxygenation in ARDS remains controversial, but it is clinically reasonable to accept an Sa o 2 of less than 90% as long as tissue oxygen delivery is adequate. This concept of accepting lower arterial oxygen saturations is termed permissive hypoxemia . In general, Fi o 2 , PEEP, and other ventilatory parameters should be set to optimize oxygen delivery with tolerance of lower oxygen saturations to allow for minimization of VILI. Specific recommendations for a lower level of acceptable oxygen saturations cannot be made.
Permissive hypercapnia is another strategy commonly used in patients with ARDS. Hypercapnia is a consequence of low tidal volume ventilation, and limited evidence suggests that low-volume, pressure-limited ventilation with permissive hypercapnia may improve outcomes in patients with ARDS. In a 10-year study, Milberg and colleagues reported an association of permissive hypercapnia with decreased mortality. Recent data from a laboratory model of ischemia-reperfusion ALI indicate that hypercapnic acidosis is protective and that buffering of the hypercapnic acidosis actually attenuates these protective effects. Animal models have also suggested that permissive hypercapnia may attenuate lung damage in sepsis-induced ARDS. Although permissive hypercapnia may inhibit the immune response to some degree, this results in decreased inflammation with an associated decrease in ALI. Inhibition of the immune system is of particular concern in patients with sepsis, but administration of antibiotics appears to prevent these deleterious effects. These findings, along with data demonstrating worsening mortality with each hour in which antibiotics are delayed in septic patients, reinforce the need for early administration of antibiotics in the management of pediatric sepsis and ARDS. Despite these potential benefits and evidence suggesting that respiratory acidosis is not harmful, the exact degree of hypercapnia that can be safely tolerated remains controversial. Most of the undesirable effects of hypercapnia are reversible and minor when pH is greater than approximately 7.20. Further clinical studies looking at the role of permissive hypercapnia in sepsis-related lung injury are needed given the increasing preclinical evidence of its potential therapeutic role.
Adjunctive Therapies
Corticosteroids
Given that inflammation is the cornerstone of the pathophysiology of ARDS, corticosteroids have therapeutic potential as immunomodulatory or antiinflammatory agents. An inherent difficulty in summarizing the use of corticosteroids is the significant variability in the timing and dose of steroids used in the various studies. The timing of steroids in published clinical studies of adults ranges from 72 hours to 4 weeks from the onset of ARDS, and the doses of methylprednisolone (or its equivalent) range from 1 mg/kg per day to 120 mg/kg per day. Meta-analyses of corticosteroid use within the first 2 weeks in adults with established ARDS demonstrated that steroids may reduce mortality and reduce total days of mechanical ventilation. A more recent meta-analysis of patients with ARDS due to community-acquired pneumonia found no difference in mortality for patients treated with steroids or placebo but did find that steroids reduced length of hospital stay and the duration of mechanical ventilation. Although few studies evaluating steroids have included children, a recent prospective observational study of 283 PARDS patients found that steroids were associated with a longer duration of mechanical ventilation with no difference in mortality. Additionally, based on current data, corticosteroids do not appear to have a role in preventing ARDS. The use of steroids has not been associated with an increase in secondary infections.
Inhaled Nitric Oxide
Pulmonary vascular dysfunction is an important component of the pathophysiologic process or ARDS. The impact of ARDS on pulmonary vascular tone has led to an extensive investigation of inhaled nitric oxide, a potent vasodilator that works exclusively in the pulmonary vasculature, as a potential therapeutic option. Studies of inhaled nitric oxide for PARDS have demonstrated temporary improvement in oxygenation acutely but not improved survival. The largest pediatric inhaled nitric oxide study to date involved 108 children. Because of the crossover design, the study was not able to describe mortality results, but it did demonstrate that the groups of children who may acutely benefit most are immunocompromised patients and those with greater severity of hypoxemia.
A recent Cochrane Database Systematic Review looking at 14 randomized controlled trials concluded that inhaled nitric oxide cannot be recommended for either children or adults with ARDS because it resulted in transient improvement in oxygenation without mortality benefit. Furthermore it appeared that inhaled nitric oxide may increase the risk for renal impairment among adults with ARDS. Except for the potential treatment of documented pulmonary hypertension and/or severe right ventricular dysfunction, the administration of inhaled nitric oxide for ARDS cannot be recommended based on the available data.
Surfactant Replacement
In ARDS, injury occurs to type 2 pneumocytes, resulting in decreased production of surfactant. This injury has prompted studies examining the possibility of the effectiveness of surfactant replacement in the management of ARDS. A study of 153 pediatric patients reported that exogenous surfactant acutely improved oxygenation and significantly decreased mortality in infants and children with ALI. There were no significant decreases in duration of mechanical ventilation, length of PICU admission, or length of hospital stay. The results of this study are controversial because a significantly higher number of immunocompromised patients (i.e., with a higher expected mortality) were included in the control group. Randomized trials in adults found that, for 450 adults with ARDS randomized to surfactant or placebo, there was no difference in mortality and, for 308 adults randomized to calfactant or placebo, there was no improvement in oxygenation or survival. More recent pediatric studies that included a total of 274 patients have also shown that surfactant does not improve mortality in infants and children. Surfactant replacement is not currently recommended for the treatment of PARDS.
