Polycystic ovary syndrome (PCOS), a heterogeneous syndrome of unknown aetiology, is the leading cause of anovulation, hirsutism and infertility in women. This multifactorial syndrome emerges at puberty and has cardiovascular and metabolic sequelae through menopause. The common features of normal puberty, namely menstrual irregularities and insulin resistance, obscure the diagnosis of adolescent PCOS, while there are no established diagnostic criteria for PCOS in this age group. The clinical implications of PCOS diagnosis in adolescents remain unclear. Experts in the field still ponder whether PCOS should be managed at such a young age with a view to hindering the long-term sequelae of the syndrome.
Prevalence – Definition
The prevalence of polycystic ovary syndrome (PCOS) in adult reproductive-aged women has been established at the level of 6.5–6.8%, according to the National Institute of Health (NIH) criteria. However, the prevalence of the syndrome in the adolescent population has not been specifically studied. A cross-sectional study reported a 3% prevalence of clinical PCOS in girls randomly selected from Iranian high schools. PCOS was diagnosed by clinical criteria if menstrual dysfunction and clinical hyperandrogenism were detected ; however, the prevalence could have been higher if hormonal parameters were included in the diagnostic criteria, as is the case from studies in adult women. The definition of PCOS remains an issue of debate. PCOS was initially defined by a NIH conference in 1990 as the combination of chronic anovulation or oligomenorrhoea and clinical or biochemical hyperandrogenism. The Rotterdam consensus in 2003 revised the diagnostic criteria and declared two of the three following criteria as prerequisites for PCOS: chronic anovulation or oligomenorrhoea, clinical or biochemical hyperandrogenism and polycystic ovarian morphology. In 2006, the Androgen Excess Society provided a contemporary version of the definition of PCOS. The final statement has highlighted hyperandrogenism, clinical or biochemical, in combination with ovarian dysfunction, including both functional and ultrasonographic abnormalities, as the core characteristics of PCOS. Since there are no established criteria for the diagnosis of PCOS in adolescents, the adult criteria are applied to adolescents as well.
Signs and symptoms of PCOS typically appear at the time of puberty. However, the clinical and laboratory features of PCOS simulate the physiological features of puberty. The normal variant of adolescence, characterised by transient postmenarcheal anovulation and multicystic ovaries, is not easily distinguishable from the persistent anovulation and the polycystic ovarian morphology related to PCOS. Nevertheless, following close investigation of oligomenorrhoeic adolescents, it seems that a substantial proportion of these girls have biochemical markers typical of PCOS and eventually develop the further clinical features of the syndrome. It is remarkable that almost 50% of the oligomenorrhoeic adolescents at the age of 15 years remain oligomenorrhoeic by the age of 18 years, thus challenging the notion that oligomenorrhoea is a normal variant in the teen years.
Ovarian imaging is also problematic in this age group, since the transvaginal ultrasound approach is not applicable to virginal adolescents. Most importantly, polycystic ovaries should be distinguished from multicystic ovaries, which occur normally during puberty. The lack of increased stroma and the larger size of cysts in the latter may be used to distinguish multicystic from polycystic ovaries. Moreover, clinical hyperandrogenism, manifested typically by hirsutism and scored using the Ferriman–Gallwey scale, varies by time since puberty. Considering that the Ferriman–Gallwey scale was standardised for adult white women, it may be less accurate in adolescents.
In addition, the definition of biochemical hyperandrogenaemia in adolescents remains arbitrary due to the paucity of normative data for the adolescent population. Adding to the complexity, the laboratory measurement of androgen levels, particularly total and free testosterone, is limited by methodological flaws. There are high inter-laboratory and intra-laboratory variabilities, especially for testosterone levels below 100 ng dl −1 . A proficiency survey by the American College of Pathologists noted a coefficient of variability of 34.9% for evaluation by several immunoassay instruments, in different laboratories, of a test sample containing testosterone concentrations in the normal range for adult women.
Pathogenesis of PCOS
PCOS is considered to arise as a complex trait with contributions from both heritable and environmental factors. The genetic basis of the syndrome is reinforced by accumulating data showing the familial clustering of PCOS components, like hyperandrogenism and insulin resistance. PCOS appears to be a multigenic trait, although the contributing genes remain undefined as yet. The pathogenesis of the syndrome involves primary theca cell defects as well as neuroendocrine dysfunction of the hypothalamic-pituitary-ovarian axis leading to hyperandrogenemia.
