- •
Important interactions between the infant and the ventilator occur routinely during invasive and noninvasive mechanical ventilation. Some of these interactions can have significant effects on ventilation and gas exchange and may prolong the need for respiratory support.
- •
Asynchrony between the ventilator and the infant’s breathing effort, excessive peak inflation pressure, or inspiratory time that is too long can affect the infant’s respiratory rhythm and lead to agitation.
- •
Ventilator waveforms and monitored parameters should be used to determine the adequacy of ventilator settings and detect any adverse condition such as autotriggering.
- •
The interaction between the infant and new modes of ventilation that become available should be carefully examined before they are widely used.
Mechanical ventilation is an important tool in the management of premature infants with respiratory failure but is associated with an increased risk of acute and chronic lung injury and other associated morbidities. Despite continued efforts to reduce its use, a large proportion of preterm infants eventually require intubation and mechanical ventilation.
The earlier association between mechanical ventilation and the risk for lung injury was primarily believed to be due to aggressive use of the ventilator, which produced injurious lung expansion and chronic lung damage. In part because of the limitations in the older ventilators, clinicians for years elected to impose a respiratory pattern on their patients to take over their ventilation completely. To achieve this, neonates with respiratory failure were sedated, paralyzed, or underwent hyperventilation to suppress their spontaneous respiratory drive. These approaches were associated with many problems that led to prolonged ventilator dependence and higher rates of complications. The likelihood of hyperventilation or hypoventilation is higher during controlled ventilation because the settings are not always adjusted to match the infant’s metabolic demands and changing respiratory mechanics. Moreover, neonates who do not exercise the respiratory muscles for long periods are less likely to be weaned successfully from mechanical ventilation.
Neonatal ventilatory support has evolved from controlled ventilation to assisted ventilation with the evolution of various modes of synchronized ventilation. These gentler strategies have been developed to take maximal advantage of the patient’s own respiratory effort and ameliorate ventilator-associated lung injury. These strategies involve careful management to minimize ventilator support to avoid volume injury and shorten the duration of mechanical ventilation. As a result, the ventilatory support provided to premature infants has changed from a strategy to fully control ventilation, targeting normal blood gas values, to a less aggressive approach in which the ventilator is used to supplement the infant’s spontaneous respiratory effort. During the course of the mechanical ventilatory support, there is continuous interaction between infant and the ventilator. Some of these interactions can have negative effects on the infant’s spontaneous breathing effort and affect adversely ventilation and gas exchange, whereas other interactions can be beneficial. This chapter describes these interactions, their effects, and discusses alternatives to enhance positive interactions.
Patient-Ventilator Interaction During Conventional Mechanical Ventilation
The interaction between the patient and the ventilator can be extremely complex because it is influenced by many factors, including the patient’s respiratory drive, various respiratory reflexes, the mechanical characteristics of the respiratory system, and the timing, flow, and pressure characteristics of the ventilator inflations. Respiratory reflexes influence the infant’s spontaneous respiratory rhythm. Activation of the Hering-Breuer vagal inhibitory reflex by lung inflation can shorten neural inspiration, whereas its activation by lung inflation during neural expiration will delay the onset of the next spontaneous inspiration. Also active in the newborn, Head’s paradoxical reflex can be activated by a rapid lung inflation and elicit a greater inspiratory effort. This could result in a greater transpulmonary pressure, larger tidal volume (V t ), and risk of alveolar overdistention. These interactions are illustrated in Fig. 16.1 .

Infant-Ventilator Asynchrony
Asynchrony between the infant and the ventilator occurs frequently during intermittent mandatory ventilation (IMV) because mechanical inflations are delivered at fixed intervals and duration, which do not coincide with the infant’s spontaneous inspiration. The ventilator positive pressure cycles can interact with the infant’s spontaneous breathing and reflex activity. The effects vary depending on the timing and volume of the spontaneous inspiration or positive-pressure inflation.