Nonpulmonary Supportive Management
Fluid Balance
Initial aggressive fluid resuscitation is important in maintaining hemodynamics and improving clinical outcomes in patients with shock. However, once hemodynamics are restored, overhydration may lead to pulmonary edema. Fluids should be carefully titrated and fluid status monitored while normalizing the patient’s intravascular volume status and maintaining adequate cardiac output. The ARDS Network conducted a study to investigate conservative and liberal fluid management strategies in adult patients with ARDS. In this study, a difference of 6000 mL was seen in the 7-day cumulative fluid balance between the two groups, with patients in the conservative fluid management group having improved lung function and shorter duration of mechanical ventilation and ICU length of stay. In critically ill children, the role of fluid balance is less conclusive. Although some studies demonstrate that fluid balance has an impact on oxygenation and morbidity, other prospective pediatric studies have shown otherwise. The Pediatric Acute Lung Injury and Sepsis Investigators (PALISI) Network investigators showed that cumulative fluid input and output did not affect the speed of weaning from mechanical ventilation or extubation outcomes.
Sedation and Neuromuscular Blockade
Appropriate sedation and analgesia are important in children who are on mechanical ventilation. Prolonged sedation and neuromuscular blockage have been associated with worse clinical outcomes, and the long-term effects of sedative medications are unknown. However, a recent study demonstrated that early neuromuscular blockage improved survival in adults with ARDS. In this study of 340 adults with ARDS, the use of cisatracurium for 48 hours improved 90-day survival by nearly 1.5 times compared to placebo without an increase in muscle weakness. In contrast, growing evidence in adults indicates substantial benefits associated with sedation weaning and promotion of early mobilization. In children, a sedation weaning protocol was not found to change the duration of mechanical ventilation, PICU or hospital lengths of stay, or in-hospital mortality. Given the lack of consistent evidence in both adults and children, the use of neuromuscular blockade in children with ARDS remains controversial. Overall use of sedatives and neuromuscular blocking agents should be driven by a balance between maintaining adequate gas exchange and the potential adverse effects of critical illness weakness and myopathy.
Nutrition
As a general approach, adequate nutrition should be provided to optimize caloric intake and avoid a negative nitrogen balance. However, few studies examining the effects of nutrition in children with ARDS have been conducted. Clinical guidelines with regard to nutritional support of critically ill children are, at best, supported by small, randomized trials with reasonable risk for bias in their results. Although a growing number of studies are investigating the potential utility of nutritional supplementation in ARDS, the results have not been consistent or encouraging. Using bronchoalveolar and plasma biomarkers as their end points, investigators examining the effect of omega-3 oil on adults with ARDS did not note any difference in IL-8, IL-6, and leukotriene B4 levels between patients with and without supplementation. This was further supported by a recent clinical study that was stopped early for futility. This study of twice-daily supplementation of omega-3 fatty acids within 48 hours of onset of ARDS showed a trend toward longer duration of mechanical ventilation and higher mortality in the treatment group.
Patient Isolation
Even though infectious etiologies are the most common cause of ARDS in children, routine isolation of all ARDS patients is not warranted. In certain infective etiologies such as viruses (e.g., influenza, RSV, adenovirus), resistant bacteria, and mycobacteria (e.g., tuberculosis), appropriate isolation measures should be instituted.
Rescue Therapies
Some patients with severe ARDS become refractory to maximal therapies and are unable to achieve acceptable therapeutic goals while avoiding oxygen toxicity or intolerably high airway pressures. In these circumstances, extracorporeal membrane oxygenation (ECMO) has increasingly been used as a rescue modality for these patients.
ECMO involves withdrawing blood from the patient by a mechanical pump and directing it to a membrane oxygenator, where oxygenation and removal of carbon dioxide occur before the blood is returned to the patient. In refractory ARDS patients, venovenous ECMO is commonly used, which involves blood being withdrawn from the patient via a cannula placed in a central vein and returned to the venous system. Cardiac function must be sufficient to circulate this returned blood systemically to the body. ECMO has been used successfully in a wide range of clinical circumstances and is a viable treatment strategy in adults and children with refractory ARDS. Traditionally ECMO has been implemented in patients with an anticipated mortality that is extremely high, exceeding 80% to 90% in many circumstances, but reported survival from the Extracorporeal Life Support Organization (ELSO) registry for adults and children with ARDS is currently 50% and 65%, respectively. In the past decade, use of ECMO has continued to increase, as evidenced by reports from the 2009 H1N1 influenza A pandemic. A more detailed discussion of ECMO support for pediatric and adult ARDS patients is beyond the scope of this chapter.
