Acute myeloid leukemia (AML) is a molecularly heterogeneous disease and age-associated molecular alterations result in younger children harboring a distinct signature from older children and adolescents. Pediatric AML has a genetic and epigenetic profile with significant differences compared to adult AML. Somatic and epigenetic alterations contribute to myeloid leukemogenesis and can evolve from diagnosis to relapse. Cytogenetic alterations, somatic mutations and response to induction therapy are important in informing risk stratification and appropriate therapy allocation. Next-generation sequencing technologies are providing novel insights into the biology of AML and have the ability to identify potential targets for therapeutic intervention.
Key points
- •
Pediatric acute myeloid leukemia (AML) has a genomic and epigenetic profile distinct from that of adult AML.
- •
Somatic mutations and epigenetic alterations contribute to myeloid leukemogenesis, and can evolve from diagnosis to relapse.
- •
Next-generation sequencing technologies are providing novel insights into the biology of AML and are highlighting potential targets for therapeutic intervention.
- •
Cytogenetic alterations, somatic mutations, and response to induction therapy contribute to current risk stratification and appropriate therapy allocation.
Introduction
Acute myeloid leukemia (AML) is a hematopoietic malignancy that is the culmination of genetic and epigenetic alterations in the hematopoietic stem/progenitor cells, leading to dysregulation of critical signal transduction pathways and resulting in the expansion of undifferentiated myeloid cells. AML can be broadly divided into 2 categories, de novo AML and secondary AML. Secondary AML refers to evolution of AML subsequent to prior exposure to cytotoxic therapy or antecedent hematopoietic insufficiency (eg, myelodysplastic syndrome [MDS], marrow failure), leading to evolution of distinct karyotypic and molecular alterations including MLL translocations following exposure to topoisomerase inhibitors. In contrast to secondary AML, AML that evolves without a prior cytotoxic exposure is referred to as de novo AML.
Numerous somatic, karyotypic and molecular alterations have been identified in de novo AML; however, despite association of many of these alterations with clinical phenotype, most have no prognostic value, nor do they identify a specific target or a distinct pathway that can be readily exploited for therapeutic intervention. The observed age-associated evolution of molecular alterations reveals a profile for younger children with AML distinct from that of older children and adolescents with AML. Furthermore, the landscape of genetic alterations differs markedly from AML in adults and the paucity of potential therapeutic targets is more notable in childhood AML.
Introduction
Acute myeloid leukemia (AML) is a hematopoietic malignancy that is the culmination of genetic and epigenetic alterations in the hematopoietic stem/progenitor cells, leading to dysregulation of critical signal transduction pathways and resulting in the expansion of undifferentiated myeloid cells. AML can be broadly divided into 2 categories, de novo AML and secondary AML. Secondary AML refers to evolution of AML subsequent to prior exposure to cytotoxic therapy or antecedent hematopoietic insufficiency (eg, myelodysplastic syndrome [MDS], marrow failure), leading to evolution of distinct karyotypic and molecular alterations including MLL translocations following exposure to topoisomerase inhibitors. In contrast to secondary AML, AML that evolves without a prior cytotoxic exposure is referred to as de novo AML.
Numerous somatic, karyotypic and molecular alterations have been identified in de novo AML; however, despite association of many of these alterations with clinical phenotype, most have no prognostic value, nor do they identify a specific target or a distinct pathway that can be readily exploited for therapeutic intervention. The observed age-associated evolution of molecular alterations reveals a profile for younger children with AML distinct from that of older children and adolescents with AML. Furthermore, the landscape of genetic alterations differs markedly from AML in adults and the paucity of potential therapeutic targets is more notable in childhood AML.
Epidemiology of acute myeloid leukemia
AML is diagnosed in very young children and comprises nearly 25% of pediatric leukemias; however, it is far more prevalent in adults, and is generally considered a disease of older adults whereby the median age at diagnosis is nearly 70 years. Incidence of AML can be better appreciated by close evaluation of the most recent SEER (Surveillance, Epidemiology, and End Results) data. Initial evaluation demonstrates low incidence of AML in children and young adults, with a significant increase in older adults ( Fig. 1 A). However, closer examination of the data demonstrates highest incidence of AML in younger patients (<40 years) to be in infancy with an incidence of 1.6 cases per 100,000, similar to that in the fourth decade of life. After infancy, there is a declining incidence of approximately 0.12 cases per 100,000 per year in the first decade of life (see Fig. 1 B) to an incidence of 0.4 per 100,000 by age 10. In the following 3 decades, there is a steady increase in AML incidence of approximately 0.02 cases per 100,000 per year to 1.3 cases per 100,000 per year by age 45 years, nearly equivalent to that seen in infants. A substantial increase in the rate of AML diagnosis occurs in the fifth decade to nearly 10 times the observed rate in the previous 3 decades, to an incidence of 6.2 per 100,000 by age 65. After age 65, AML diagnosis increases again substantially, more than 30-fold higher than that seen in younger patients (age 10–40), likely attributable in part to its evolution from an underlying MDS. This epidemiologic observation parallels the underlying known and emerging karyotypic and genomic makeup of AML, and provides insight into the contribution of genomic alterations to the evolution of AML.