Inspiratory asynchrony occurs when the ventilator inflation is delivered toward the end of the spontaneous inspiration and extends beyond the end of inspiration. Fig. 16.2 shows an example of asynchrony during the spontaneous inspiratory phase. The resulting inspiratory hold can affect the spontaneous respiratory rate, and the additional lung inflation on top of the spontaneous breath may cause volutrauma. Expiratory asynchrony occurs when the ventilator inflation is delivered during exhalation and prolongs the spontaneous expiratory phase, in turn affecting the spontaneous breathing frequency, as shown in Fig. 16.3 . This type of asynchrony can also elicit active exhalation against an elevated pressure at the airway, producing a rise in intrathoracic and probably also intracranial pressure.


Asynchrony between the infant’s spontaneous breathing and the ventilator inflations can have multiple effects, including poor gas exchange, air leaks, and increased risk of intraventricular hemorrhage (IVH).
Asynchrony was common in earlier generations of neonatal ventilators. Monitoring was limited to visual assessment of chest expansion and breathing rate, with limited ability to determine asynchrony or the adequacy of V T . Manipulation of the ventilator inspiratory and expiratory time has been suggested as a strategy to decrease asynchrony by adapting to the infant’s breathing pattern. This, however, requires frequent adjustment of these settings because of the variability of the infant’s breathing. Use of high ventilator rates has also been suggested as a way to prevent asynchrony. However, this may not be the most adequate approach for premature infants because of the risk of hypocapnia and its association with IVH and periventricular leukomalacia.
Patient-Ventilator Interaction During Synchronized Mechanical Ventilation
Because of the serious drawbacks of controlled ventilation, there has been an almost complete shift toward the use of assisted (i.e., synchronized) ventilation modes. The patient’s spontaneous respiratory drive is not inhibited, so infants are more likely to be weaned sooner from mechanical ventilation because their respiratory muscles remain fit and can cope with the increased work of breathing during the weaning process. Because the ventilator is used only to supplement the infant’s respiratory effort, lower peak inflation pressure (PIP) is needed to maintain adequate minute ventilation. The likelihood of hyperventilation is also reduced by allowing the infant to determine the total minute ventilation.
Ventilators used to provide assisted ventilation must respond on a timely basis to the demands of even the smallest infants. Incorporation of sensors and microprocessors for monitoring and control of different functions has made possible the development of ventilators capable of synchronizing the ventilator positive pressure cycle with the infant’s spontaneous inspiration (i.e., patient-triggered ventilation).
The use of synchronized ventilation to assist the infant’s spontaneous breathing while avoiding the effects of asynchrony can lead to a gentler ventilatory strategy and preservation of the infant’s breathing rhythm. During synchronized ventilation, cycling of the ventilator shortly after the onset of the spontaneous inspiration achieves a larger transpulmonary pressure because of the sum of the positive-pressure cycle to the negative pressure generated by the diaphragm. This produces a larger V T than that generated by the infant or the ventilator alone, as illustrated in Fig. 16.4 . The positive interaction between the infant and the ventilator during inflation explains the better gas exchange and ventilation with more consistent V T and lower breathing effort during synchronized than during conventional ventilation. One of the most consistent and important advantages of patient-triggered ventilation is that the preservation of spontaneous respiration facilitates weaning from the ventilator and shortens the duration of mechanical ventilation. This explains the improved respiratory outcome with synchronized ventilation, which was more striking among the smaller infants at higher risk for bronchopulmonary dysplasia. Synchronous ventilation has also been shown to reduce the stress response and fluctuations in blood pressure and oxygenation in preterm infants.

Methods of Synchronization
Synchronization of the ventilator cycle with spontaneous inspiration is achieved by using different methods to detect inspiratory activity. Their efficacy and reliability vary, and these performance characteristics significantly influence the interaction between the ventilator and the infant’s spontaneous inspiratory effort.
Mainstream (proximal) or internal flow sensors are used in neonatal ventilators to detect the inspiratory flow generated by the infant’s spontaneous inspiratory effort. The ventilator cycle starts when the inspiratory flow exceeds a set threshold (see Fig. 16.4 ). Flow triggering has been shown to be more sensitive and specific than other methods; the ability to use low flow thresholds for triggering make this method appealing for use in sicker and more immature infants. Flow triggering is limited by the presence of gas leaks around the endotracheal tube. Leaking gas travels through the flow sensor in the same direction as the inspiratory flow and may produce autocycling if this exceeds the trigger threshold. This is less of a problem with some modern ventilators designed specifically for neonatal use, which have effective compensation for even moderately large endotracheal tube leaks.
