Objective
This study was undertaken to identify the paraoxonase 1 gene and glutathione S-transferase μ 1 gene interaction for the risk of preterm delivery and to determine the serum paraoxonase activity according to paraoxonase 1 genotypes.
Study Design
This case-control study was performed on 162 gravida women with preterm delivery and 306 controls. Serum paraoxonase activity was measured by a ultraviolet spectrophotometer. Logistic regression, 2-way analysis of variance, and multifactor dimensionality reduction analysis were used.
Results
Gravida women with the QQ and QR genotype of paraoxonase 1 with high body mass index had 6.19- and 4.41-fold increased risks of preterm delivery. The glutathione S-transferase μ 1 null genotype and the interaction between the paraoxonase 1 genotype and glutathione S-transferase μ 1 null type conferred a risk for preterm delivery. Serum paraoxonase activity was significantly different according to paraoxonase 1 genotypes ( P < .0001).
Conclusion
The glutathione S-transferase μ 1 null genotype confers a risk for preterm delivery in Korean gravida women independent of and interactive with the paraoxonase 1 genotype.
Preterm delivery (<37 weeks’ gestation) accounts for 10% of deliveries in Korea; the rate has been increasing in the last decade. Preterm delivery is associated with perinatal death, and neonatal morbidity and mortality. However, the cause of preterm delivery has not been precisely determined. It has become well-established that preterm delivery has a genetic component through a family history of preterm delivery and twin studies.
Among the associations between preterm delivery and genetic factors, oxidative stress-related genes involved in metabolic detoxification processes have been reported to be associated with preterm delivery. In particular, glutathione S-transferase μ 1(GSTM1) and θ 1 (GSTT1), which are major phase II enzymes, are good candidates for preterm delivery susceptibility genes. Our group has shown that the interaction between cytochrome p450 IA1(CYP1A1)-1462V and the GSTM1 null genotype increases the risk for preterm delivery in Korean gravida women.
Human serum paraoxonase 1 (PON1) is a high-density lipoprotein (HDL)-bound enzyme that is considered the major determinant of the antioxidant action of HDL and can modulate the level of oxidative stress. PON1 exerts paraoxonase and arylesterase activities because the enzyme hydrolyzes organophosphates and aromatic esters. PON activity varies widely among individuals related to PON1 gene polymorphism, and this activity is decreased in early pregnancy failure and preeclampsia. Human PON1 has 2 genetic polymorphisms, 1 at position 55, which is a methionine (M)/leucine (L) substitution, and one at position 192, which is a glutamine (Q)/arginine (A) substitution. In regard to the association with preterm delivery, the infant PON1-192R allele has been associated with preterm delivery, and in a large study of British gravida women, maternal PON1 RR genotype was reported to increase preterm risk.
In the current study, we hypothesized that maternal polymorphisms of GSTM1, GSTT1, and PON1 are likely to be associated with the risk of preterm delivery, and considered that gene-gene interactions (epistasis) probably affect this risk. The objective of this study was to identify the PON1 gene and GSTM1 gene interaction that increases the risk of preterm delivery and also to investigate the serum PON activity in gravida women according to PON1 genotypes.
Materials and Methods
Population and data collection
This study involved a case-control study conducted at Ewha Womans University Hospital in Seoul, Korea from July 2006 to December 2008. The study was approved by the Institutional Review Board of Ewha Womans University Hospital. We recruited cases that delivered preterm births (<37 weeks of gestation) from the patients who had delivered at the hospital. Normal controls who underwent a term delivery (≥37 weeks of gestation) were selected among women who had undergone prenatal examinations in the hospital and were followed until infant delivery according to the ratio of cases to controls as being approximately 2. Control subjects were not matched to cases on any specific factors and were identified over the same period as cases, and generally identified on the same day as an individual case. Inclusion criteria were a singleton birth and gestational age between 25 and 42 weeks. We excluded women with multiple births, stillbirths, congenital anomalies, chronic hypertension, placenta previa, and abruption placenta.
