Onset
Acute
Oxygenation
ALI
PaO2/FiO2 < 300 (regardless of positive end-expiratory pressure level)
ARDS
PaO2/FiO2 < 200 (regardless of positive end-expiratory pressure level)
Chest radiograph
Bilateral infiltration seen on frontal chest radiograph
Pulmonary artery occlusion pressure
<18 mmHg when measured or no clinical evidence of left atrial hypertension
49.2 Pathophysiology
ALI is triggered by a local injury or infection directly in the lung (direct injury) or by overwhelming systemic inflammation (indirect injury). In children, viral and bacterial pneumonia and systemic sepsis are most common triggering events (Dahlem et al. 2003; Flori et al. 2005; Hammer et al. 1997). Other triggers for direct lung injury are aspiration of gastric contents, foreign bodies, or toxic agents such as hydrocarbons, smoke inhalation, near-drowning events, and pulmonary contusion. Indirect triggers of ALI include pancreatitis, systemic burn injury, and major trauma. After the initial injury, cell-mediated mechanisms cause a variety of reactions such as mediator release, activation of polymorphonuclear neutrophils, and initiation of the coagulation cascade. Alveolar-capillary cell damage and disturbance of the alveolar-capillary membrane cause high-permeability edema with flooding of the alveolar space and inactivation of surfactant (Bachofen and Weibel 1977, 1982).
In general, resolution occurs soon after the underlying disease (e.g., sepsis) is successfully treated. Usually within the second week of illness, inflammation ceases, alveolar-capillary function restores, surfactant production restarts, water clearance in lung tissue occurs, and, thus, lung compliance and gas exchange improve (Bernard et al. 1994; Marshall et al. 2000; Ware and Matthay 2000). Resolution of ALI is hampered in a proportion of patients. Histologically, they show pulmonary fibrosis with permanent loss of lung tissue and lung compliance (Bitterman 1992; Ingbar 2000; Shimabukuro et al. 2003). Figures 49.1 and 49.2 summarize the current understanding of the inflammatory scenario (Dahlem et al. 2007).
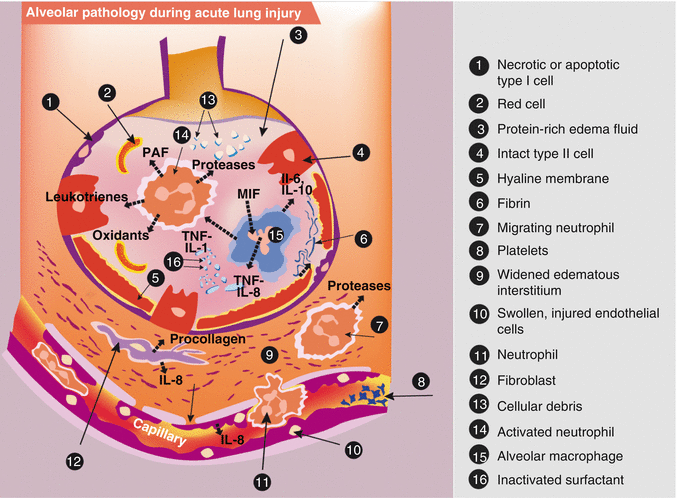
Fig. 49.1
Illustration of the acute phase of acute lung injury (ALI). In the acute phase of ALI, there is formation of protein-rich hyaline membranes on the denuded basement membrane. Neutrophils travel through the interstitium into the air space. Alveolar macrophages secrete interleukin-1, interleukin-6, interleukin-8, and interleukin-10, as well as tumor necrosis factor alpha (TNF–a) which stimulate and activate neutrophils. Neutrophils release pro-inflammatory molecules (oxidants, proteases, leukotrienes, platelet-activating factor (PAF)). The influx of protein-rich edema fluid into the alveolus has led to the inactivation of surfactant and, together with unresolved fibrin deposition, fibrin-rich hyaline membranes are formed (Adapted from Dahlem et al. (2007))
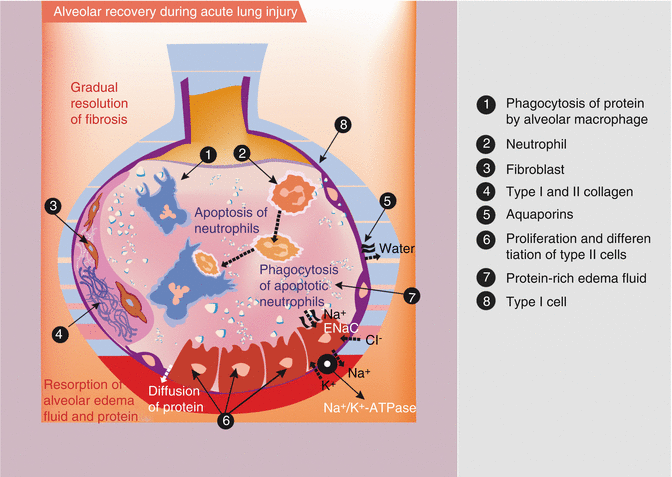
Fig. 49.2