Inherited Susceptibility of Acute Myeloid Leukemia
Although AML is a rare event in childhood, there are a wide range of inherited chromosomal and gene defects and marrow failure syndromes that predispose to the development of AML. Some of the more common AML predisposition syndromes include trisomy 21 (Down syndrome [DS]), Fanconi anemia, dyskeratosis congenita (DC), Shwachman-Diamond syndrome (SDS), and Kostmann syndrome (severe congenital neutropenia or SCN). Down syndrome is associated with 10- to 20-fold increased risk of leukemia, as well as high incidence of development of transient myeloproliferative disorder (TMD) in the first 3 months of life that resembles AML, but in most cases the disease undergoes spontaneous resolution. Fanconi anemia is an autosomal recessive disorder caused by mutations in DNA repair genes, and has been estimated to show a cumulative incidence of AML/MDS of approximately 50% by age 40 years. DC is an X-linked disorder caused by mutations in the dyskerin gene, DKC1 , which is a key subunit of ribosomal RNA processing and the telomerase complex. Autosomal recessive forms of DC exist and are due to mutations in TERC , the RNA component of telomerase, or TERT , the enzymatic component of telomerase. SDS is an autosomal recessive disorder caused by mutations in the SBDS gene, which functions in ribosome processing and function and is characterized by neutropenia and pancreatic insufficiency. Kostmann syndrome is progressive neutropenia caused by defects in neutrophil elastase ( ELA2 ), and is associated with evolution into AML.
Genomic landscape of childhood acute myeloid leukemia
Until recently, most genomic events defined in children were derived from adult studies, thus demonstrating a lower prevalence or lack of such AML-associated genomic lesions in childhood AML. More recently, focused characterization on the somatic genomic changes in childhood AML have provided new insights into the biology of pediatric AML, affording an opportunity to begin to correlate these changes with clinical and biological phenotypes. To date, disease-associated genomic alterations, including translocations, numerical structural alterations (eg, monosomies, trisomies), or sequence variations (mutations, indels), have been used as powerful tools for prediction of response to therapy and outcome in a subset of patients.
Karyotypic Alterations
Cytogenetic alterations have been a cornerstone in the diagnosis of AML, and an invaluable tool for consideration of stratification for treatment based on risk categorization. Although a large number of chromosomal alterations can be identified in AML, most pediatric AML cases separate into distinct cytogenetic categories based on specific chromosomal events: 25% have t(8;21) or Inv(16)/t(16;16), which are collectively referred to as core binding factor AML (CBF AML); 12% have t(15;17); 20% have rearrangements involving the MLL gene; and 20% do not have a discernible karyotypic abnormality (normal karyotype). Such cytogenetic alterations have significant age-associated variations ( Fig. 2 ), with some highly prevalent in younger patients (eg, MLL ) and others more prevalent in teenagers (CBF). In addition to karyotypic alterations, disease-associated mutations have been identified in AML; greater than 90% of pediatric AML cases have at least 1 genomic alteration that can be detected by current methods, with the highest fraction occurring in those with a normal karyotype.
Subkaryotypic (cryptic) chromosomal abnormalities
Cytogenetic abnormalities involving large chromosomal regions can be detected by conventional cytogenetics. However, karyotypic analysis in AML is limited by the ability to culture leukemic cells in vitro before analysis in addition to the size of the region involved. Cryptic translocations, those not amenable to identification by conventional karyotyping, may require more specialized techniques such as polymerase chain reaction or fluorescence in situ hybridization studies (FISH) for identification. Several cryptic translocations, including NUP98/NSD1 , CBFA2T3/GLIS2 , and NUP98/KDM5A , have been discovered recently and shown to be prevalent in children with AML, and may provide significant insight into the pathogenesis of childhood AML. Segmental or whole chromosome deletions and their associated loss of heterozygosity (LOH) lead to haploinsufficiency of involved genes, and may contribute to malignant transformation. More recent sequencing and array technologies have identified regions of LOH without associated deletions (copy-neutral LOH [CN-LOH]), also referred to as acquired uniparental disomy, a homologous recombination-mediated process whereby a homozygous state is achieved after an initial acquisition of a heterozygous mutation. With the use of array and sequencing technologies, regions of CN-LOH have been shown to be prevalent in AML, but their contribution to malignant transformation or progression remains unclear.