The natural course of PCOS in adolescents
In view of the potential congenital origins of the syndrome, research focus has been placed on prenatal life to identify risk factors for PCOS. Gestational factors, such as birth weight, have been incriminated. Specifically, small-for-gestational-age (SGA), as well as large-for-gestational-age (LGA), infants have been postulated to be the earliest precursors of PCOS in postnatal life. Intrauterine growth retardation leading to low birth weight (LBW), or alternatively, excess nutrient supply to foetus leading to high birth weight (HBW) may initiate developmental pathways which culminate in PCOS. In the first postnatal years, rapid weight gain in SGA girls and sustained adiposity in LGA girls may precipitate premature adrenarche, the putative precursor of PCOS, characterised by hyperandrogenism, visceral obesity and insulin resistance. Adiposity appears to have a key role in this pathogenic sequence ,as discussed in a recent review proposing the adipose tissue expandability hypothesis . The exhaustion of the capacity to expand subcutaneous AT in a metabolically safe way leads to hyperinsulinemia and finally to hyperinsulinemic androgen excess .
Unfolding the putative natural course of the syndrome from foetal life to adulthood ( Fig. 1 ), premature pubarche is considered a forerunner of PCOS. Premature pubarche refers to the appearance of sexual hair before the age of 8 years in girls. Girls with premature pubarche appear to carry a 15–20% risk of developing PCOS. The risk seems to be relatively high in those with exaggerated adrenarche indicated by the sulphated version of dehydroepiandrosterone (DHEA-S) levels in the range of 130–185 μg dl −1 and/or androstenedione levels exceeding the range of 75–99 ng dl −1 . Moreover, atypical central precocious pubarche may pose a risk for PCOS. The respective risk in girls with ordinary premature adrenarche indicated by DHEA-S levels in the range of 40–130 μg dl −1 or idiopathic premature pubarche, indicated by DHEA-S less than 40 μg dl −1 , or in those with ordinary central precocious pubarche does not appear to be significantly elevated.
In adolescence and adulthood, the final PCOS phenotype arises. Women with a history of HBW are bound to demonstrate the complete triad of hyperandrogenism, anovulation and polycystic ovaries, while their counterparts with LBW may be more likely to demonstrate hyperandrogenism and anovulation but normal ovaries on ultrasound. This evolutionary pathway of PCOS from foetal life to adulthood remains speculative, and this should be further explored.
The pathogenetic role of androgen excess
Hyperandrogenism appears to play a key role in the pathogenesis of PCOS, contributing to the reproductive and metabolic aspects of the syndrome.
Intrauterine androgen excess may be the earliest gestational factor linked with the pathogenesis of PCOS. Experimental studies in prenatally androgenised monkeys constructed the basis for the theory of foetal programming of PCOS by intrauterine androgen excess. In these elegant studies, prenatal exposure to androgen excess disturbed the neuroendocrine regulation of the hypothalamic–pituitary–ovarian axis, leading to increased luteinising hormone (LH) production and, consequently, to increased ovarian androgen production.
By inhibiting the negative-feedback of ovarian steroids on gonadotropin-releasing hormone (GnRH)–LH secretion, hyperandrogenaemia may increase LH secretion. Progesterone, oestrogen and androgen normally exert suppressive effects on GnRH–LH secretion. However, in PCOS, the feedback loops between the hypothalamus, the pituitary and the ovary are disturbed. Consequently, PCOS has been associated with persistently rapid GnRH pulse frequency, attributed to impaired ovarian steroid feedback on the hypothalamic–pituitary secretion. Hypothalamic secretion may be minimally responsive not only to progesterone, which is deficient in PCOS, but also to androgens which are in excess. The defect of progesterone inhibition was found to be reversed with the use of the androgen receptor blocker flutamide, indicating that androgen excess may be a causative factor. However, only half of the HA girls were found to have impaired progesterone sensitivity similar to that seen in adult women with PCOS, while the others maintained normal sensitivity despite equivalent degrees of hyperandrogenaemia. This differential response remains under investigation. Differences in hormonal, demographic, genetic or metabolic parameters may contribute to the differences in hypothalamic sensitivity to progesterone. If applicable to humans, this model raises the question about the putative intrauterine sources of intrauterine androgen excess in the absence of congenital virilising disorders. Counter-intuitively, possible androgen exposure of the female foetus, caused by a shared intrauterine environment with a male foetus, did not result in PCOS-like traits. Nor does maternal hyperandrogenism appear to be a plausible mechanism, since the placental aromatase acts as a foetal barrier to maternal androgens. Nevertheless, there are data from hyperandrogenic pregnant women showing a positive correlation of maternal serum androgen levels with foetal androgen excess and intrauterine growth delay. However, androgen excess is recognised as a primary abnormality in PCOS, independent from hypothalamic–pituitary neurodysregulation. Hyperandrogenism in PCOS arises primarily from hyperactive theca steroidogenesis. The underlying ovarian defect seems to be a constitutive overproduction of androgenic steroids, mainly 7-hydroxyprogesterone and androstenedione.