Illustration of the recovery from acute lung injury. During recovery de novo proliferation and differentiation of alveolar type II cells occurs (on the right-hand side of the figure). For complete resolution, it is important that water is moving via additional aquaporines and by recovery of sodium and chloride channel function (ENaC) and sodium pump (Na+/K+-ATPase). Also intra-alveolar protein must be cleared by paracellular diffusion and secondarily by endocytosis. Furthermore, insoluble debris (protein, apoptotic neutrophils) is removed by macrophages. The gradual remodeling and resolution of intra-alveolar and interstitial granulation tissue and fibrosis are very important for complete recovery (left-hand side of the figure) (Adapted from Dahlem et al. (2007))
49.3 Lung Mechanics
From the first anatomical and functional characterizations of ARDS in adult patients until the present, ALI is recognized as a restrictive lung disease. Lung compliance is reduced due to accumulation of interstitial and alveolar plasma leakage, inactivation of surfactant, and alveolar collapse (Gunther et al. 1996; Petty and Ashbaugh 1971). These alterations are distributed inhomogeneously in all lung regions with a preponderance of dependent lung regions (Gattinoni et al. 1986). Distinct respiratory function testing in children with ALI confirms the restrictive abnormalities in lung mechanics in this age group (Newth et al. 1997). As a routine bedside assessment, an X-ray can visualize bilateral new infiltrates (Fig. 49.3) (Dahlem et al. 2007).
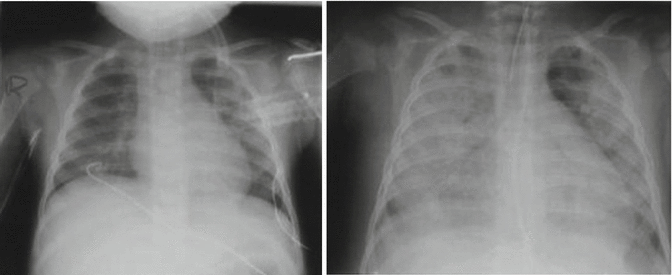
Fig. 49.3
A child with meningococcal septic shock and acute lung injury. Day 1 of ICU admission: the left-hand image shows bilateral infiltrates. Day 3 of ICU admission: the right-hand image shows progression to generalized infiltrates with pleural effusions (Adapted from Dahlem et al. (2007))
49.4 Epidemiology
Population-based studies revealed a prevalence of ALI in children that is between 2 and 16 per 100,000 person-years with an age-related increase (Bindl et al. 2005; Erickson et al. 2007). Mortality ranges between 18 and 27 % accounting, e.g., for up to 2,000 deaths per year in the USA (Dahlem et al. 2003; Flori et al. 2005; Peters et al. 1998; Randolph 2009). Boys are more prone to develop ALI than girls (Bindl et al. 2003). It appears that differences in lung mechanics and lung volume between the sexes might be responsible for this observation (Stocks et al. 1997). The exact mechanisms of this observation require further exploration.
49.5 Risk Factors
Five pediatric studies on the epidemiology of ALI have shown a correlation between the severity of hypoxia and mortality (Costil et al. 1995; Dahlem et al. 2003; Erickson et al. 2007; Flori et al. 2005; Goh et al. 1998). Commonly used markers of hypoxia such as the ratio of partial arterial oxygen pressure to fraction of inspired oxygen (P/F ratio) and the oxygenation index ((mean airway pressure x and fraction of inspired oxygen)/partial arterial oxygen pressure) are predictive of mortality. In four of these studies, the presence of multiple organ failure also showed a trend towards higher mortality in these children (Dahlem et al. 2003; Erickson et al. 2007; Flori et al. 2005; Goh et al. 1998).