Somatic Mutations in Childhood Acute Myeloid Leukemia
Somatic mutations in genes known to regulate hematopoiesis have been identified in a significant proportion of AML, and the presence of these mutations has been shown to be associated with clinical outcome. At present, numerous mutations have been implicated in AML pathogenesis, with the number rising with new discovery phase initiatives. At present, mutations in 3 genes ( FLT3 , NPM1 , and CEBPA ) have been shown to have clinical implications in childhood AML and have been incorporated in clinical trials as prognostic markers, therapeutic targets, or both.
FLT3 mutations
The most commonly mutated gene in childhood AML is FMS-like tyrosine kinase 3 ( FLT3 ), which results in constitutive activation of the receptor kinase activity, and can be due to the internal tandem duplication ( FLT3 /ITD) of the juxtamembrane domain coding sequence, or a missense mutation in the activation loop domain ( FLT3 /ALM). FLT3 /ITD is detected in 15% of all children with AML, and has been shown to be highly associated with poor response to induction chemotherapy and high relapse rate. Despite biological similarity to FLT3 /ITD, those with FLT3 /ALM do not have increased rates of treatment failure. Several studies have shown that patients with FLT3 /ITD who receive allogeneic stem cell transplantation in complete remission have an improved outcome, thus providing a rationale for therapy allocation in this high-risk cohort of patients. Furthermore, tyrosine kinase inhibitors (TKI) targeting FLT3 have shown efficacy in inducing high rates of remission in patients with FLT3 /ITD, although the long-term benefits of such interventions are yet to be determined. In contrast to FLT3 /ITD, FLT3 /ALM has not been shown to be similarly amenable to TKI-mediated FLT3 inhibition, which may be in part due to FLT3 conformational changes resulting from the distinct mutations and TKI-binding affinity.
Nucleophosmin (NPM1) mutations
NPM1 encodes a ubiquitously expressed molecular chaperone that shuttles rapidly between the nucleus and cytoplasm. Mutations in NPM1 are common in AML, with a prevalence of 30% in adult and 8% to 10% in pediatric AML. NPM1 mutations appear to be more prevalent in AML with normal karyotype, with a prevalence of nearly 40% to 50% in adults and 20% in pediatric AML. Disease-associated mutations, characterized by 4 base insertions in exon 12 of the NPM gene, lead to impaired nuclear localization of the nucleophosmin protein. Presence of NPM1 mutations portends a favorable outcome with reduced relapse risk and improved survival, similar to the favorable outcome achieved in patients with CBF AML. In pediatric AML, the presence of NPM1 mutations appears to overlap in a subset of patients with FLT3 /ITD, and its coexpression seems to partially ameliorate the poor prognosis conferred by FLT3 /ITD alone.
CEBPA mutations
The CEBPA gene encodes CCAAT/enhancer binding protein alpha (C/EBPa), which functions as a transcription factor that regulates granulocytic proliferation and terminal differentiation. Mutations in the CEBPA occur in approximately 5% of childhood AML, and most cases with this mutation occur in a biallelic manner whereby two distinct mutations occur, one in the N-terminal domain (NTD) and the second in the opposite allele affecting the bZip domain. Such a biallelic mutation results in the expression of a truncated protein, which has been shown to be sufficient for the development of AML. CEBPA mutations tend to occur in patients with normal cytogenetics, and are associated with decreased relapse risk and improved survival.
Epigenetic alterations
Some of the differences observed in gene and protein expression are regulated by alterations that are not encoded in the genome. Alterations in DNA methylation can lead to silencing of genes, similar to that of a functional mutation. Silencing of the CEBPA gene by promoter methylation appears to have a functional consequence similar to that seen with mutations in the CEBPA gene and, on a genome-wide scale, altered CpG methylation profiles have been associated with distinct subsets of adult AML. Aberrant methylation can be caused by genomic alterations (mutations, deletions, or translocations) in genes regulating methylation, including a large number of methyltransferase genes (eg, MLL1 , DNMT3A , TET2 ). The strategy of targeting aberrant methylation for therapeutic benefit with demethylating agents is currently under investigation.
