Pathologic Causes of Jaundice
Indirect-reacting Hyperbilirubinemia
Table 35-15 lists the causes of pathologic indirect-reacting hyperbilirubinemia in the neonate and Table 35-16 lists the causes of direct-reacting hyperbilirubinemia (cholestatic jaundice). For further discussion of neonatal cholestasis, please see Chapter 40.
TABLE 35-15 CAUSES OF INDIRECT HYPERBILIRUBINEMIA IN NEWBORNS | |||||||
---|---|---|---|---|---|---|---|
|
Increased Bilirubin Load: Hemolytic Disease
Immune-Mediated Hemolytic Disease
Rh Erythroblastosis Fetalis.
Pathogenesis. Hemolytic causes of hyperbilirubinemia are discussed fully in Chapter 46. Most cases of Rh immunization are a result of the Rh (D) antigen although alloimmunization can occur to other fetal red cell surface antigens including the other antigens of the Rh blood-group system (c, C, e, E, cc, and Ce) and those belonging to the Kell, Duffy, Kidd, and MNS systems (294,295,296). Rh alloimmunization occurs when fetal red blood cells from an Rh (D)-positive fetus cross the placenta into the circulation of an Rh-negative mother and less than 0.1 mL of fetal red cells is sufficient to result in sensitization. Although Kleihauer-Betke measurements indicate that fetal-maternal hemorrhage occurs in 75% of women, more sensitive molecular detection techniques show that fetal red cells enter the maternal circulation in all pregnancies (296). If the Rh-positive fetus is ABO
compatible with its mother, the likelihood of Rh immunization is 16%, but it is only 1.5% to 2% if they are ABO incompatible. This is because the fetal ABO-incompatible red blood cells are rapidly destroyed in the maternal circulation, diminishing the opportunity of the Rh antigen to induce an immune response (294). Once a primary immune response to Rh antigen has been mounted, however, ABO incompatibility between the mother and fetus conveys no protection against a secondary immune response (294). The risk of alloimmunization following induced abortion is 4% to 5%, and it is 2% following spontaneous abortion. Other invasive procedures, such as amniocentesis, chorionic villus sampling, and fetal blood sampling, all increase the risk of fetal maternal hemorrhage and, therefore, alloimmunization (296).
compatible with its mother, the likelihood of Rh immunization is 16%, but it is only 1.5% to 2% if they are ABO incompatible. This is because the fetal ABO-incompatible red blood cells are rapidly destroyed in the maternal circulation, diminishing the opportunity of the Rh antigen to induce an immune response (294). Once a primary immune response to Rh antigen has been mounted, however, ABO incompatibility between the mother and fetus conveys no protection against a secondary immune response (294). The risk of alloimmunization following induced abortion is 4% to 5%, and it is 2% following spontaneous abortion. Other invasive procedures, such as amniocentesis, chorionic villus sampling, and fetal blood sampling, all increase the risk of fetal maternal hemorrhage and, therefore, alloimmunization (296).
TABLE 35-16 CAUSES OF NEONATAL CHOLESTASIS | ||
---|---|---|
|
The initial response to the foreign antigen in the maternal circulation is for the maternal immune system to produce immunoglobulin (Ig) M antibodies that do not cross the placenta. This response is followed by production of IgG antibodies, which then cross the placental barrier. The secondary immune response to repeat exposure to the Rh antigen produces anti-D IgG antibodies. This response can be induced with as little as 0.03 ml of D-positive red blood cells (297). The degree of Rh sensitization is related to the dose of antigen exposure and, therefore, to the volume of transplacental hemorrhage (294).
Clinical Course.
Approximately 50% of affected infants do not require treatment; they are mildly anemic at birth and never develop severe hyperbilirubinemia. Approximately 25% to 30% will require intervention with phototherapy and/or exchange transfusion and approximately 20% to 25% are so severely affected that they develop hydrops in utero (296). About half of this last group become hydropic before 34 weeks’ gestation and require direct intravascular fetal transfusion (294). A fetal hematocrit of less than 30% is generally considered an indication for intrauterine transfusion, which is performed, as required, until 34 to 35 weeks’ gestation with delivery planned close to term (296).
Prevention of Rh Sensitization.