49.6 Clinical Relevant Outcomes in Trials
Mortality is considered the most relevant primary end point for clinical trials investigating life-threatening disorders. Because of the low mortality in pediatric ALI, 2,000 children would be required in each ALI study arm to detect a moderate (25 %) decrease in mortality (α = 0.05, β = 0.80) (Randolph 2009). Because this is not feasible, non-mortality outcome measures are needed for clinical trials in ALI. Ventilator-free days (VFDs), respiratory function tests, cognitive deficits, and estimates of the quality of life might be acceptable end points due to their importance for health care economics and for the developing child (Ben Abraham et al. 2002; Dahlem et al. 2004a; Knoester et al. 2007; Schoenfeld and Bernard 2002).
49.7 Clinical Management of Acute Hypoxic Respiratory Failure
Due to hypoxia, children with ALI should receive oxygen supplementation immediately via a face mask. Additionally, the underlying disease (e.g., sepsis) should be treated vigorously and the general condition of the child should be stabilized by supportive therapy following the rules of intensive care therapy. With respect to respiratory failure, anatomic and physiological considerations in young children should be addressed. Children have smaller airways with higher airway resistance, lower functional residual capacity (FRC), and more rigid chest wall than adults. These characteristics in anatomy and lung mechanics put children with ALI at higher risk of severe hypoxic respiratory failure (Newth et al. 1997). Furthermore, 80 % of children with ALI develop hypoxic respiratory failure deteriorating rapidly within the first 48 h to the severe category of this syndrome, ARDS (Dahlem et al. 2003). Therefore, and not surprisingly, most children with ALI need mechanical ventilation (MV) during the course of the disease. In one study, it was shown that although a substantial fraction of children (28 %) did not require mechanical ventilation at the onset, 46 % of these patients eventually required MV for worsening ALI (Flori et al. 2005).
Disappointingly, no precise guidelines exist regarding initialization of MV except in situations with loss of consciousness or the need for airway protection. Depending on the underlying disease and the child’s general condition (e.g., decreased mental status, exhaustion due to high workload of breathing), clinicians should be ready at all times to institute mechanical ventilation while closely monitoring of vital parameters. Finally, appropriate MV can provide adequate ventilation volumes for clearance of CO2 and restoring FRC for oxygenation.
Before initializing MV and choosing appropriate ventilator settings, intensive care physicians in particular are obliged to ensure that they are optimizing the benefit of the therapy and minimizing the risk of inflicting secondary harm (Hippocratic Oath, Greek: ἐπὶ δηλήσει δὲ καὶ ἀδικίῃ εἴρξειν). Against this background and the knowledge of deleterious complications during the early years of MV, investigations focused on the dark side of MV. Convincing evidence has accrued over the last three decades that MV – despite the potential benefits and life-saving aspects – can damage the lungs and can kill patients (Kumar et al. 1973). What is now called ventilator-induced lung injury (VILI) shows similar histopathological and biochemical alterations as original ALI (American Thoracic Society, The European Society of Intensive Care Medicine, and The Societe de Reanimation de Langue Francaise 1999; Slutsky 2005).
