1.1.1.2 Hydroxyl Radical (•OH)
The interaction between hydrogen peroxide (H2O2) and reduced transition metals such as copper and iron results in Fenton reaction, which leads to the formation of hydroxyl radical (•OH). In addition, the exposure of water molecules to ionizing radiation can also result in the production of hydroxyl radical [1, 11, 12].


1.1.2 Oxidation-Reduction (Redox) Reactions
Redox reactions or oxidation–reduction reactions are reactions that involve transfer of electrons between two chemicals. The number of electrons accepted, lost or shared is known as the oxidation number. When the oxidation number of any chemical increases, it is said that this chemical is oxidized and the process is known as oxidation. In contrast, when the oxidation number is decreased, the chemical is reduced and the process is known as reduction C [12, 13]. Iron rust is a good example of a redox reaction. As shown below, iron (Fe) loses electrons to oxygen. Hence, the oxidation number of iron increases while the oxidation number of oxygen decreases. In other words, iron is oxidized while oxygen is reduced at the same time. This is what happens when an iron nail rusts [13].


1.1.2.1 Haber-Weiss Reaction
Haber-Weiss reaction is another example of a redox reaction. In Haber-Weiss reaction, superoxide anion interacts with hydrogen peroxide in the presence of a metal ion (as a catalyst) to produce a hydroxyl radical [13]. The net reaction can be broken down into two reactions. The first step involves the reduction of ferric to ferrous ion; followed by the second step, the Fenton reaction. Along with the Fenton reaction, these two reactions are important in generating the highly reactive hydroxyl radicals [14, 15].






1.1.2.2 Electron Transport Chain (ETC)
Electrons passage through a couple of specialized enzymes known as ETC in the mitochondria is important in cellular energy (adenosine triphosphate, ATP) production. It involves a series of redox reactions. Electrons first enter the chain after the reduction of NADH and FADH2, both of which act as electron carriers. Cytochrome oxidase, acts as the final acceptor of these electrons, enhances the tetravalent reduction of oxygen into water through a process known as oxidative phosphorylation [16]. During this process, a small amounts of oxygen (2–5 %) are univalently reduced into free radicals [17]. Ubisemiquinone, a part of ETC, is considered the main site of electron leak leading to the generation of superoxide anions (Fig. 1.1) [4].


Fig. 1.1
Generation of ROS via electron transport chain
1.1.3 Reactive Oxygen Species (ROS)
The term reactive oxygen species (ROS) includes the reduced form of oxygen and their reaction products with other molecules. Some but not all ROS are free radicals [1]. Superoxide anion and hydroxyl radical are examples of radical ROS, whereas hydrogen peroxide and hypochlorite ion are non-radical ROS (Table 1.1). ROS usually have high reactivity due to the extra-unpaired electron at their outer shells and their half-lives are in the order of milliseconds [10, 18, 19]. The hydroxyl radical is the most damaging radical because it cannot be scavenged easily in our bodies [1, 20].
Table 1.1
Radical and non-radical reactive oxygen species (ROS)
ROS | |
---|---|
Free radicals | Non-radicals |
Hydroxyl radical (•OH) Superoxide anion (•O2 −) Lipid peroxyl (•LOO−) Thiyl (•RS) | Hydrogen peroxide (H2O2) Singlet oxygen (1O2) Ozone (O3) Lipid peroxide (LOOH) Peroxynitrite (ONOO−) |
Intracellular ROS can be produced by stimulation of tightly regulated NADPH intracellular oxidases and peroxidases or as an alternate product by other enzymes such as cytochrome P450 and nitric oxide synthase. Additionally, ROS can be produced by the leakage of electrons from the ETC (Fig. 1.1), which is regarded as the main ROS source in most cells and xanthine oxidase [4, 21, 22].
The univalent reduction of oxygen in the mitochondria generates superoxide anion, which can be further reduced by the action of mitochondrial superoxide dismutase into hydrogen peroxide [21]. As previously mentioned, ubisemiquinone, a component of mitochondrial respiratory chain, is the most common site for this univalent reduction of oxygen. While small amounts of reduced oxygen exist in all functional cells, large quantities of superoxide anion and its derivatives are generated by neutrophils and activated macrophages via the NADPH oxidase pathway [4].
Another ROS production pathway involves xanthine oxidase (XO). The conversion of hypoxanthine into xanthine by XO and xanthine into uric acid yields superoxide radicals [23]. But only a small amount of ROS is released by the activity of XO under normal circumstances. However, increased degradation of ATP as a result of metabolic stress activates XO leading to increased production of superoxide anion [23]. As a matter of fact, XO has been implicated as a major contributor to ROS levels in certain pathological conditions involving ischemia and reperfusion [24].