Rh sensitization can almost always be prevented by the administration of Rh immunoglobulin to Rh-negative women at 28 weeks of gestation and again within 72 hours of delivery of an Rh-positive infant (295). In the United States, the dose is 300 μg, but in many other countries it is 100 to 125 μg. If the Kleihauer-Betke test or the fetal red cell assay indicates that there is a transplacental hemorrhage of more than 30 mL of fetal blood (which occurs in 1 in 400 pregnancies) then the dose of Rh (D) immunoglobulin must be at least 10 μg/mL of fetal blood in the maternal circulation (296). Rh immunoglobulin must also be given after abortion or threatened abortion and after amniocentesis or chorionic villus sampling or any other invasive intrauterine procedure. These interventions have dramatically reduced the incidence of erythroblastosis fetalis caused by Rh (D) sensitization, which now has an estimated incidence of about 1 per 1,000 live births (298).
Some laboratories have replaced the Kleihauer-Betke assay with a fetal red blood cell assay (Fetalscreen, Ortho-Clinical Diagnostics, Raritan, NJ) (299). In this assay, an antihemoglobin F antibody is added to the mother’s blood to tag hemoglobin F molecules in fetal red cells. Flow cytometry quantifies the number of fetal red cells (of a total of 50,000 maternal cells) so tagged. If less than 0.1% of the maternal cells are tagged it is considered a negative result. Positive results can be quantified to provide the volume of fetal blood in the mother’s circulation and the appropriate dose of Rh (D) immunoglobulin to be given.
In mothers who are already sensitized, the administration of intravenous immunoglobulin (IVIg) in early pregnancy has had some benefit in cases of severe fetal alloimmunization (300). The mechanism of action of IVIg appears to involve blockage of Fc receptors on macrophages in the fetal reticuloendothelial system. In one study, fetal survival was 36% higher when high-dose IVIg treatment preceded intrauterine transfusion than it was with transfusion alone (300).
Hydrops Fetalis.
The pathogenesis of hydrops fetalis, with its attendant edema and serous effusions, is not clear. It commonly occurs when the fetal hemoglobin drops below 6 to 7 g/dL. The rapid production of severe anemia in fetal sheep produced hydrops associated with an increased central venous pressure and placental edema, whereas the same degree of anemia produced over a longer period did not result in hydrops, placental edema, or an increased central venous pressure (301). In Rh isoimmunization, fetal edema may result from the extensive erythropoiesis that takes place in the fetal liver. This can disrupt the portal circulation and impair albumin synthesis (302,303). Fetuses with severe hydrops also have elevated concentrations of atrial natriuretic factor (304). Hypoxia produces myocardial dysfunction with increased umbilical venous pressure that leads to the release of atrial natriuretic factor (305). Severely affected infants die of progressive cardiorespiratory failure, in which asphyxia and hyaline membrane disease play a major role.
In one hydropic fetus with erythroblastosis fetalis, pulse-Doppler studies of left and right ventricular outputs were obtained over time. Despite severe anemia, cardiac outputs were normal and remained normal after in utero percutaneous intravascular transfusions, which reversed the hydrops. These measurements of normal cardiac output in utero suggest that high-output failure caused by anemia is not the mechanism for hydrops in these infants and supports the hypothesis that portal hypertension and disruption of normal liver function from extramedullary hematopoiesis is the primary mechanism for the development of hydrops in isoimmune hemolytic disease of the fetus (306).
Treatment of Infants with Rh Hemolytic Disease and Hydrops Fetalis.
Late Anemia.
A well-known late complication of intrauterine transfusion is the development of anemia in
the first months of life. This is commonly seen after about age 2 weeks and is characterized by a persistently low reticulocyte count. Although originally considered to be a hyporegenerative anemia (294,307), there is now some evidence that a decrease in erythropoietin production may not be the primary cause of this late anemia. Recent studies suggest that the anemia is most likely caused by persistence of anti-D antibodies and destruction of red blood cell precursors in the marrow or of reticulocytes in the peripheral circulation (308). In a study of 30 neonates with erythroblastosis, 18 of whom had received intrauterine transfusions and 12 who had not (308), blood samples were analyzed for hemoglobin, erythropoietin, and reticulocytes. As the hemoglobin declined between 10 and 80 postnatal days, there was a matching rise in erythropoietin, which occurred both in infants who had received intrauterine transfusions and those who had not. Thus, intrauterine transfusions, themselves, may not play such an important role in suppressing erythropoiesis. Furthermore, reticulocyte counts remained persistently low despite rising erythropoietin and falling hemoglobin levels. One possible explanation for this is the effect of circulating anti-D antibodies on the erythrocyte precursors in the bone marrow and on the reticulocytes in the peripheral circulation (308).