Hyperandrogenism at the intra-ovarian level is causatively linked with anovulation in PCOS. Intra-ovarian androgen excess may impair folliculogenesis in a dual fashion, by stimulating the growth of small follicles and by hindering follicular maturation towards the dominant stage.
In addition, androgen excess may hinder gonadotropin-induced oestrogen and progesterone synthesis in the PCOS follicle. The androgen-induced inhibition of aromatase activity may contribute to the distortion of the later stages of folliculogenesis. This abnormality may lead to the failure of mono-ovulation, since the oestrogen peak is required for the suppression of follicle-stimulating hormone (FSH) levels, which allows for the selection of the dominant follicle.
In addition to androgen-induced ovulatory dysfunction, hyperandrogenaemia that persists from early life to adulthood may cause alterations in body composition and upper body fat may become more pronounced, inducing insulin resistance in early adulthood.
Intrauterine androgen exposure may predispose to metabolic PCOS-like traits in adulthood. Visceral fat accumulation, which is linked with insulin resistance, beta-cell dysfunction, hyperlipidaemia and type 2 diabetes are the main phenotypic PCOS-like traits reproduced in prenatally androgenised female rhesus monkeys.
The role of hyperinsulinaemia and the ‘metabolic phenotype’ of PCOS in adolescents
Insulin resistance and hyperinsulinaemia may be a key mechanism underlying this perpetual sequence of hormonal and metabolic aberrations in adolescents. This sequence may commence early and extend throughout life. Hyperinsulinism may be a familial characteristic of PCOS children (or at least girls), although it may not appear until the later stages of puberty. During puberty, insulin sensitivity decreases while insulin requirements increase. Basal insulin levels are higher at the end of puberty (Tanner stages 4 and 5) than during prepuberty or adulthood. This suggests a role for hyperinsulinaemia in the development of PCOS, which occurs during this stage of life. There are contradictory data as to whether hyperandrogenism is the result or the cause of hyperinsulinism in PCOS or else which of the two abnormalities precedes the other one. A study by van Hooff et al. has shown that a majority of adolescents with oligomenorrhoea and hyperandrogenaemia are not hyperinsulinaemic, suggesting that in PCOS, hyperandrogenism may precede hyperinsulinaemia. Another study suggested that the presence of hyperinsulinaemia in adolescents with hyperandrogenaemia and oligomenorrhoea depends on the presence of obesity. Definite proof is still lacking, as fasting insulin indices markers are only surrogate markers for hyperinsulinism and insulin resistance.
In association with hyperinsulinaemia/insulin resistance, PCOS adolescents demonstrate metabolic abnormalities which are most evident in overweight/obese PCOS adolescents. The metabolic phenotype of PCOS in adolescents may include lipid and carbohydrate abnormalities and may precede the metabolic phenotype of PCOS in adulthood.
Obese PCOS adolescents were reported to have an increased prevalence of glucose intolerance with decreased first-phase insulin secretion, adjusted for ambient insulin resistance and increased endogenous glucose production.
In general, hyperandrogenaemia should be considered as the primary abnormality in PCOS, mainly deriving from an inherent defect in the ovarian theca steroidogenesis. Hyperinsulinaemia is also a common feature, which is attributable to the inherent association of PCOS with insulin resistance. Hyperinsulinaemia and insulin resistance are superimposed upon the pre-existing ovarian dysfunction. Therefore, in PCOS, insulin resistance and hyperinsulinaemia accentuate androgen overproduction and anovulation, while also contributing to metabolic abnormalities.
