A foetus exposed to oxygenation compromise is capable of several adaptive responses, which can be categorised into those affecting metabolism and those affecting oxygen transport. However, both the extent and duration of the impairment in oxygenation will have a bearing on these adaptive responses. Although intrapartum events may account for no more than one-third of cases with an adverse neurological outcome, they are important because they can be influenced successfully. This review describes the mechanisms underlying foetal hypoxia during labour, acid–base balance and gas exchange, and the current scientific understanding of the role of intrauterine asphyxia in the pathophysiology of neonatal encephalopathy and cerebral palsy. Although the mechanisms involved include similar initiating events, principally ischaemia and excitotoxicity, and similar final common pathways to cell death, there are certain unique maturational factors that influence the type and pattern of cellular injury.
Highlights
- •
Foetuses exhibit cardiovascular, metabolic and behavioural response to hypoxia.
- •
Gradually developing hypoxia affecting the brain damages typically the grey matter.
- •
Acute severe hypoxia damages the basal ganglia while sparing the cerebral cortex.
- •
The severity of damage is determined by the occurrence hypoxic-induced hypotension.
All human cells require oxygen and glucose to maintain aerobic metabolism and for energy production. The foetal oxygen requirement is determined by foetal size, activity and essential metabolic processes. During foetal life, the oxygen supply is dependent entirely on maternal respiration and circulation, placental perfusion, gas exchange across the placenta and the umbilical and foetal circulations. If the supply and requirement are in balance, the foetus has adequate oxygen to metabolise glucose aerobically to produce the energy required for organ function.
During the human birth process, the foetus is squeezed through the birth canal over a period of up to several hours, during which the head sustains considerable pressure and the foetus is intermittently deprived of oxygen. A reduced oxygen supply is often categorised into three types: (1) hypoxaemia, involving a reduced oxygen in the arterial blood, but where cell and organ function are not usually affected; (2) hypoxia, with reduced oxygen and subsequent anaerobic metabolism, mainly in the peripheral tissues; and (3) asphyxia, where the hypoxia extends to the central organs – the heart, brain and adrenal glands – and potentially leads to metabolic acidosis.
The main direct causes of neonatal death worldwide are estimated to be preterm birth, severe infections and asphyxia . Asphyxia is one of the main causes of neonatal and childhood mortality and morbidity, and it has been associated with neonatal death , hypoxic–ischaemic encephalopathy (HIE), seizures , intraventricular haemorrhage, cerebral palsy (CP) and delayed development .
The foetal blood supply
The placenta acts as the foetus’s lungs, kidneys and gastrointestinal tract, allowing gas exchange, waste elimination and nutrient uptake via the mother’s blood supply. In the intervillous space, oxygenated blood from the mother’s spiral arteries flows around the foetal chorionic villi, which contain deoxygenated blood. The two umbilical arteries transport deoxygenated blood and waste products from the foetus to the placenta, whereas the umbilical vein provides the foetus with oxygenated blood and nutrients from the mother. The neonatal acid–base status is therefore best reflected by the umbilical arterial blood, whereas the venous umbilical blood contents depend on the maternal acid–base status and placental function.
The foetal cardiovascular system is designed such that the most highly oxygenated blood is delivered to the myocardium and brain. These circulatory adaptations are achieved in the foetus by both the preferential streaming of oxygenated blood and the presence of intracardiac and extracardiac shunts. Thus, the foetal circulation can be defined as a ‘shunt-dependent’ circulation.
Blood from the placenta passes via the umbilical vein almost unhindered through the ductus venosus across the right atrium, and through the foramen ovale to the left atrium and then to the left ventricle, which pumps into the aortic arch and neck and head vessels. The heart is equipped with baroreceptor and volume receptor, which sense changes in the pressure and volume of blood in the heart. The aortic arch and carotid bodies, which contain chemoreceptors, are well positioned to sense any alteration in the oxygen content of the blood coming from the placenta via the umbilical vein. This enables the foetus to produce a cardiovascular response to hypoxia when necessary .
Foetal oxygenation in labour
Labour increases the risk of compromised oxygenation in the foetus. Uterine contractions produce transient decreases in the blood flow to the placenta, which can lead to interruptions in gas exchange across the placental barrier. Certain degrees of hypoxaemia and acidaemia are normal in healthy foetuses during a normal labour, and so one of the challenges is to successfully identify foetuses experiencing levels of hypoxaemia and acidaemia that are sufficient to cause physiological damage. Table 1 lists acid–base values in the healthy, term foetus before and healthy neonates after birth, along with the adult values for comparison.