the first months of life. This is commonly seen after about age 2 weeks and is characterized by a persistently low reticulocyte count. Although originally considered to be a hyporegenerative anemia (294,307), there is now some evidence that a decrease in erythropoietin production may not be the primary cause of this late anemia. Recent studies suggest that the anemia is most likely caused by persistence of anti-D antibodies and destruction of red blood cell precursors in the marrow or of reticulocytes in the peripheral circulation (308). In a study of 30 neonates with erythroblastosis, 18 of whom had received intrauterine transfusions and 12 who had not (308), blood samples were analyzed for hemoglobin, erythropoietin, and reticulocytes. As the hemoglobin declined between 10 and 80 postnatal days, there was a matching rise in erythropoietin, which occurred both in infants who had received intrauterine transfusions and those who had not. Thus, intrauterine transfusions, themselves, may not play such an important role in suppressing erythropoiesis. Furthermore, reticulocyte counts remained persistently low despite rising erythropoietin and falling hemoglobin levels. One possible explanation for this is the effect of circulating anti-D antibodies on the erythrocyte precursors in the bone marrow and on the reticulocytes in the peripheral circulation (308).
ABO Hemolytic Disease
Approximately 45% of Americans of western European descent have type O blood, and a similar percentage are type A. Types B and AB make up the balance. African Americans are 50% type O, 29% type A, 17% type B, and 4% AB (309). Hemolytic disease related to ABO incompatibility is generally limited to group A or B infants born to group O mothers, and it tends to run in families. One study found an 88% risk of recurrence of ABO hemolytic disease in ABO incompatible newborns born to parents whose first-born child was similarly affected (310).
In a prospective study of 4,996 consecutive live born infants (311) (Table 35-17), cord blood was analyzed for blood type, hematocrit, and results of the direct antiglobulin test (DAT or Coombs test) and the indirect Coombs test (311). The DAT detects antibodies attached to the red cell, whereas the indirect Coombs test detects IgG antibody in the serum. Only 0.29% of type A, B, or AB infants who were incompatible with their type A or B mothers had a positive DAT result, whereas 32% of type A or B infants born to type O mothers had positive DATs. A positive DAT was the best predictor of an elevated bilirubin level, but only 20% of infants with a positive DAT developed TSB levels of ≥12.8 mg/dL (224 μmol/L) (311). This large prospective study confirms what was found in other smaller studies: although about one-third of group A or B infants born to group O mothers have anti-A or anti-B antibodies attached to their red cells, only 1 in 5 of those with a positive DAT have a modest degree of hyperbilirubinemia.
TABLE 35-17 BILIRUBIN LEVELS IN ABO-INCOMPATIBLE INFANTS ACCORDING TO THE COOMBS TEST | ||||||||||
---|---|---|---|---|---|---|---|---|---|---|
|
Consequently, although ABO-incompatible DAT-positive infants are about twice as likely as their compatible peers to have moderate hyperbilirubinemia, severe jaundice in these infants is uncommon (311,312,313,314,315) and ABO hemolytic disease is a relatively rare cause of severe hyperbilirubinemia (Table 35-18).
Diagnosing ABO Hemolytic Disease.
There is a wide spectrum of severity in ABO hemolytic disease. Nevertheless, this diagnosis generally should not be made unless there is a positive DAT and clinical jaundice within the first 12 to 24 hours. Reticulocytosis and the presence of microspherocytes on the smear help to confirm the diagnosis (Table 35-19).
ABO Incompatibility, Hyperbilirubinemia, and a Negative Direct Antiglobulin Test.
Although the epidemiologic data suggest otherwise, most clinicians have seen the occasional ABO-incompatible infant in whom there is an early rising TSB level, yet the DAT is negative. In the past, we have often considered these to be cases of ABO hemolytic disease and have attributed the negative DAT to the technical vicissitudes of Coombs testing in the laboratory. Recent data, however, raise questions about alternative mechanisms.