Trained interviewers collected the general information on epidemiologic and clinical data from participants, who signed the consent forms before enrollment. The weight, height, and blood samples of each participant were obtained according to standard protocols. We determined gestational age in accordance with the onset of the last menstrual period or the first ultrasonographic estimation in the event that the last menstrual period was unreliable. Finally, 162 gravida women with preterm births and 306 normal controls with term deliveries were enrolled in the current study.
Genotyping analysis
We extracted genomic DNA from whole blood using a QIAmp blood kit (Qiagen, Hilden, Germany). The GSTM1 and GSTT1 deletion polymorphisms were detected by a polymerase chain reaction (PCR) reaction, which was performed in a total volume of 10 μL, containing 10 ng of genomic DNA, 0.5 uM each of the sense and antisense primers, 0.5 mM each of the 4 deoxynucleotide phosphates (dATP, dTTP, dCTP, and dGTP), and 0.05 U of Taq polymerase (I-star Taq; iNtRON Biotechnology, Gyeonggi-Do, Korea). The primers for GSTM1 and GSTT1 were used to amplify 219 and 480 base pair (bp) fragments, respectively. We considered the absence of the 219/480 bp fragment in both alleles as the GSTM1/GSTT1 null genotype and the presence of the 219/480 bp fragment in 1 or both alleles as the GSTM1/GSTT1 positive genotype.
PON1 polymorphism was analyzed according to Humbert et al. The genotyping of the PON1 polymorphsim (rs662) was screened with a single base primer extension assay using an ABI PRISM SNaPShot Multiplex kit (ABI, Foster City, CA) according to the manufacturer’s recommendation. Briefly, the genomic DNA flanking the interested SNP was amplified using a PCR reaction with forward and reverse primer pairs and standard PCR reagents in a 10 μL reaction volume containing 10 ng of genomic DNA, 0.5 pM of each oligonucleotide primer, 1 μL 10X PCR buffer, 250 μM dNTP (2.5 mM each), and 0.25 units of i-StarTaq DNA polymerase (5 units/μL; iNtRON Biotechnology). The PCR reactions were carried out as follows: 10 minutes at 95°C for 1 cycle, and 35 cycles at 95°C for 30 seconds, 60°C for 1 minutes, 72°C for 1 minutes, and followed by 1 cycle at 72°C for 10 minutes. After amplification, the PCR products were treated with 1 unit each of shrimp alkaline phosphatase (SAP; Roche, Basle, Switzerland) and exonuclease I (USB Corporation, Cleveland, OH) at 37°C for 75 minutes and 72°C for 15 minutes to purify the amplified products. One microliter of the purified amplification products was added to a SNaPshot Multiplex Ready reaction mixture containing 0.15 pmol of genotyping primer for the primer extension reaction. The primer extension reaction was carried out for 25 cycles at 96°C for 10 seconds, 50°C for 5 seconds, and 60°C for 30 seconds. The reaction products were treated with 1 unit of SAP at 37°C for 1 hour and 72°C 15 minutes to remove excess fluorescent dye terminators. One microliter of the final reaction samples containing the extension products were added to 9 μL Hi-Di formamide (ABI). The mixture was incubated at 95°C for 5 minutes, followed by 5 minutes on ice, and then analyzed by electrophoresis in an ABI Prism 3730×l DNA analyzer. Analysis was carried out using Genemapper software (version 4.0; Applied Biosystems, Foster City, CA). The sequences of the primers were as follows: forward primer, GAGCACTTTTATGGCACAAATGA; reverse primer, ATAGTAGACAACATACGACCACGCTA; and genotyping primer TTTTCTTGACCCCTACTTAC.