Umbilical vein before birth a | Umbilical cord artery after birth | Umbilical cord vein after birth | Adult artery d | |
---|---|---|---|---|
pH | >7.38 | 7.27 (7.09–7.38) b | 7.35 (7.21–7.46) b | 7.36–7.44 |
p CO 2 (kPa) | <5.6 | 7.1 (5.1–10.0) b | 5.2 (3.7–7.1) b | 4.7–5.9 |
BDecf (mmol/l) | <3 | 2.2 (−1.8–8.1) b | 3.5 (−0.4–8.7) b | −3.0–3.0 |
p O 2 (kPa) | >2.93 | 2.1 (0.7–4.1) c | 3.5 (1.7–4.9) c | 10–14 |
a Results obtained by cordocentesis .
b Median (2.5th–97.5th percentiles). Data from 13,181 term neonates, spontaneous vaginal deliveries, no epidural analgesia, no induction and 5-min Apgar score of ≥7 .
c Data from 1145 term neonates with a 5-min Apgar score of ≥7 after spontaneous vaginal deliveries .
d 95% reference interval, from Laboratory Book, Department of Medical Biochemistry, Oslo University Hospital, Rikshospitalet.
However, if the oxygen deficit is prolonged, foetal metabolism switches to anaerobic. This leads to a decrease in pH, accumulation of lactate and an increase in the base deficit (BD). The concentrations of free hydrogen ions (H + ; [H + ]) are expressed as pH values, and they are calculated as the negative logarithm of [H + ]; thus, pH decreases as [H + ] increases.
According to the international consensus, metabolic acidosis in the umbilical cord arterial blood at birth is defined as umbilical cord pH <7.00 and BD ≥12.0 mmol/l . The aim of calculating BD is to have a measure of the metabolic component of an acidosis. This measure should be expressed in such a way that it is independent of the respiratory component. An acute increase in the partial pressure of carbon dioxide (CO 2 ; p CO 2 ) in vivo causes an increase in the BD in the blood (BDblood) and a reduction in the BD in the plasma (BDplasma), whereas the BD in the extracellular fluid (BDecf) remains constant. The cause of these changes is a redistribution of H + within the extracellular volume and erythrocytes. H + diffuses from the poorly buffered interstitial fluid into the blood plasma, and then into the erythrocytes. Very little H + transfer occurs between the intracellular and extracellular spaces, and so BDecf remains virtually constant during acute changes in p CO 2 in vivo . In other words, changes in p CO 2 affect BDblood and BDplasma, but not BDecf . It has been reported that BDecf is typically 1–2 mmol/l lower than BDblood .
Foetal oxygenation in labour
Labour increases the risk of compromised oxygenation in the foetus. Uterine contractions produce transient decreases in the blood flow to the placenta, which can lead to interruptions in gas exchange across the placental barrier. Certain degrees of hypoxaemia and acidaemia are normal in healthy foetuses during a normal labour, and so one of the challenges is to successfully identify foetuses experiencing levels of hypoxaemia and acidaemia that are sufficient to cause physiological damage. Table 1 lists acid–base values in the healthy, term foetus before and healthy neonates after birth, along with the adult values for comparison.
Umbilical vein before birth a | Umbilical cord artery after birth | Umbilical cord vein after birth | Adult artery d | |
---|---|---|---|---|
pH | >7.38 | 7.27 (7.09–7.38) b | 7.35 (7.21–7.46) b | 7.36–7.44 |
p CO 2 (kPa) | <5.6 | 7.1 (5.1–10.0) b | 5.2 (3.7–7.1) b | 4.7–5.9 |
BDecf (mmol/l) | <3 | 2.2 (−1.8–8.1) b | 3.5 (−0.4–8.7) b | −3.0–3.0 |
p O 2 (kPa) | >2.93 | 2.1 (0.7–4.1) c | 3.5 (1.7–4.9) c | 10–14 |
a Results obtained by cordocentesis .
b Median (2.5th–97.5th percentiles). Data from 13,181 term neonates, spontaneous vaginal deliveries, no epidural analgesia, no induction and 5-min Apgar score of ≥7 .
c Data from 1145 term neonates with a 5-min Apgar score of ≥7 after spontaneous vaginal deliveries .
d 95% reference interval, from Laboratory Book, Department of Medical Biochemistry, Oslo University Hospital, Rikshospitalet.
However, if the oxygen deficit is prolonged, foetal metabolism switches to anaerobic. This leads to a decrease in pH, accumulation of lactate and an increase in the base deficit (BD). The concentrations of free hydrogen ions (H + ; [H + ]) are expressed as pH values, and they are calculated as the negative logarithm of [H + ]; thus, pH decreases as [H + ] increases.