Inflammation and infection also play a significant role in generating ROS. For instance, a high production of ROS has been noted in endothelial cells induced by tumor necrosis factor (TNF) [25], lymphocytes can produce ROS via the 5-lipoxygenase (5-LO) pathway possibly with CD 40 ligation [26], and cyclooxygenase-1 generates ROS after stimulated by different chemicals including TNFα, interleukin-1, bacterial lipopolysaccharide, and tumor promoter 4-O-tetradecanoylphorbol-13-acetate (TPA).
Interestingly, oxidation of dopamine can also lead to ROS production and this has been shown to be related to neurodegenerative diseases like Parkinson’s disease [4].
1.1.3.1 Physiological Roles
ROS are formed during normal cellular metabolism. They are involved in many physiological processes, including the activation of the immune system. For instance, phagocytes activated as part of the immune system release hydrogen peroxide to kill the pathogens. Superoxide anion is also known to have a role in fighting against infections [1, 27]. On the other hand, ROS released by non-phagocytic cells, including fibroblast, endothelial cells and cardiac myocytes is involved in regulating different intercellular signaling pathways. These cells contain various NADPH oxidase isoforms [4, 5, 28, 29]. Furthermore, physiological amount of ROS is required in spermatozoa capacitation, hyper-activation, acrosome reaction and sperm-oocyte fusion [30].
1.1.4 Reactive Nitrogen Species (RNS)
Reactive Nitrogen species (RNS) are special forms of ROS that contain nitrogen [2]. Similar to ROS, RNS can also include radicals such as nitric oxide (NO) and nitrous acid (HNO2) and non-radicals such as nitrogen dioxide (NO2) and dinitrogen tetraoxide (N2O4) (Table 1.2). Nitric oxide synthase (NOS) can convert l-arginine and oxygen to NO and l-citrulline in the presence of multiple cofactors, including calcium, NADPH, flavin mononucleotide (FMN), calmodulin and flavin adenine dinucleotide (FAD) [31]. Furthermore, different forms of RNS including nitrosonium cation (NO+), nitroxyl anion (NO−) or peroxynitrite (ONOO−) can be produced as a result of the interactions of NO with various chemicals. Although NO is not reactive enough to cause DNA damage, NO can react with superoxide anion to produce peroxynitrite (ONOO−) [32].
Table 1.2
Radical and non-radical reactive nitrogen species (RNS)
RNS | |
---|---|
Free radicals | Non-radicals |
Nitric oxide (•NO−) | Nitrogen dioxide: NO2 |
Nitrous acid (HNO2 −) | Dinitrogen tetraoxide: N2O4 |
1.1.4.1 Physiological Roles
RNS are involved in many physiological mechanisms and processes including production of several hormones and regulation of inflammatory response by inhibiting platelets aggregation and preventing the margination of neutrophils to endothelial cells [33, 34].
NO can alter the heme moiety of guanlate cyclase resulting in alteration in the structure of the enzyme leading to the activation of the enzyme. The activated form of guanylate cyclase results in the production of cGMP, which controls the activity of phosphodiesterases, protein kinases and ion channels. These modulations are required for many physiological processes including the control of the smooth muscle tone and suppressing platelet adhesion [35].
1.1.5 Sources of ROS in Male Reproductive System
1.1.5.1 Generation of ROS
Superoxide anion is considered to be the most abundant ROS in human sperm and it is the precursor of other ROS types. Accepting another electron to form hydrogen peroxide and reacts with other radicals such as nitric oxide to form peroxynitrate can further reduce superoxide anion. Finally, it can also react with itself by dismutation, a reaction that is catalyzed by SOD to produce hydrogen peroxide. In the presence of catalase and a transition metal, hydrogen peroxide is converted to the hydroxyl radical, one of the strongest oxidants in nature [9].
The generation of such ROS occurs via two methods: (1) in the cell membranes, using NADPH oxidases, and (2) in the mitochondria, using NADH oxido-reductase.
NADPH Oxidases
NADPH oxidase enzymes are located in the cell membranes of the spermatozoa [9]. NADPH oxidases are a group of enzymes that catalyses the conversion of molecular bivalent oxygen to superoxide. The substrates for these reactions are NADPH molecules generated by the hexose monophosphate shunt. NOX 5 gene was found to be expressed in NADPH oxidase in the spermatozoa, located both in the flagella/neck region and the acromsome and hence, may involve in this process [36].