One obvious possibility is that there is another cause for the hemolysis. Using measurements of end-tidal carbon
monoxide concentration (ETCOc), a direct measurement of heme catabolism, Herschel and associates (316) identified four DAT-negative ABO-incompatible neonates who had elevated ETCOc levels. Further investigation revealed that two of these infants had G6PD deficiency and one had elliptocytosis. Can ABO incompatibility with a negative DAT nevertheless contribute to hyperbilirubinemia? Kaplan and associates (317) found that 43% of DAT-negative, ABO-incompatible infants who were homozygous for the variant UGT promoter associated with Gilbert syndrome, had a TSB level ≥15 mg/dL (256 μmol/L) versus none of the ABO-incompatible DAT-negative infants who were homozygous normal (for the variant promoter) (Fig. 35-17). There was no difference between ABO-incompatible and ABO-compatible DAT-negative newborns, as long as the ABO-incompatible neonates did not have Gilbert syndrome (see Inherited Unconjugated Hyperbilirubinemia). These observations confirm, for the first time, that if another icterogenic factor is present, then ABO-incompatible newborns are at risk for hyperbilirubinemia even if they are DAT negative (317).
monoxide concentration (ETCOc), a direct measurement of heme catabolism, Herschel and associates (316) identified four DAT-negative ABO-incompatible neonates who had elevated ETCOc levels. Further investigation revealed that two of these infants had G6PD deficiency and one had elliptocytosis. Can ABO incompatibility with a negative DAT nevertheless contribute to hyperbilirubinemia? Kaplan and associates (317) found that 43% of DAT-negative, ABO-incompatible infants who were homozygous for the variant UGT promoter associated with Gilbert syndrome, had a TSB level ≥15 mg/dL (256 μmol/L) versus none of the ABO-incompatible DAT-negative infants who were homozygous normal (for the variant promoter) (Fig. 35-17). There was no difference between ABO-incompatible and ABO-compatible DAT-negative newborns, as long as the ABO-incompatible neonates did not have Gilbert syndrome (see Inherited Unconjugated Hyperbilirubinemia). These observations confirm, for the first time, that if another icterogenic factor is present, then ABO-incompatible newborns are at risk for hyperbilirubinemia even if they are DAT negative (317).
TABLE 35-18 DISCHARGE DIAGNOSIS IN 306 INFANTS ADMITTED WITH SEVERE HYPERBILIRUBINEMIAa | ||||||||||||||||||||||||
---|---|---|---|---|---|---|---|---|---|---|---|---|---|---|---|---|---|---|---|---|---|---|---|---|
|
TABLE 35-19 CRITERIA FOR DIAGNOSING ABO HEMOLYTIC DISEASE AS THE CAUSE OF NEONATAL HYPERBILIRUBINEMIA | |
---|---|
|
Should a blood type and DAT be performed on the cord blood of all infants of group O mothers?
In these days of cost containment, this is a commonly asked question. A recent survey found that 58% of hospital blood banks in the United States were routinely performing Coombs tests and blood typing on newborn cord bloods (318). Approximately 36% of hospitals tested all cord bloods routinely, and 35% tested those of type O or Rh-negative mothers, even though the data suggest that such routine screening is not warranted (311,318). Furthermore, even when such testing is done, there is evidence that it is often ignored by the responsible pediatrician (318,319). The AAP notes that routine cord blood screening for infants of group O, Rh-positive mothers, is an option, but is not required “provided there is appropriate surveillance, and risk assessment before discharge and follow up” (76) so that significantly jaundiced infants are not missed.
Heritable Causes of Hemolysis
Red Cell Membrane Defects
The red cell membrane defects that may produce hemolysis and hyperbilirubinemia in the newborn include hereditary spherocytosis, elliptocytosis, pyropoikilocytosis, pyknocytosis and stomatocytosis syndromes (320,321). Detailed descriptions of their clinical presentation and management can be found elsewhere (320,321,322). The diagnosis of these disorders can be difficult, because newborns commonly exhibit a marked variation in red cell membrane size and shape (322).
In 75% of hereditary spherocytosis patients, inheritance is autosomal dominant so that a positive family history that includes anemia, jaundice, gallstones, and splenectomy can often be elicited. As in G6PD deficiency (see Glucose-6-Phosphate Dehydrogenase Deficiency below), the presence of jaundice severe enough to require phototherapy in newborns with hereditary spherocytosis is strongly related to an interaction with the Gilbert syndrome allele (323). Severe anemia and hydrops fetalis has occurred
in infants with hereditary spherocytosis associated with defective genes for band 3 or spectrin protein (321). The diagnosis can be made using the incubated osmotic fragility test which is a reliable diagnostic tool in newborns when coupled with fetal red cell controls (321,322).
in infants with hereditary spherocytosis associated with defective genes for band 3 or spectrin protein (321). The diagnosis can be made using the incubated osmotic fragility test which is a reliable diagnostic tool in newborns when coupled with fetal red cell controls (321,322).