Age-associated genomic variation in acute myeloid leukemia
There is a significant age-associated variation in the incidence of AML, with infants and older adults having an elevated incidence. There is a close association between the biology of AML and its age-based incidence, as evidenced by the significant variability of genomic alterations in AML from infancy to adulthood.
The age-based variation in the prevalence of structural and sequence variations is notable in childhood AML ( Figs. 3 and 4 ). Examination of the karyotypic alterations demonstrates significant age-based variation, with MLL translocations being highly prevalent in infants whereby those younger than 1 year harbor MLL alterations in nearly 60% of cases. This rapid evolution of disease, within weeks to months of birth, signifies the impact and high penetrance of MLL translocations in causing leukemia. The MLL prevalence declines over the following decade of life to less than 10%, similar to what is observed in younger adults (see Fig. 3 A). In addition, CBF AML is relatively rare in infants, but increases in prevalence to that seen in adults by the second decade of life. It is notable that CBF AML, which is associated with favorable outcome, decreases in prevalence in older adults. Similarly, patients with normal karyotype are rare in younger patients (likely owing to the high prevalence of MLL translocations), and their prevalence increases to nearly adult prevalence of nearly 50% in older pediatric patients.
The aforementioned alterations are present in nearly 70% of all childhood AML; however, less common structural alterations are described with variable biology and prognostic significance. Notable examples are monosomy 7 (-7), monosomy 5, and deletion 5q (-5/del5q), which cumulatively account for 2% to 4% of cases of childhood AML and greater than 10% of adult AML. Although specific genes in these chromosomal regions that contribute to disease pathogenesis have not been identified, the presence of these alterations is associated with resistance to chemotherapy and extremely poor survival.
Acute promyelocytic leukemia (APL), whereby normal hematopoiesis is replaced by immature granulocytes (promyelocytes), is the consequence of chromosomal translocation between chromosomes 15 and 17 (t(15;17)), involving the PML gene on chromosome 15 and the retinoic acid receptor alpha ( RARα or RARA ) gene on chromosome 17 (t(15;17)). The t(15;17) translocation juxtaposes the PML and RARA gene and leads to the expression of a novel fusion product, which is a potent recruiter of transcriptional repressors, resulting in the arrest of leukemic cells in the immature, promyelocyte stage.
Cryptic translocations that previously have gone undetected are emerging as significant contributors to childhood AML, with a relatively high prevalence in younger children and significant decline in adults (see Fig. 3 B). These cryptic structural events include translocations involving methyltransferase genes ( NSD1 and KDM5A ), which were not previously implicated in AML pathogenesis. Most notable is the fact that somatic mutations affecting the methylation pathway ( DNMT3A , IDH , TET2 ) are highly prevalent in adults but rare or absent in children (see Fig. 4 A), whereas structural alterations in methyltransferase genes are prevalent in young children but are rare or absent in adults (see Fig. 3 B). These data highlight the significant contribution of epigenetic derangements to myeloid leukemogenesis that is achieved by different mechanisms in children in comparison with adults.
Similar to the cytogenetic alterations, sequence variations also vary by age, and, as most of these mutations were identified in adults, these AML-associated genes are rare in pediatric AML. However, assessment of prevalence of these mutations has demonstrated paucity of these mutations at a younger age, with rapid evolution of these lesions to adult prevalence by the second decade of life. For example, mutations in FLT3 , NPM , and CEBPA are the most prevalent and clinically relevant mutations in childhood AML but are not usually detected in the first year of life, with increasing age-related prevalence to a level commensurate to what is observed in adults by the second decade of life (see Fig. 4 B).