The role of obesity and environmental factors
Obesity often co-exists with PCOS. It is estimated that 40–60% of women with PCOS are overweight or obese, with greater abdominal or visceral adiposity compared with weight-matched controls. However, there is widespread variability in the degree of adiposity in women with PCOS by geographic location and ethnicity. In studies in Spain, China, Italy and the United States, 20%, 43%, 38% and 69%, respectively, of women with PCOS were noted to be obese.
The role of pre- and peripubertal obesity as a contributing factor in PCOS development has been steadily gaining ground. Environmental factors, mainly nutrition, influence the clinical and biochemical phenotype of PCOS in genetically predisposed individuals. Intractable prepubertal obesity has been associated with severe insulin resistance syndromes that heralded adolescent PCOS in four cases. More ordinary manifestations of insulin resistance, such as the metabolic syndrome (MBS) may predispose to PCOS. Pre- and early-pubertal obese girls have been reported to have a subtle, sub-clinical increase in testosterone that normalises with substantial weight loss. Obesity, particularly of early onset, appears to unmask the inherent susceptibility to PCOS, mainly by accentuating insulin resistance. The resultant hyperinsulinaemia acts synergistically with LH to amplify ovarian theca cell androgen production. Most interestingly, insulin has been demonstrated to directly stimulate androgen biosynthesis by theca cells.
In addition, adipose tissue is a major site for the direct formation of testosterone from circulating steroid precursors. Obesity is a major determinant of the metabolic phenotype in PCOS adolescents, while lean juveniles appear to be protected from the metabolic consequences of PCOS. However, a recent study by Rossi et al. in adolescents with and without PCOS has shown that the prevalence of MBS, a cluster of central obesity, hyperglycaemia, hypertension and dyslipidaemia, is mostly affected by obesity rather than the PCOS per se .
A major strength of this study was that control women were directly enrolled from a similar population of young women who were of similar weight with documented endocrine and menstrual history. The latter study has challenged earlier data reported by Coviello et al. Using the same criteria for the paediatric definition of MBS, Coviello et al. reported increased rates of MBS in their PCOS subjects compared with a national dataset. However, the study by Coviello et al. had two major limitations. First, the group of PCOS adolescents and the national dataset were not matched for body mass index (BMI). Second, the investigators have failed to assess the endocrine characteristics of the control population, as neither menstrual data nor androgens were recorded in the database for controls.
In summary, it is possible that the impact of obesity is a more significant contributor to the prevalence of MBS in adolescents than PCOS/endocrine status. However, prospective studies may reveal the potential independent impact of PCOS on the cardiometabolic aspects later in life.
Not only the quantity (calorie excess leading to obesity), but also the quality of food may contribute to the pathogenesis of PCOS. Advanced glycated end products (AGEs) are oxidative molecules, which are abundant in Westernised diet and certain types of food, and partly account for the detrimental role of modern diet in cardiometabolic disease. A high-AGE beverage has been shown to induce pro-inflammatory and pro-atherogenic cascades in non-diabetic humans and circulating levels of AGEs have been associated with several markers of cardiometabolic disease. Thus, the finding of increased serum AGEs levels in lean, non-diabetic women with PCOS may bear significant cardiometabolic implications.
The reported positive association of serum AGEs with serum androgen levels in PCOS women extend the potential role of AGEs in reproductive aspects as well. Most strikingly, since nutritional excess of AGEs in normal rats was shown to accumulate in ovarian tissue, these molecules may exert potentially direct ovarian actions, further indicated by significant correlation of AGEs with anti-Műllerian hormone (AMH), a granulosa cell-derived hormone, in humans.
Pathogenesis of PCOS
PCOS is considered to arise as a complex trait with contributions from both heritable and environmental factors. The genetic basis of the syndrome is reinforced by accumulating data showing the familial clustering of PCOS components, like hyperandrogenism and insulin resistance. PCOS appears to be a multigenic trait, although the contributing genes remain undefined as yet. The pathogenesis of the syndrome involves primary theca cell defects as well as neuroendocrine dysfunction of the hypothalamic-pituitary-ovarian axis leading to hyperandrogenemia.