Although mainstream flow sensors are usually small, they increase the instrumental dead space and may affect carbon dioxide (CO 2 ) elimination, particularly in smaller infants. This effect, however, is relatively small and is outweighed by the advantages of flow triggering.
Outward motion of the abdomen during inspiration can be detected by a pressure capsule applied on the abdominal surface. The use of this capsule for ventilator triggering is relatively simple but requires individualized sensitivity adjustment to avoid autocycling during patient activity. This capsule has been used effectively to synchronize the ventilator during invasive and noninvasive respiratory support in the past but is no longer available in the United States.
The transmission of the negative pressure changes produced by spontaneous inspiration to the airway can also be used for triggering. However, because of their respiratory disease and relatively weak respiratory pump, preterm infants do not consistently produce the pressure changes at the airway required for triggering, which can lead to lack of or delayed triggering with these systems. Using the electrical activity of the diaphragm (EA DIA ) is an attractive way of triggering ventilator inflations because it eliminates the trigger delay occasioned by pneumatic coupling. Onset of inflation is triggered by an increase in the electrical potential generated by diaphragmatic muscle contraction, as sensed by a special feeding catheter with an array of miniature electrodes. Inflation is terminated when EA DIA declines below a fixed threshold, resulting in optimal synchrony for both initiation and termination of ventilator inflation. EA DIA is unaffected by leakage, making it uniquely suited for synchronization during noninvasive positive-pressure ventilation. Cost and availability (it is currently only available from one manufacturer) are limiting factors for more widespread use of this promising triggering modality.
Modalities of Synchronized Ventilation
Synchronized IMV
Synchronized IMV (SIMV) is similar to conventional IMV but with synchronous delivery of ventilator cycles. In both IMV and SIMV, the number of ventilator cycles delivered every minute is set by the clinician but the interval between cycles (expiratory duration, Te), which is constant in IMV, is variable in SIMV. Fig. 16.4 illustrates how synchrony is achieved during SIMV, and Fig. 16.5 shows a more regular ventilatory pattern during SIM compared with IMV. The disadvantage of SIMV in small preterm infants with narrow endotracheal tubes and insufficient muscle strength is that these immature infants often do not achieve adequate V T with spontaneous breaths that are in excess of the supported rate. These small breaths largely rebreathe dead-space gas and contribute little to alveolar minute ventilation, which often necessitates a larger V T for the low rate of SIMV inflations.

Assist/Control Ventilation
In assist/control (A/C) ventilation, every spontaneous inspiratory effort is assisted with a mechanical inflation. These synchronous inflations reduce the work of breathing and improve V T , as illustrated in Fig. 16.6 . Because all breaths are supported and easily clear dead space, the V T needed with A/C is substantially lower than with SIMV. Most preterm infants have an inconsistent respiratory drive. Hence, in A/C ventilation, a backup IMV rate is necessary to prevent hypoventilation during episodes of apnea. Backup ventilator cycles delivered during apnea may not always prevent hypoventilation if the rate is insufficient; optimally the backup rate is set only 10 cycles below the infant’s spontaneous effort to avoid large fluctuations in minute ventilation. On the other hand, a backup rate too near the infant’s breathing frequency or above it may lead to ventilator takeover if it provides all the required minute ventilation. In some neonatal ventilators, the duration of inspiration (Ti) in A/C ventilation is set by the operator, and in others, the ventilator cycle can be automatically terminated in synchrony with the declining inspiratory flow at the end of inspiration. This latter arrangement is also known as flow cycling. This allows the preterm infant to increase breathing frequency without shortening Te and affecting V T , unlike A/C ventilation with a set Ti. In most ventilators, the mode of A/C with flow cycling is referred to as pressure support ventilation (see the next section).

Pressure-Support Ventilation
Pressure-support ventilation (PSV) is a flow-cycled modality in which, as in A/C ventilation, every breath is assisted and the positive pressure is automatically terminated when the patient ends inspiration. This modality gives the infant complete control of the frequency and Ti. The synchronous support pressure is aimed at compensating for the loads induced by reduced lung compliance and increased airway and endotracheal tube resistance.