Electron Leakage in the Mitochondria
ROS are generated by sperm mitochondria under normal cellular respiration. Spermatozoa are rich in mitochondria because they constantly require ATP for motility [37]. Electron leakage from cytochromes in the ETC sources the monovalent reduction of oxygen to the superoxide anion. There is a link between ROS production and NADH oxido-reductases activity in the inner and outer mitochondrial membranes within respiring spermatozoa. NADH oxido-reductase (ubiquinone oxido reductase) catalyses electrons transfer from NADH to coenzyme Q10 in the ETC. Electron leakage occurs during reverse electron transfer, which acts as a source of electrons for the reduction of oxygen to the superoxide anion.
Hyperoxic conditions also increase ROS production [38]. When sperm respiration increases due to an increased need for ATP, the concentration of molecular oxygen in the matrix of the mitochondria increases thus leading to increased superoxide production as well. However, it is interesting to note that ROS production also increases under hypoxic conditions as ROS producing sites in mitochondria have a higher affinity for oxygen than the cytochrome oxidases under such conditions [39].
1.1.5.2 Sources of ROS in Seminal Plasma
Immature/Abnormal Spermatozoa
A marked difference has been noted in the quantity of ROS produced by spermatozoa at different stage of maturation, suggesting that the level of maturation affects ROS production [40].
Immature spermatozoa are characterized by retaining excessive residual cytoplasm (ERC) around their mid-piece, which is normally expelled during spermiogenesis. ERC contains high levels of glucose-6-phosphate dehydrogenase (G6PDH), a cytosolic enzyme that utilizes the hexose monophosphate shunt to produce abnormally high levels of NADPH. Excessive NADPH results in a greater production of superoxide anions by NADPH oxidases [41]. A positive correlation of high ROS levels with excessive cytoplasmic retention in spermatozoa is documented [42].
Furthermore, abnormal morphological sperm produce more ROS than their normal counterparts [43]. In one study, a negative correlation was observed between the production of ROS and the number of healthy sperm with normal or borderline morphology. In the same study, a positive correlation of was seen between ROS production and the number of sperm with amorphous heads, tail defects, excess cytoplasm and abnormal midpiece [44].
Leukocytes
Activated leukocytes (peroxidase positive) produce large quantities of ROS and it has been shown that leukocytes are the predominant source of ROS in raw human semen. ROS produced by leukocytes is believed to account for toxicity against human sperm.
Peroxidase positive leukocytes are primarily macrophages and polymorphonuclear (PMN) leukocytes originating from the prostate and seminal vesicles [40]. Such leukocytes are often found in the seminal fluid. In one study in which seminal oxidative stress (OS) was artificially-induced, removal of leukocytes by “Dyanabeads” reduced ROS levels significantly [45].
Although it is generally accepted that an association exists between the number of leukocytes in the seminal fluid and excessive ROS production, OS can still result in the absence of leukocytospermia. Of note, one study reported that ROS production from PMN up to a concentration of 0.5 × 106/ml is negligible [46].
On the contrary, when PMN leukocytes are activated by stimuli like infection and/or inflammation, a discharge of ROS ensues [47]. For instance, neutrophils that contain myeloperoxidase use superoxide anions to oxidize chloride ions. This results in the production of hypochlorous acid (HOCL), which acts as an anti-bacterial agent but is also a kind of ROS. Moreover, leukocytes increase the production of NADPH by the hexose monophosphate shunt, thus increasing the activity of NADPH oxidases that ultimately increase the production of superoxide anion.
As a matter of fact, a strong association exists between the presence of male accessory gland infections (MAGI) and seminal ROS levels. Prostatitis, prostato-vesiculitis and prostato-vesiculo-epididymitis are the commonest MAGI; the most prevalent being prostatitis. The immune response to MAGI results in an increased number of leukocytes in the male genital tract, which subsequently leads to ROS production [9].
Studies have also linked bacterial prostatitis and the autoimmune response to prostate antigens with excessive ROS production [48]. Although it is generally accepted that granulocytes in the prostatic fluid are a source of ROS, semen of individuals suffering from chronic prostatitis that do not contain leukocytes may also have elevated ROS levels, suggesting that another source of ROS exist in the prostate gland.
The degree of OS is increased when the prostatitis is associated with seminal vesiculitis. Along the same lines, when compared to prostato-vesiculitis and prostatitis, a higher number of white blood cells and ROS levels are observed in prostato-vesiculo-epididymitis [49].
Varicocele
Varicocele is the dilatation of veins in pampiniform plexus around the spermatic cord. Studies have associated varicocele with many abnormal sperm parameters, including DNA damage. The latter has been linked to increased levels of ROS and OS. The higher the varicocele grade, the higher the ROS production [30].