According to the international consensus, metabolic acidosis in the umbilical cord arterial blood at birth is defined as umbilical cord pH <7.00 and BD ≥12.0 mmol/l . The aim of calculating BD is to have a measure of the metabolic component of an acidosis. This measure should be expressed in such a way that it is independent of the respiratory component. An acute increase in the partial pressure of carbon dioxide (CO 2 ; p CO 2 ) in vivo causes an increase in the BD in the blood (BDblood) and a reduction in the BD in the plasma (BDplasma), whereas the BD in the extracellular fluid (BDecf) remains constant. The cause of these changes is a redistribution of H + within the extracellular volume and erythrocytes. H + diffuses from the poorly buffered interstitial fluid into the blood plasma, and then into the erythrocytes. Very little H + transfer occurs between the intracellular and extracellular spaces, and so BDecf remains virtually constant during acute changes in p CO 2 in vivo . In other words, changes in p CO 2 affect BDblood and BDplasma, but not BDecf . It has been reported that BDecf is typically 1–2 mmol/l lower than BDblood .
Foetal energy supply and metabolism
In the foetus, energy is produced mainly by glycolysis, ending with the formation of adenosine triphosphate (ATP) and pyruvate. In this pathway, glucose is first converted into pyruvate, and two ATP molecules are generated.
Under aerobic conditions, pyruvate is usually converted to acetyl coenzyme A, and in the presence of oxaloacetate, it enters the citric acid cycle. Further on, it is subjected to mitochondrial oxidation to CO 2 as a waste product from these reactions, and to water. The CO 2 produced by the cells diffuses across the cell membrane into the surrounding blood, and it enters the erythrocytes where it is rapidly converted by the enzyme carbonic anhydrase into carbonic acid (H 2 CO 3 ) and bicarbonate ion (HCO 3 − ). Thus, the excess CO 2 is removed by the bicarbonate buffering system, and this prevents large increases in acidity that H + would otherwise cause:
This reaction occurs bidirectionally until the blood reaches the placenta, where the CO 2 is eliminated .
As in adults, buffers in the foetus play a central role in neutralising H + production and in maintaining constant blood and tissue pH values. Adequate buffering prevents significant changes in the foetal pH. The bicarbonate buffer, which is the main buffer system in the plasma, accounts for 35% of the foetal buffering capacity in the blood . Bicarbonate and the fixed acids cross the placenta much more slowly than does CO 2 ; their equilibration takes hours rather than minutes. The rate at which CO 2 crosses the placenta is limited by the blood flow, and not the resistance to diffusion .
In the steady state, the total cellular CO 2 production is equal to the transplacental CO 2 elimination, and thus no changes occur in foetal pH. However, the margin of safety for the foetal elimination of CO 2 is small. The only way the foetus can increase the elimination somewhat is by increasing the foetal heart rate (FHR), which will in turn increase placental perfusion . Another major buffer is haemoglobin (Hb) , which accounts for approximately 35% of the foetus’s total buffering capacity in the blood :
Inorganic phosphates and erythrocyte bicarbonate are also potential buffers, although they play a lesser role in foetal acid–base haemostasis .
When a tissue is exposed to a reduced oxygen supply, it loses its capacity for efficient oxidative phosphorylation, and this results in the cells switching from aerobic to anaerobic metabolism. Under anaerobic conditions, pyruvate is reduced to lactate, via lactate dehydrogenase in the presence of nicotinamide adenine dinucleotide (NADH). This leads to an energy-inefficient state with the depletion of ATP reserves, the accumulations of lactic acid and H + , and the impairment of cellular functioning . This process is presented schematically in Fig. 1 .
The end product of the citric acid cycle is 36 ATP molecules and NADH, which is formed by combining NAD + and H + . Under aerobic conditions, the reaction reverses, and lactate is oxidised back to pyruvate. Lactate can then be recreated to glucose and glycogen through gluconeogenesis, but the main fate of pyruvate is to enter the citric acid cycle. In the metabolic steady state, the relationship between pyruvate and lactate reflects the intracellular redox status. The ratio of lactate to pyruvate is normally 10–16:1 . Under anaerobic conditions, the accumulated NADH promotes NAD + regeneration by increasing the pyruvate reduction to lactate. Anaerobic metabolism leads to the accumulation of lactate and subsequently a decrease in pH. Therefore, an increased lactate:pyruvate ratio is a sign of hypoxia.
The importance of anaerobic metabolism in the maintenance of myocardial function, and survival of the foetus, depends on the prehypoxic glycogen content in the myocardium . Glycogen stores are consumed rapidly during anaerobic metabolism, since one molecule of glucose provides only two ATP molecules, compared with aerobic metabolism producing 36 ATP molecules.