A consistent respiratory drive is needed to ensure maintenance of ventilation in PSV, but if apnea occurs, a backup IMV rate prevents hypoventilation. In many ventilators PSV can be combined with SIMV and thus the spontaneous breaths are pressure-supported, eliminating some of the problems of SIMV. Because the aim of PSV is mainly to boost the V T of spontaneous breaths, the pressure-supported breaths are usually assisted with lower pressures and result in smaller V T s than breaths assisted with SIMV. However, the level of pressure support should be sufficient to achieve a physiologic V T , of at least 3.5 to 4 mL/kg or it will not be effective due to a continued high dead space-to- V T ratio of the spontaneous breaths.
The addition of PSV to SIMV in preterm infants is aimed at boosting the spontaneous breaths and thus reducing the reliance on the larger SIMV inflations. PSV used with SIMV has been shown to reduce breathing effort and increase V T in proportion to the support pressure used. The combined use of SIMV and PSV was found to accelerate weaning in preterm infants compared with SIMV alone, preferentially in infants weighing more than 700 g at birth. This advantage is likely due to these infants’ more consistent respiratory drive, which ensures effective triggering of the ventilator.
Infant-Ventilator Maladaptation
Although synchronized ventilation can unload the respiratory pump by sharing the respiratory workload, the opposite occurs when there is maladaptation between the patient and the ventilator and the infant “fights” the ventilator. This lack of adaptation can be due to inadequate function of the synchronization mechanism, which leads to delayed triggering or trigger failure, autocycling, end-inspiratory asynchrony, or flow starvation. These problems may vary among ventilators according to their triggering methods and other characteristics, but there is also great variability during routine clinical practice, depending on the infant population, the underlying lung disease, and the ventilator settings.
Long Inspiratory Time and End-Inspiratory Asynchrony
End-inspiratory asynchrony occurs when the Ti of the ventilator cycle exceeds the patient’s neural inspiration or when there is delayed triggering and the mechanical cycle starts late during spontaneous inspiration. Continuation of the mechanical inflation into neural expiration results in a prolonged inspiratory plateau similar to an inspiratory hold and decreases the time for unopposed exhalation. In some cases, this can elicit active exhalation efforts against the positive pressure. The long Ti can produce a prolonged volume plateau that keeps the lung distended, delays the initiation of the next spontaneous inspiration, and affects the infant’s breathing rhythm (illustrated in Fig. 16.7 ). This effect is mediated by the Hering-Breuer inhibitory reflex.

In A/C ventilation, an excessive Ti may also result in an inverse inspiration-to-expiration ratio, insufficient expiratory time, and gas trapping, if the spontaneous breathing frequency increases ( Fig. 16.8 ). This can limit breathing frequency and disrupt the neural breathing pattern. To prevent this situation, in addition to avoiding a Ti that is too long, mechanical inflations can be terminated automatically based on the decline of the inspiratory flow below a set threshold, known as termination criteria. Fig. 16.9 shows an example of automatic termination of Ti (flow cycling as used in PSV). In ventilators not specifically designed for newborn infants, this automatic termination of ventilator inflations may not work properly when a large gas leak around the endotracheal tube is present, because the leak can maintain the measured inspiratory flow above the breath termination criteria. Manual adjustment of the termination criteria is possible to deal with this issue, but because the leak is often variable, this is difficult. Setting the termination criteria too high (e.g., 30% or 40% of peak flow) would lead to premature breath termination when the leak decreases. Specialized neonatal ventilators have effective leak compensation that eliminates this problem. On the other hand, a set Ti that is shorter that the infant’s spontaneous inspiration can result in inadequate transpulmonary pressure and insufficient V T . Flow cycling largely eliminates this problem by automatically adjusting the Ti in response to changing lung mechanics and spontaneous inspiratory time of the infant.