The natural course of PCOS in adolescents
In view of the potential congenital origins of the syndrome, research focus has been placed on prenatal life to identify risk factors for PCOS. Gestational factors, such as birth weight, have been incriminated. Specifically, small-for-gestational-age (SGA), as well as large-for-gestational-age (LGA), infants have been postulated to be the earliest precursors of PCOS in postnatal life. Intrauterine growth retardation leading to low birth weight (LBW), or alternatively, excess nutrient supply to foetus leading to high birth weight (HBW) may initiate developmental pathways which culminate in PCOS. In the first postnatal years, rapid weight gain in SGA girls and sustained adiposity in LGA girls may precipitate premature adrenarche, the putative precursor of PCOS, characterised by hyperandrogenism, visceral obesity and insulin resistance. Adiposity appears to have a key role in this pathogenic sequence ,as discussed in a recent review proposing the adipose tissue expandability hypothesis . The exhaustion of the capacity to expand subcutaneous AT in a metabolically safe way leads to hyperinsulinemia and finally to hyperinsulinemic androgen excess .
Unfolding the putative natural course of the syndrome from foetal life to adulthood ( Fig. 1 ), premature pubarche is considered a forerunner of PCOS. Premature pubarche refers to the appearance of sexual hair before the age of 8 years in girls. Girls with premature pubarche appear to carry a 15–20% risk of developing PCOS. The risk seems to be relatively high in those with exaggerated adrenarche indicated by the sulphated version of dehydroepiandrosterone (DHEA-S) levels in the range of 130–185 μg dl −1 and/or androstenedione levels exceeding the range of 75–99 ng dl −1 . Moreover, atypical central precocious pubarche may pose a risk for PCOS. The respective risk in girls with ordinary premature adrenarche indicated by DHEA-S levels in the range of 40–130 μg dl −1 or idiopathic premature pubarche, indicated by DHEA-S less than 40 μg dl −1 , or in those with ordinary central precocious pubarche does not appear to be significantly elevated.
In adolescence and adulthood, the final PCOS phenotype arises. Women with a history of HBW are bound to demonstrate the complete triad of hyperandrogenism, anovulation and polycystic ovaries, while their counterparts with LBW may be more likely to demonstrate hyperandrogenism and anovulation but normal ovaries on ultrasound. This evolutionary pathway of PCOS from foetal life to adulthood remains speculative, and this should be further explored.
The pathogenetic role of androgen excess
Hyperandrogenism appears to play a key role in the pathogenesis of PCOS, contributing to the reproductive and metabolic aspects of the syndrome.
Intrauterine androgen excess may be the earliest gestational factor linked with the pathogenesis of PCOS. Experimental studies in prenatally androgenised monkeys constructed the basis for the theory of foetal programming of PCOS by intrauterine androgen excess. In these elegant studies, prenatal exposure to androgen excess disturbed the neuroendocrine regulation of the hypothalamic–pituitary–ovarian axis, leading to increased luteinising hormone (LH) production and, consequently, to increased ovarian androgen production.
By inhibiting the negative-feedback of ovarian steroids on gonadotropin-releasing hormone (GnRH)–LH secretion, hyperandrogenaemia may increase LH secretion. Progesterone, oestrogen and androgen normally exert suppressive effects on GnRH–LH secretion. However, in PCOS, the feedback loops between the hypothalamus, the pituitary and the ovary are disturbed. Consequently, PCOS has been associated with persistently rapid GnRH pulse frequency, attributed to impaired ovarian steroid feedback on the hypothalamic–pituitary secretion. Hypothalamic secretion may be minimally responsive not only to progesterone, which is deficient in PCOS, but also to androgens which are in excess. The defect of progesterone inhibition was found to be reversed with the use of the androgen receptor blocker flutamide, indicating that androgen excess may be a causative factor. However, only half of the HA girls were found to have impaired progesterone sensitivity similar to that seen in adult women with PCOS, while the others maintained normal sensitivity despite equivalent degrees of hyperandrogenaemia. This differential response remains under investigation. Differences in hormonal, demographic, genetic or metabolic parameters may contribute to the differences in hypothalamic sensitivity to progesterone. If applicable to humans, this model raises the question about the putative intrauterine sources of intrauterine androgen excess in the absence of congenital virilising disorders. Counter-intuitively, possible androgen exposure of the female foetus, caused by a shared intrauterine environment with a male foetus, did not result in PCOS-like traits. Nor does maternal hyperandrogenism appear to be a plausible mechanism, since the placental aromatase acts as a foetal barrier to maternal androgens. Nevertheless, there are data from hyperandrogenic pregnant women showing a positive correlation of maternal serum androgen levels with foetal androgen excess and intrauterine growth delay. However, androgen excess is recognised as a primary abnormality in PCOS, independent from hypothalamic–pituitary neurodysregulation. Hyperandrogenism in PCOS arises primarily from hyperactive theca steroidogenesis. The underlying ovarian defect seems to be a constitutive overproduction of androgenic steroids, mainly 7-hydroxyprogesterone and androstenedione.