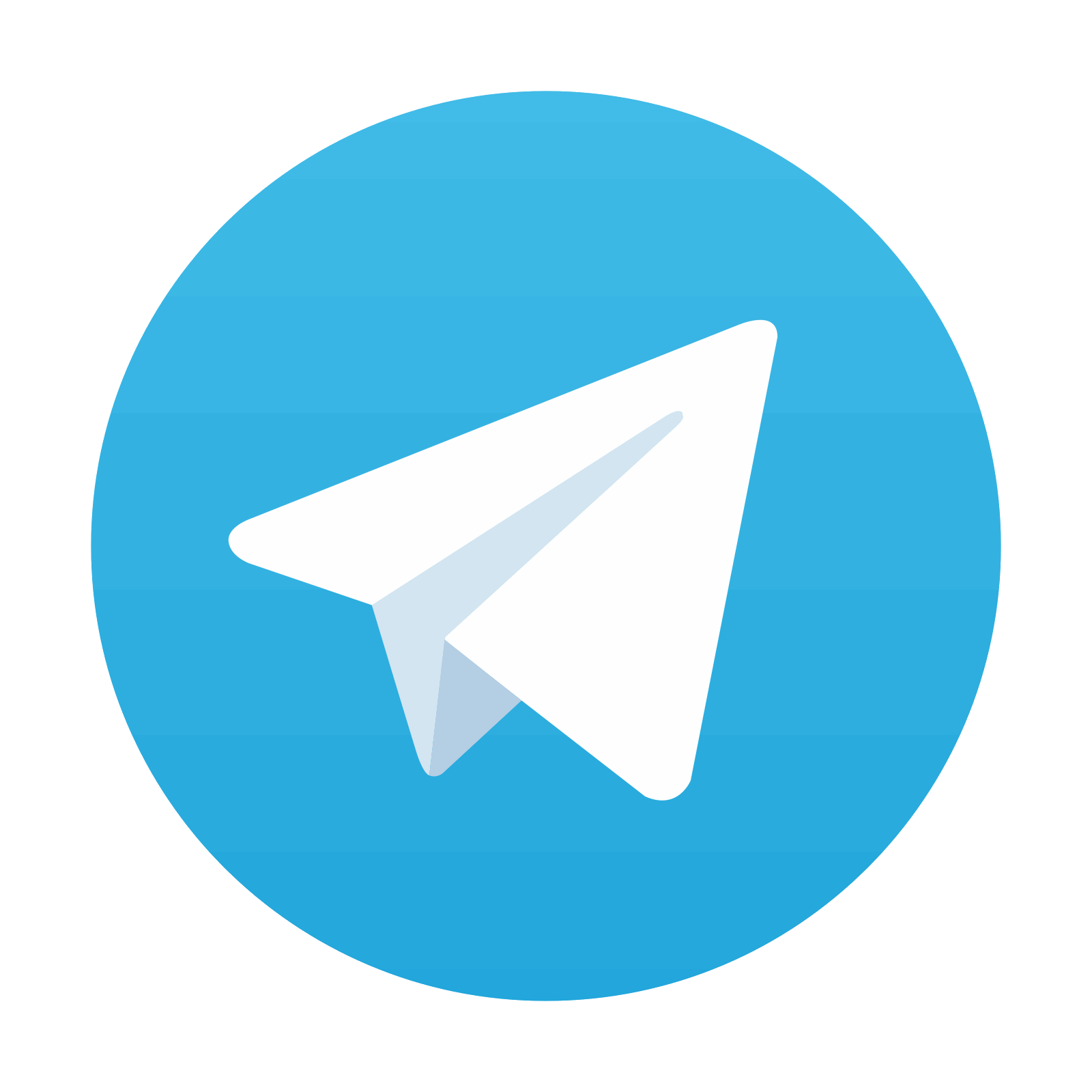
Stay updated, free articles. Join our Telegram channel

Full access? Get Clinical Tree
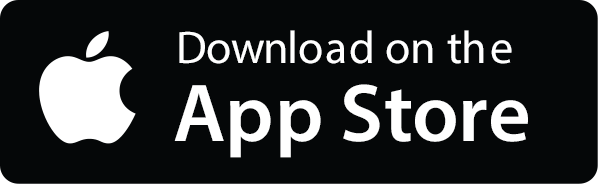
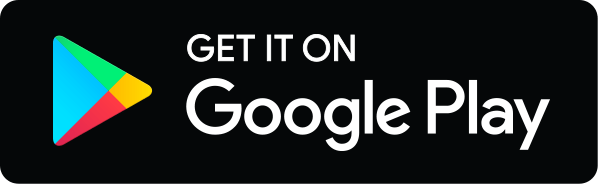