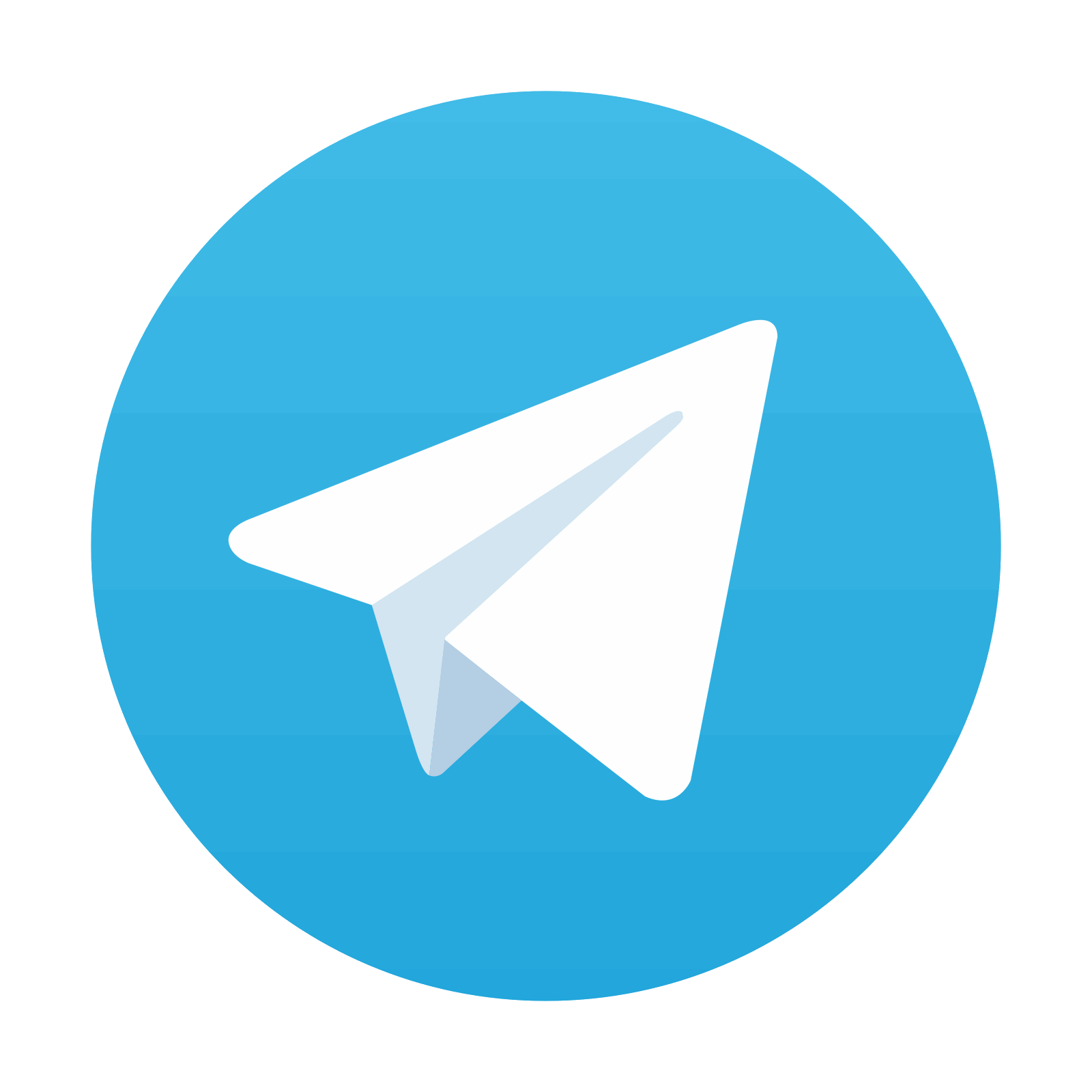
Stay updated, free articles. Join our Telegram channel

Full access? Get Clinical Tree
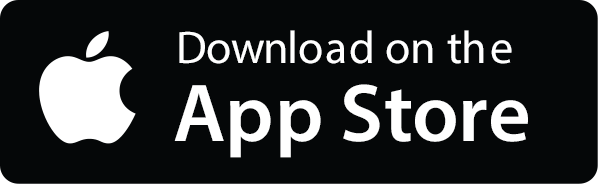
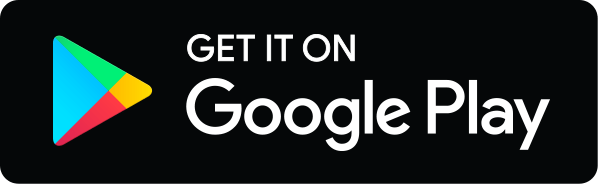