Hyperandrogenism at the intra-ovarian level is causatively linked with anovulation in PCOS. Intra-ovarian androgen excess may impair folliculogenesis in a dual fashion, by stimulating the growth of small follicles and by hindering follicular maturation towards the dominant stage.
In addition, androgen excess may hinder gonadotropin-induced oestrogen and progesterone synthesis in the PCOS follicle. The androgen-induced inhibition of aromatase activity may contribute to the distortion of the later stages of folliculogenesis. This abnormality may lead to the failure of mono-ovulation, since the oestrogen peak is required for the suppression of follicle-stimulating hormone (FSH) levels, which allows for the selection of the dominant follicle.
In addition to androgen-induced ovulatory dysfunction, hyperandrogenaemia that persists from early life to adulthood may cause alterations in body composition and upper body fat may become more pronounced, inducing insulin resistance in early adulthood.
Intrauterine androgen exposure may predispose to metabolic PCOS-like traits in adulthood. Visceral fat accumulation, which is linked with insulin resistance, beta-cell dysfunction, hyperlipidaemia and type 2 diabetes are the main phenotypic PCOS-like traits reproduced in prenatally androgenised female rhesus monkeys.
The role of hyperinsulinaemia and the ‘metabolic phenotype’ of PCOS in adolescents
Insulin resistance and hyperinsulinaemia may be a key mechanism underlying this perpetual sequence of hormonal and metabolic aberrations in adolescents. This sequence may commence early and extend throughout life. Hyperinsulinism may be a familial characteristic of PCOS children (or at least girls), although it may not appear until the later stages of puberty. During puberty, insulin sensitivity decreases while insulin requirements increase. Basal insulin levels are higher at the end of puberty (Tanner stages 4 and 5) than during prepuberty or adulthood. This suggests a role for hyperinsulinaemia in the development of PCOS, which occurs during this stage of life. There are contradictory data as to whether hyperandrogenism is the result or the cause of hyperinsulinism in PCOS or else which of the two abnormalities precedes the other one. A study by van Hooff et al. has shown that a majority of adolescents with oligomenorrhoea and hyperandrogenaemia are not hyperinsulinaemic, suggesting that in PCOS, hyperandrogenism may precede hyperinsulinaemia. Another study suggested that the presence of hyperinsulinaemia in adolescents with hyperandrogenaemia and oligomenorrhoea depends on the presence of obesity. Definite proof is still lacking, as fasting insulin indices markers are only surrogate markers for hyperinsulinism and insulin resistance.
In association with hyperinsulinaemia/insulin resistance, PCOS adolescents demonstrate metabolic abnormalities which are most evident in overweight/obese PCOS adolescents. The metabolic phenotype of PCOS in adolescents may include lipid and carbohydrate abnormalities and may precede the metabolic phenotype of PCOS in adulthood.
Obese PCOS adolescents were reported to have an increased prevalence of glucose intolerance with decreased first-phase insulin secretion, adjusted for ambient insulin resistance and increased endogenous glucose production.
In general, hyperandrogenaemia should be considered as the primary abnormality in PCOS, mainly deriving from an inherent defect in the ovarian theca steroidogenesis. Hyperinsulinaemia is also a common feature, which is attributable to the inherent association of PCOS with insulin resistance. Hyperinsulinaemia and insulin resistance are superimposed upon the pre-existing ovarian dysfunction. Therefore, in PCOS, insulin resistance and hyperinsulinaemia accentuate androgen overproduction and anovulation, while also contributing to metabolic abnormalities.