The lungs are damaged by shear forces created by ventilation with high VT (volutrauma) in situations where alveolar collapse (atelectrauma) occurs after each respiratory breath. In combination with ALI, VILI aggravates the clinical course and increases mortality of ALI patients. Many experimental studies with lung injury models have shown that ventilator settings should be chosen to keep the lung open (atelectrauma) and to avoid high tidal volumes. Theoretically, this can be achieved by institution of ventilation within a safe – i.e., the most compliant – zone of the pressure-volume curve (Fig. 49.4) (American Thoracic Society, The European Society of Intensive Care Medicine, and The Societe de Reanimation de Langue Francaise 1999; Slutsky 2005). Identifying this safe zone in actual practice is more difficult. Despite some controversy, clinical and experimental studies suggest that in patients with severe ARDS, lung-protective ventilation is established by sufficient positive end-expiratory pressure (PEEP), plateau pressure below 30 cm H2O, and tidal volume (Vt) below 6 mL/kg (The Acute Respiratory Distress Syndrome Network 2000; Hager et al. 2005; Petrucci and Iacovelli 2007). FiO2 should be kept as low as possible to achieve adequate SpO2 and titrated according to a systematic approach with PEEP to avoid oxygen toxicity. In the ARDS-network trial, adult patients with ARDS showed improved survival with this ventilation strategy. Controlled clinical studies to reproduce these results in children were regarded as not feasible due to the low incidence and mortality of pediatric ALI (Hanson and Flori 2006). Alternatively, comparison with historical controls has shown that the implementation of the high PEEP and low Vt approach already changed ventilation practice on PICUs and might be responsible for the improvement of outcomes during the past two decades (Albuali et al. 2007; Miller and Sagy 2008). Although not designed to examine ventilation strategy, a pediatric ALI study investigating the effects of prone position during MV also applied lung-protective ventilator settings. In the control group with an average Vt not higher than 7 mL/kg and an average PEEP between 7 and 10 cmH2O, the lowest mortality rate yet of only 8 % was reported (Curley et al. 2005).
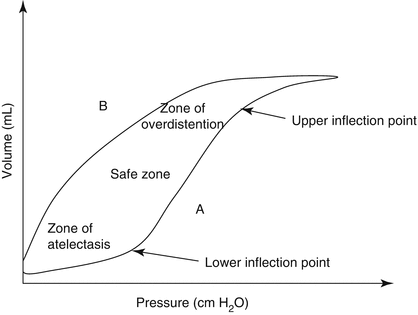
Fig. 49.4
The hysteresis of the pressure-volume curve of the lung. A inflation limb; B deflation limb. On inflation (A) lungs need higher pressures to inflate than on deflation. On deflation (B), the higher lung volume can be maintained on lower pressure. Thus, once the lung is open, it is more compliant. At the lower inflection point, the lung opens up, compliance improves, and, at the upper inflection point, optimal lung volume is achieved. At overinflation, compliance decreases and lung injury occurs (Adapted from Dahlem et al. (2007))
Alternatively to conventional pressure ventilation, high-frequency oscillatory ventilation (HFOV) is thought to be the ideal ventilation technique in patients with ALI. HFOV should prevent VILI by reducing Vt to a minimum of dead-space volume and by a continuous distending pressure guaranteeing FRC. Despite positive experiences of single centers, controlled data of a single randomized controlled trial (RCT) showed only improvement in oxygenation but no improvement in survival (Arnold et al. 1994, 2000).
Mechanical ventilation in children requires endotracheal intubation by experienced and skilled personal. Thereby, cuffed tubes are safe and prevent air leakage due to volume loss especially in lungs with very low compliance and high ventilator settings (Clements et al. 2007). Endotracheal intubation, however, carries risks such as tube misplacement, injuries to the airway, the need for deep sedation, and pneumonia (Page et al. 1998). Therefore, noninvasive ventilation methods gained increasing attention and were subjected to clinical trials in adult patients, with no conclusive evidence in favor of use. Recently, a Cochrane review examined the use of continuous negative extrathoracic pressure and continuous positive airway pressure ventilation as noninvasive modes of respiratory support. They found that there are no controlled studies in children on noninvasive modes of respiratory support available; therefore, no definite conclusions can be drawn on this topic at the moment (Shah et al. 2008).
Vital organs (brain, heart, liver, and kidney) are sensitive to hypoxia. However, oxygen mixed to the inspired gas is considered toxic with the potential hazards for organ damage (Capellier et al. 1999). Therefore, oxygen concentrations in arterial blood should be monitored closely by measuring percutaneous oxygen saturation (SpO2) or arterial oxygen concentrations (PaO2). To avoid aggressive ventilator settings, reasonable target values of SpO2 88–95 % and PaO2 55–88 torr or strategies accepting even lower values are routinely applied; however, these lack validation of potential harm to the developing brain. In this context, it has also been recommended to accept higher values of PaCO2 and lower pH to keep plateau pressure and Vt as low as possible (Kavanagh and Laffey 2006). However, similarly, there are no appropriate outcome studies in children with ALI supporting these strategies.