Assay of PON activity
For the serum PON assay, we collected the blood samples from 37 gravida women with preterm deliveries and 298 control gravida women. Serum for PON activity was obtained from serum separator tubes after 30-60 minues of clotting time at room temperature. All specimens were stored at −80°C until the time of analysis. PON (O,O-diethyl p -nitrophenylphosphate, 1.0 mM; Sigma-Aldrich, St. Louis, MO) was used as a substrate, and enzyme activity was measured at 37°C in 50 mM Tris/HCl buffer (pH 6.8; Amresco Inc, Solon, OH) containing 1.0 mM CaCl 2 , (Duksan Pure Chemical Co, Ansan, Gyeonggi-Do, Korea) using an ultraviolet spectrophotometer U-3000 (Hitachi High-Tech, Tokyo, Japan). Serum was added to start the reaction, and the increase in A 405 was recorded. The amount of p -nitrophenol formed/minute was calculated from the molar absorption coefficient at pH 6.8 (7480M −1 .cm −1 ). PON activity was expressed as nmol p -nitrophenol formed/minute.
Statistical analysis
We first examined the relationship between preterm delivery and covariates to be controlled, such as maternal age, maternal education, body mass index (BMI) at delivery, parity, and infant’s gender, using a χ 2 test. The relationship between PON1 and the GSTM1/GSTT1 genotype with preterm births was determined using a χ 2 test. Also, logistic regression was used to calculate the odds of preterm birth for genetic polymorphisms, whereas controlling for potential confounders. To evaluate PON activity according to preterm birth and PON1 genotype, 2-way analysis of variance (ANOVA) was performed. All statistical analyses were performed using SAS (version 8.2; SAS Institute, Cary, NC).
We explored the gene-gene interactive effect of GSTM1, GSTT1, and PON1 on the risk of preterm delivery using the multifactor dimensionality reduction (MDR) method, which is a nonparametric and model-free data mining strategy, making it a unique tool for identifying gene-gene interactions. This method selects 1 genetic model, either single or multilocus, that most successfully predicts phenotype or disease status. In other words, multidimensional space is reduced to either low- or high-risk based on the ratio of number of cases to the numbers of controls within each multifactor class (or in a cell). In this study, the new 1-dimensional multilocus genotype variables (or newly constructed genotype variables in 1-dimension) were evaluated to determine testing accuracy in terms of predicting delivery type on matched pairs of women with preterm births and controls. The prediction error of the model was estimated by (or using) 10-fold crossvalidation. The data (ie, subjects) were randomly divided into 10 equal pairs and the MDR model was generated for the possible 9 of 10 subjects. The model was then used to make predictions about the status of the remaining 1 of 10 subjects excluded. To ensure that the results were not due to chance divisions of the dataset, the 10-fold crossvalidation was repeated 10 times, and the prediction errors were averaged; a nonparametric 1-tailed sign test was performed for the number of crossvalidation intervals with a testing accuracy ≥0.5. Crossvalidation consistency and the lowest prediction error were both used to choose the best model. Because MDR is best suited for equal numbers of cases and controls, we used 160 gravida women with a preterm birth and 160 controls, who were matched for maternal age. The MDR analysis was performed by MDR (version 1.0.0; http://www.epistasis.org/ ).