The role of obesity and environmental factors
Obesity often co-exists with PCOS. It is estimated that 40–60% of women with PCOS are overweight or obese, with greater abdominal or visceral adiposity compared with weight-matched controls. However, there is widespread variability in the degree of adiposity in women with PCOS by geographic location and ethnicity. In studies in Spain, China, Italy and the United States, 20%, 43%, 38% and 69%, respectively, of women with PCOS were noted to be obese.
The role of pre- and peripubertal obesity as a contributing factor in PCOS development has been steadily gaining ground. Environmental factors, mainly nutrition, influence the clinical and biochemical phenotype of PCOS in genetically predisposed individuals. Intractable prepubertal obesity has been associated with severe insulin resistance syndromes that heralded adolescent PCOS in four cases. More ordinary manifestations of insulin resistance, such as the metabolic syndrome (MBS) may predispose to PCOS. Pre- and early-pubertal obese girls have been reported to have a subtle, sub-clinical increase in testosterone that normalises with substantial weight loss. Obesity, particularly of early onset, appears to unmask the inherent susceptibility to PCOS, mainly by accentuating insulin resistance. The resultant hyperinsulinaemia acts synergistically with LH to amplify ovarian theca cell androgen production. Most interestingly, insulin has been demonstrated to directly stimulate androgen biosynthesis by theca cells.
In addition, adipose tissue is a major site for the direct formation of testosterone from circulating steroid precursors. Obesity is a major determinant of the metabolic phenotype in PCOS adolescents, while lean juveniles appear to be protected from the metabolic consequences of PCOS. However, a recent study by Rossi et al. in adolescents with and without PCOS has shown that the prevalence of MBS, a cluster of central obesity, hyperglycaemia, hypertension and dyslipidaemia, is mostly affected by obesity rather than the PCOS per se .
A major strength of this study was that control women were directly enrolled from a similar population of young women who were of similar weight with documented endocrine and menstrual history. The latter study has challenged earlier data reported by Coviello et al. Using the same criteria for the paediatric definition of MBS, Coviello et al. reported increased rates of MBS in their PCOS subjects compared with a national dataset. However, the study by Coviello et al. had two major limitations. First, the group of PCOS adolescents and the national dataset were not matched for body mass index (BMI). Second, the investigators have failed to assess the endocrine characteristics of the control population, as neither menstrual data nor androgens were recorded in the database for controls.
In summary, it is possible that the impact of obesity is a more significant contributor to the prevalence of MBS in adolescents than PCOS/endocrine status. However, prospective studies may reveal the potential independent impact of PCOS on the cardiometabolic aspects later in life.
Not only the quantity (calorie excess leading to obesity), but also the quality of food may contribute to the pathogenesis of PCOS. Advanced glycated end products (AGEs) are oxidative molecules, which are abundant in Westernised diet and certain types of food, and partly account for the detrimental role of modern diet in cardiometabolic disease. A high-AGE beverage has been shown to induce pro-inflammatory and pro-atherogenic cascades in non-diabetic humans and circulating levels of AGEs have been associated with several markers of cardiometabolic disease. Thus, the finding of increased serum AGEs levels in lean, non-diabetic women with PCOS may bear significant cardiometabolic implications.
The reported positive association of serum AGEs with serum androgen levels in PCOS women extend the potential role of AGEs in reproductive aspects as well. Most strikingly, since nutritional excess of AGEs in normal rats was shown to accumulate in ovarian tissue, these molecules may exert potentially direct ovarian actions, further indicated by significant correlation of AGEs with anti-Műllerian hormone (AMH), a granulosa cell-derived hormone, in humans.