1.2 Conclusion
Despite the strong evidence indicating that animal sperm make ROS, the ability of human sperm to produce significant amounts of ROS is surrounded by controversy. Notwithstanding, some evidence that human sperm produce ROS exists but experiments should ensure that sperm preparations are free of leukocytes, since leukocytes are prolific sources of ROS.
1.2.1 Sources of ROS in Female Reproductive System
While the role of ROS has been extensively investigated in the male reproductive system, the literature is relatively poor in studies addressing the role of ROS in the female counterpart. Nonetheless, ROS have been shown to be associated with pathologies of the female reproductive system, including preeclampsia, polycystic ovary syndrome, recurrent pregnancy loss and endometriosis [3].
In the female reproductive system, ROS are mainly found in the cells of ovarian follicles, the fallopian tube, the endometrium and the peritoneum.
1.2.2 Steroidogenesis and Ovulation
Completion of meiosis I during oocyte maturation is induced by an increase in ROS and is inhibited by an increase in antioxidants. The contrary occurs during meiosis II, thus indicating that ROS play an important role during the pre-ovulatory states [50]. Besides, steroidogenesis is also linked to increased ROS production in the ovaries by utilizing the cytochrome P450 pathway [51].
An earlier study in the rat suggested that ROS levels in corpus luteum increased during the regression phase [52]. Newer discoveries shed light to the relationship between different hormones and ROS production in the luteal phase. In the growing follicle, ROS induces apoptosis whereas FSH and reduced glutathione (GSH) are anti-apoptotic. FSH stimulates estrogen production within the granulosa cells, which in turn increases catalase that inhibits apoptosis [53].
On the contrary, excessive production of LH increases ROS production. The LH surge in the ovulatory is followed by a post-surge inflammatory phase. If LH secretion is in excess, the post-surge inflammatory precursors may increase thus causing OS-induced damage due to the overly produced ROS [21, 54].
After ovulation, the corpus luteum produces progesterone. It was found that both copper/zinc-superoxide dismutase (Cu/Zn-SOD) and progesterone levels increase in the early-mid luteal phase but decline in the regression phase. Lipid peroxide opposes Cu/Zn-SOD and progesterone in both phases [21, 54]. On the contrary, manganese-superoxide dismutase (Mn-SOD) is not affected by progesterone levels and is independent in its action, whereby its levels are sustained to protect the luteal cells from OS-induced inflammation [21].
1.2.3 Graffian Follicle
The Graffian follicle has been studied as a potential source of ROS because it contains macrophages, neutrophils, granulosa cells and the oocyte [21].
The oocyte has shown to be a source of ROS by different mechanisms. First, oxidative phosphorylation within the oocyte utilizes O2 to produce energy (ATP). Second, the XO system eliminates toxic wastes in the form of uric acid, but at the expense of generating ROS. Lastly, the NADPH oxidase system whereby the oxidative burst produces HOCl to kill microbes. All these systems are prominent sources of ROS in the oocyte [21].
Aging is defined by the gradual loss of organ and tissue functions. The ageing of oocytes was linked with ROS production that may increase oocyte damage [20]. This hypothesis has led researchers to speculate that ROS might be related to the occurrence of congenital anomalies in neonates seen originated form older women [21]. It has been observed that oocytes from women at advanced reproductive age carry dysfunctions at the mitochondrial DNA level and chromosomal aneuploidy The marker that has been utilized to assess mitochondrial DNA (mtDNA) damage is an oxidized derivative of the deoxyguanosine base, namely oxodeoxyguanosine (8-OHdG) [54].
Menstrual blood is one pivotal way females lose iron (Fe) As discussed earlier, Fe potentiates oxidative damage—and its build-up in the body poses a higher risk on menopausal women to OS-related diseases [21]. Menopause also causes decreased production of estrogen along with its protective properties against oxidative damage of the endometrium [55].
1.2.4 Granulosa Cells and Cumulus Mass Cells
The granulosa cells are one of the main sources of ROS in the graffian follicle. Undifferentiated granulosa cells give rise to the cumulus oophorus, which anchors the oocyte and keeps it in place. SOD is the main antioxidant present in these cells to counteract the ROS production [20, 56]. OS in the granulosa cells and cumulus oophorus may damage the cell contents, including DNA [54]. There has been shown that a positive correlation between 8-OHdG levels in the granulosa cells and poor oocyte quality [57, 58] but the exact mechanisms of ROS production in the granulosa cells and cumulus mass remain unclear.