In the past decade, advances in next-generation sequencing (NGS) technologies have revolutionized the ability to interrogate cancer genomes, culminating in whole-genome sequencing (WGS), whole-exome sequencing (WES), and transcriptome sequencing (RNA Seq). These approaches provide comprehensive coverage of most of the genome at a single base resolution of the genome, exome, or transcriptome, respectively. WGS, in addition to identifying sequence variations (SNVs and indels), provides additional layers of information, including structural alterations such as deletions, duplications, and inter- and intrachromosomal junctions. Transcriptome sequencing provides sequence and fusion data, in addition to mRNA expression levels that are not available by WGS. NGS technologies have rapidly transformed the genomic landscape in cancer by providing high-throughput, comprehensive genomic profiles in a large number of patients. Ley and colleagues identified IDH1 and DNMT3A to be 2 highly prevalent, clinically significant somatic alterations in adult AML. Despite confirmation of the prevalence and clinical utility of these mutations in adult AML, they appear to be rare or absent in childhood AML (see Fig. 2 ), highlighting the significant differences between AML in older and younger patients. More recent data from The Cancer Genome Atlas Research Network (TCGA) AML study demonstrated that 23 genes were significantly mutated in this adult cohort. Of the 23 genes, 14 are well-known mutations in AML that have also been well studied in childhood AML and, with the exception of a few ( FLT3 , NPM , WT1 ), have been shown to be rare events in childhood AML.
Based on the significant age-associated differences in somatic alterations in AML, a concerted effort has been devoted to the characterization of genomic and epigenomic profiles for the spectrum of childhood AML. Two such projects, the Children’s Oncology Group (COG)/National Cancer Institute (NCI) Therapeutically Applicable Research to Generate Effective Treatments (TARGET) AML initiative, and the St Jude/Washington University Pediatric Cancer Genome Project (PCGP), have initiated comprehensive evaluations of the genomic landscape of childhood AML using currently available NGS approaches. Early data emerging from these studies has provided new biological insights, and will only further the investigation of the molecular basis of childhood AML.
Already new insights have emerged from these projects. For instance, based on an analysis of RNA Seq, the St Jude PCGP project evaluated the transcriptome profile of a subset of patients with megakaryocytic leukemia (FAB M7), known to be associated with a poor outcome in children. This study identified a cryptic translocation between CBFA2T3 and GLIS2 genes in nearly 30% of children with FAB M7 AML, and further demonstrated that the CBFA2T3/GLIS2 fusion provides enhanced self-renewal capacity in colony-forming assay and that its presence is associated with adverse outcomes in FAB M7 AML. Subsequent studies have demonstrated the presence of this translocation in other AML subsets and its association with adverse outcome.
The COG/NCI-sponsored TARGET AML initiative is using WGS and RNA Seq to establish the genomic profile of childhood AML in a large cohort of children with AML and to establish the nature of genomic evolution of childhood AML from diagnosis to relapse. The pilot phase of the TARGET AML initiative used WES to evaluate diagnostic and relapse specimens from 20 patients who lacked karyotypic high-risk features to identify somatic alterations that contribute to AML pathogenesis, and to study genomic evolution from diagnosis to relapse. In this study, of the 195 distinct nonsynonymous mutations present in 180 genes, mutations in 6 genes were detected in more than 1 patient, including ETV6 , KIT , KRAS , and NRAS in 2 patients and TET2 or WT1 in 3 patients, suggesting that, in contrast to adult AML, there may not be a set of highly recurrent mutations in childhood AML. Although most of the identified mutations had been previously described as somatic alterations in pediatric AML, subsequent frequency validation of somatic mutations in ETV6 showed the mutations to be present in 6% of childhood AML and in less than 1% of adult AML. In this study the presence of ETV6 mutations, which were primarily observed in those without previously known risk factors, were highly associated with a significantly higher risk of relapse and worse survival. In addition to identification of the somatic mutations in AML at diagnosis, this study surveyed the genomic landscape at relapse compared with that seen at diagnosis, demonstrating significant clonal evolution from diagnosis to relapse, whereby nearly one-third of total mutations identified at diagnosis persisted at relapse with acquisition of a myriad of new mutations at relapse, indicating significant genomic evolution of diagnostic clone en route to relapse. Furthermore, a large number of diagnostic events, primarily minor and subclonal events, resolved from diagnosis to relapse. These findings were in line with observations in 8 matched diagnostic/relapse pairs previously reported by Ding and colleagues in adult AML using WGS, whereby they identified “founding” clones in the primary tumor that gained novel mutations evolving into the relapse clone. This observation signifies that in addition to the genomic alterations that may lead to disease evolution, additional events, whether genomic or epigenomic, may arise or be selected for, and may mediate resistance to therapy and the emergence of new leukemic clones with a genomic profile distinct from that of the diagnostic one.