Appropriate ventilator settings can preserve FRC. Computerized tomography studies of ARDS lungs examined dependent lung regions in dorsal parts of the lung during supine positioning (Gattinoni et al. 1986). Gravity and loss of compliance predispose to alveolar collapse. It was shown that by turning the patient around into prone position, these regions can be kept patent or reopened, thus improving lung mechanics and oxygenation (Pelosi et al. 2002). In children, these positive physiological effects have been confirmed (Numa et al. 1997). Disappointingly, and consistent with trials in adults with ALI, the largest RCT did not show benefits in the most relevant outcome variables such as mortality or ventilator-free days (Casado-Flores et al. 2002).
49.8 Adjunctive Therapies
MV is only a supportive measure. Therapies should address the pathophysiological alterations in ALI. Hypoxia in ALI is caused by ventilation/perfusion mismatch with increased intrapulmonary shunting and imbalance in pulmonary blood flow. Selective pulmonary vasodilatation is, therefore, considered one promising therapeutic option. Inhaled nitric oxide (INO) and aerosolized prostacyclin (PGI2) are commonly used therapeutics for this indication (Adhikari et al. 2007; Dahlem et al. 2007). In the only RCT in children with severe hypoxic respiratory failure including cases of ALI, INO improved hypoxia by at least 20 % (Dobyns et al. 1999). Inhaled PGI2 showed similar physiological effects in one RCT (Dahlem et al. 2004b). However, both studies did not show positive effects on clinical relevant outcomes, although they were not designed to answer these questions.
Surfactant inactivation and disruption of production is one of the major consequences of pulmonary inflammation during ALI (Gunther et al. 1996). A first RCT of surfactant installation in children with ALI improved oxygenation and reduced mortality (Willson et al. 2005). Efficacy appears to depend on the cumulative dose and timing of application. The PALISI network (http://pedsccm.org/PALISI_network.php) is now studying the effects of calfactant and lucinactant in children with ALI (Randolph 2009).
Another approach for a causative therapy is to slow down or inhibit the inflammatory alterations during ALI. Results from adult ALI/ARDS studies with corticosteroids raised more questions than gained answers. It seems that within a narrow (low dose) and a well-defined timing range (early in the course), corticosteroid therapy has the potential to improve outcome (Steinberg et al. 2006). To date, there has been no clinical trial of steroids for ALI in children.
49.9 Mechanical Ventilation Failure
In the absence of appropriate causative therapies and with the knowledge of the difficulties to design the best ventilator settings to prevent VILI, one should support the lungs until critical illness has abated. Extracorporeal membrane oxygenation (ECMO) has shown to bridge this period facilitating oxygenation and CO2 removal in desperate situations of pediatric hypoxic respiratory failure and ALI (Goldman et al. 1997; Green et al. 1996). Parallel to the extensive requirements to install ECMO and the fact that it is not available on every PICU, most physicians regard ECMO as a rescue therapy for desperate cases. In these children with an expected mortality of almost 100 %, ECMO can save lives in approximately 50 % of such cases. Due to high costs, bleeding complications, and decreasing mortality in pediatric ALI, attempts to conduct a RCT led to early termination (Spear and Fackler 1998).
49.10 General Supportive Care
Children with ALI are critically ill as devastating underlying illness deregulates many crucial physiological processes leading to life-threatening abnormalities. The mainstay of supportive care in children with ALI is treatment on a well-equipped PICU with appropriate monitoring and respiratory machines and special trained nurses and physicians. Correction of the most important alterations is reasonable and occurs daily; however, no RCTs have yet shown cutoff values for therapies with regard to improving outcome (Dahlem et al. 2007; Randolph 2009; Randolph et al. 2003). One should also not disregard the hazards of overtreatment (Cochrane Injuries Group Albumin Reviewers 1998; Sanchez and Toy 2005). Despite the large gap in scientific data for evidence-based recommendations, the authors offer in Table 49.2 an overview of a practical approach to routine management.
Table 49.2
Recommendations for routine management of children with ALI beside routine critical management
Data to |
Recommended guide use |
Promising preliminary data |
May be applied on individual trial basis |
Option for rescue therapy
![]() Stay updated, free articles. Join our Telegram channel![]() Full access? Get Clinical Tree![]() ![]() ![]() |
---|