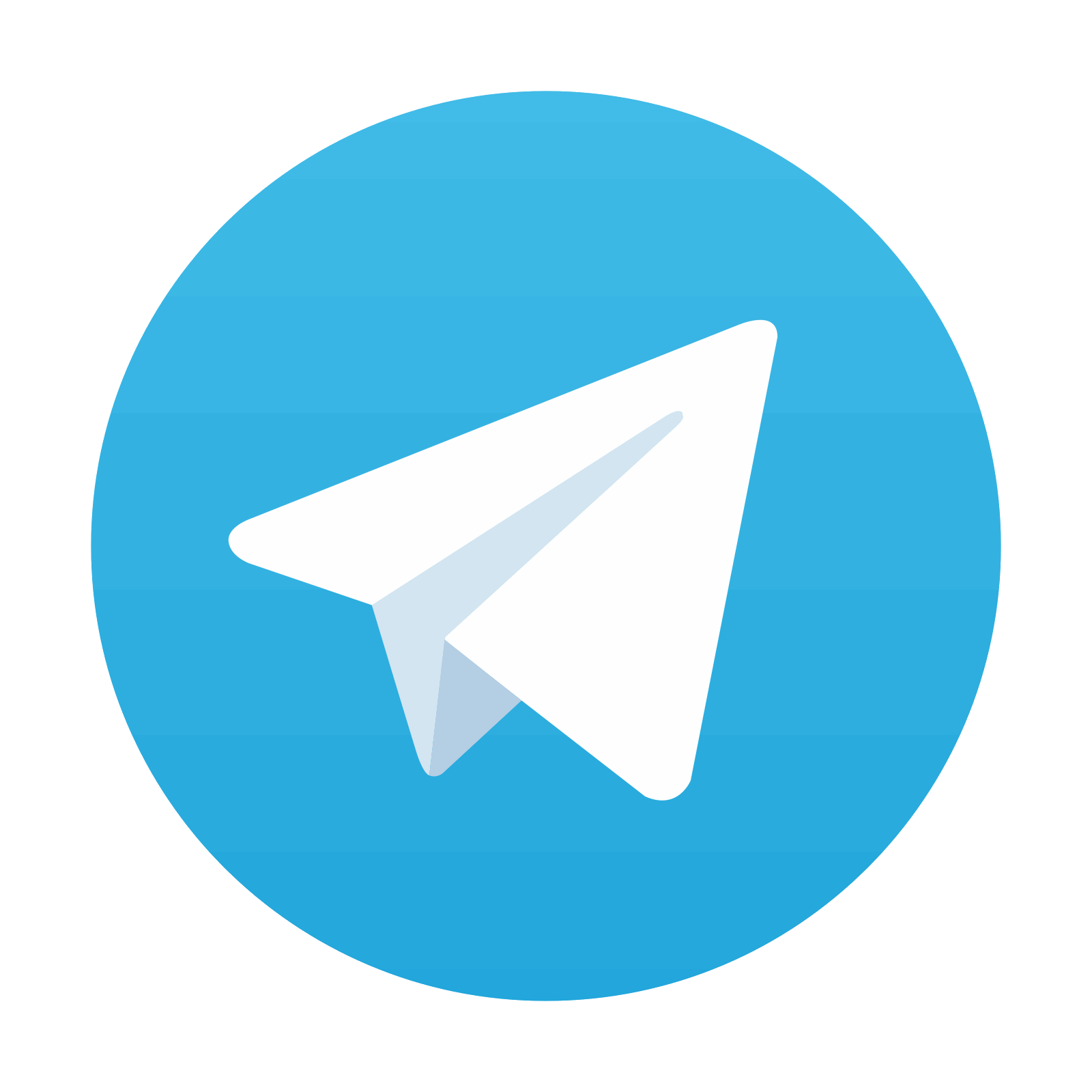
Stay updated, free articles. Join our Telegram channel

Full access? Get Clinical Tree
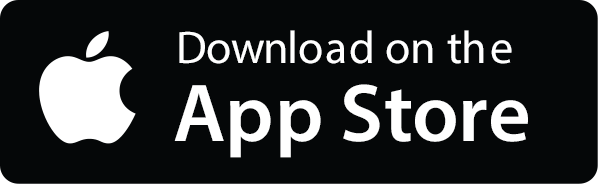
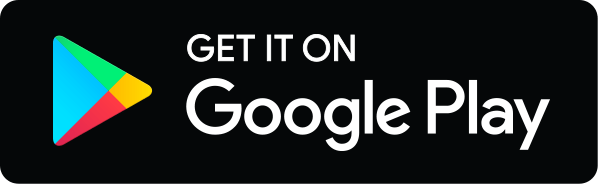