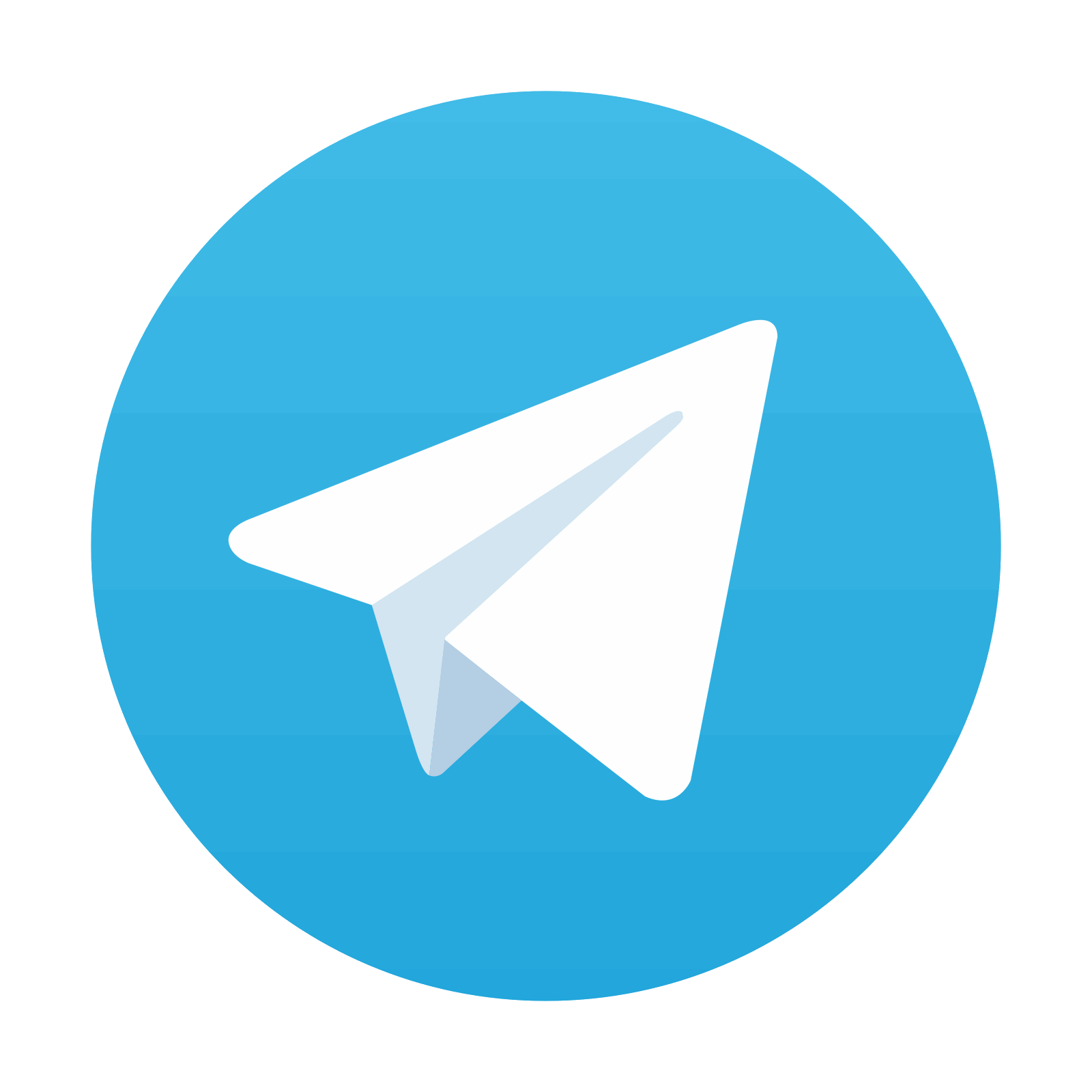
Stay updated, free articles. Join our Telegram channel

Full access? Get Clinical Tree
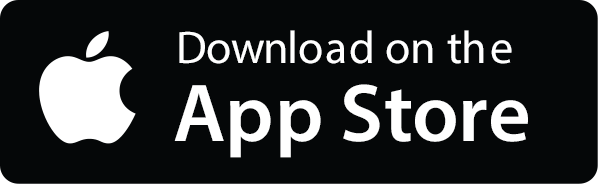
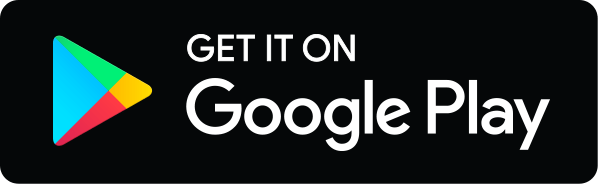