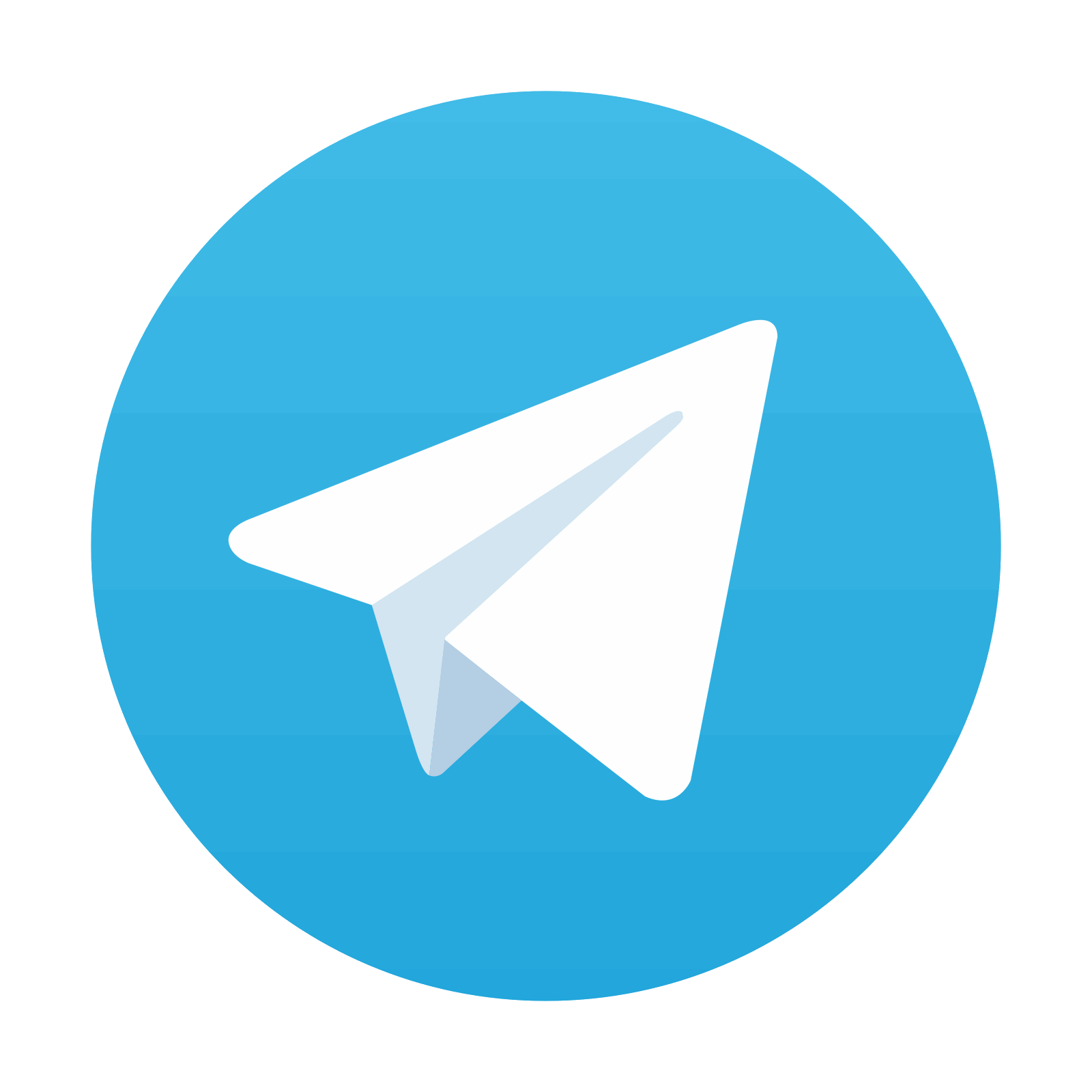
Stay updated, free articles. Join our Telegram channel

Full access? Get Clinical Tree
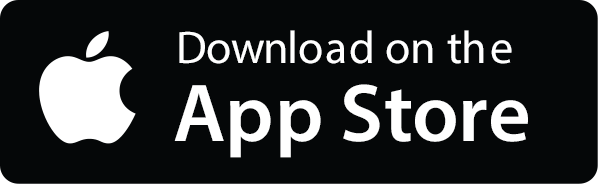
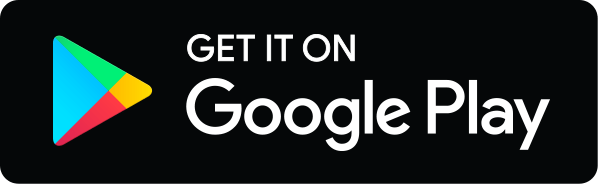