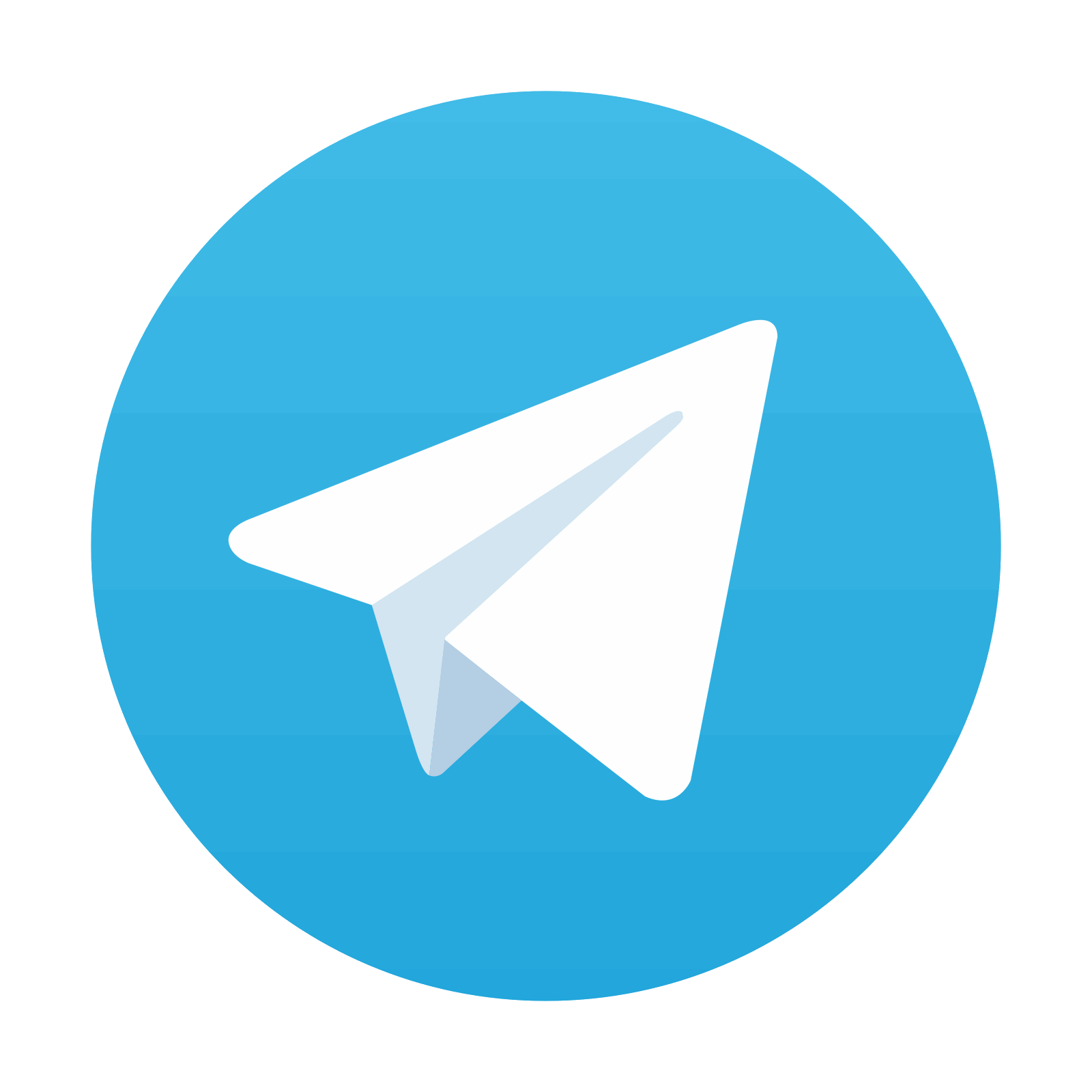
Stay updated, free articles. Join our Telegram channel

Full access? Get Clinical Tree
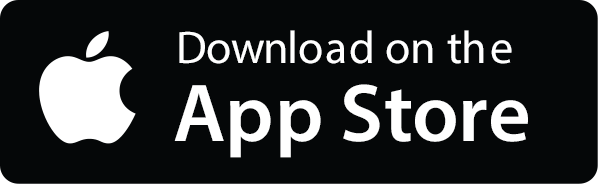
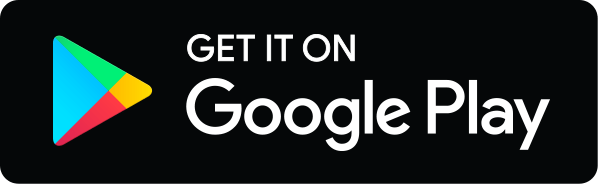