Development of asphyxia
Foetal asphyxia almost always occurs as a result of a gradual insufficiency in the umbilical blood flow or insufficient uterine blood flow, and in most cases, it is attributable to a reduction in gas exchange for variable time periods. However, a sudden complete cessation of oxygen delivery to the foetus as a cause of asphyxia is clinically rare, but often catastrophic.
Occlusion of one or more of the vessels in the umbilical cord can impede circulation to and from the foetus. During these events, the oxygen content of the foetal blood may decrease and the CO 2 or H 2 CO 3 content may increase (producing hypercapnia). The excess CO 2 is removed by the HCO 3 − . Respiratory acidosis is defined as a decreased blood pH with an elevated blood p CO 2. Further decreases in the oxygen content of the foetal blood, if repetitive and/or prolonged, can lead to hypoxia, which will in turn lead to an increase in lactate in the blood. The accumulation of lactic acid can deplete the buffer system, cause failure of the ATP-dependent sodium–potassium pump and disrupt the exchange of ions across the cell membrane, initiating a cascade of reactions that lead to cell injury and death.
Maternal–foetal gas exchange
The oxygen tension (or partial pressure of oxygen, p O 2 ) and drive for effective foetal gas exchange in utero is quite different from that in the adult population. Sir Joseph Barcroft summarised this in 1946 with the phrase ‘Mount Everest in utero’, comparing the p O 2 in the foetus with what we found in humans at the top of Mount Everest . However, this does not mean that the foetus spends months with inadequate oxygenation. The foetal physiology is characterised by several adaptive mechanisms that facilitate the transfer of gases between the maternal and foetal circulation, and that support function and growth in a low-oxygen environment. The diffusion of oxygen is dependent on the p O 2 , the concentration of Hb, the type of Hb, oxygen saturation and blood flow. The progesterone-induced hyperventilation that occurs during pregnancy reduces the maternal p CO 2, and it maintains a negative gradient over the placental membrane, which facilitates the transfer of CO 2 from the foetus to the mother . p O 2 is higher in the maternal circulation than in the foetal circulation, which facilitates maternal-to-foetal transfer of oxygen via diffusion across the placental membranes ( Table 1 ). The amount of oxygen transported depends on (1) the driving force (i.e., the diffusion gradient from mother to foetus), (2) the blood flow on both sides of the placental membranes and (3) the affinity for oxygen in adult Hb (HbA) and foetal Hb (HbF).
HbA is chemically different from HbF. HbF is a structure of the Hb tetramer molecule, which consists of γ-chains instead of the adult β-chains, in addition to the α-chains included in both molecules . The oxygen–Hb dissociation curve for HbF is shifted to the left compared with that for HbA, as HbF has a greater affinity for oxygen at the same p O 2 . This permits the saturation of foetal blood with oxygen at a lower p O 2 ( Fig. 2 ) . Thus, an oxygen saturation of 50% corresponds to 3.6 kPa for foetal blood and 4.8 kPa for adult blood . The oxygen–Hb dissociation curve is dependent on pH and pCO 2 , as described by the Bohr effect , whereby a decrease in pH and an increase in p CO 2 each cause the curve to shift to the right. This Bohr effect facilitates the dissociation of oxygen in tissues where the pH is relatively low and the p CO 2 is high. HbF is gradually replaced by HbA with advancing gestational age, so that at near term, about 20% of the Hb is HbA . As Hb is a buffer and HbF and HbA have different oxygen-carrying and oxygen-delivering properties, the change in Hb type with advancing gestational age may alter both the foetal buffering capacity and the oxygen–Hb dissociation curve. Finally, the concentration of Hb is 40% higher in the foetus than in the adult.

Full access? Get Clinical Tree
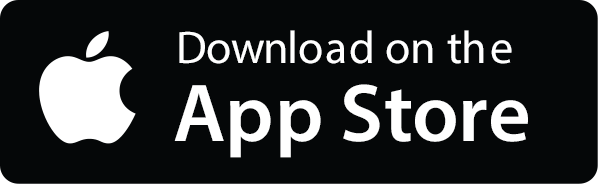
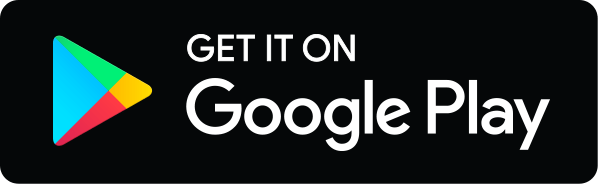