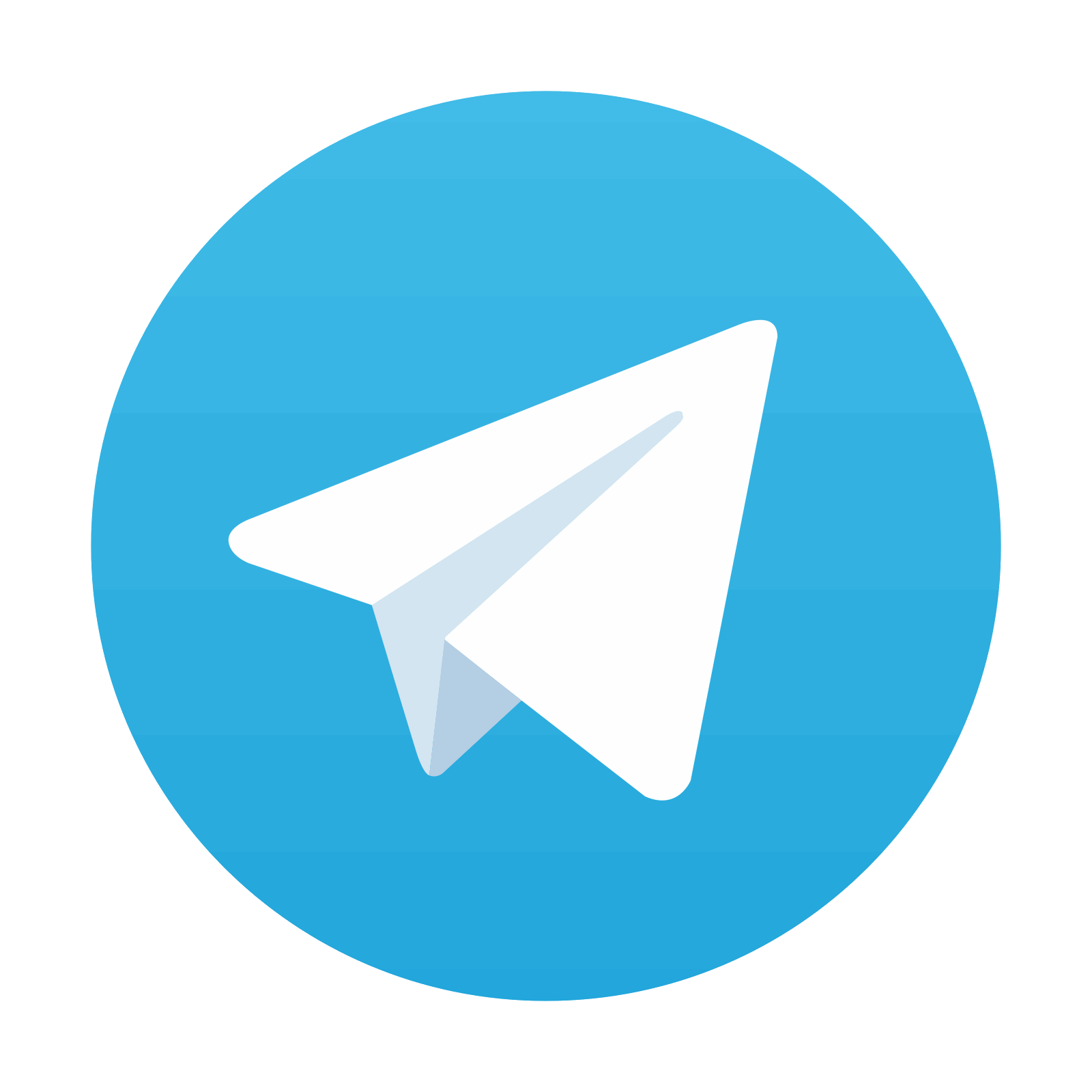
Stay updated, free articles. Join our Telegram channel

Full access? Get Clinical Tree
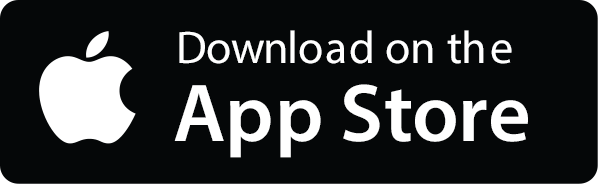
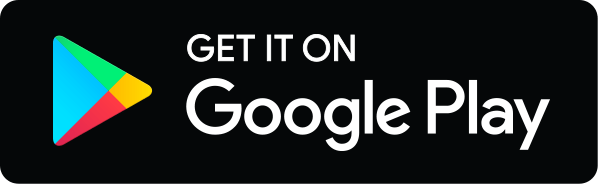