Fig. 4.1
The effect of lipid peroxidation of 4-HNE on sperm motility
Sperm Morphology
Normal range of spermatozoa morphology values along with other semen parameters have been lowered in the past few decades [25]. The average value for normal sperm morphology is about 4 % [26]. In a study of men with good sperm motility and concentration but poor morphology, it suggested that the sperm morphology best reflects its fertilization potential.
Most of the morphological abnormalities have been reported to be caused by genetic and chromosomal abnormalities such as aneuploidy of the sex chromosomes [27]. However, OS can also cause morphological defect in the spermatozoa, impairing its fertilizing ability [28]. A study showed that increased proportion of spermatozoa with mid-piece defects had increased production of ROS [29]. These mid-piece defects have been negatively correlated with in-vitro fertilization rates [30]. The presence of excessive cytoplasm has been speculated as the cause of such ROS production [31].
Furthermore, Agarwal et al. showed that men with normal white blood cell levels and normal spermatozoa parameters but manifesting teratozoospermia (abnormal sperm morphology) displayed increased production of ROS [28]. This further suggests abnormal morphology of spermatozoa contributes to infertility by increasing ROS production.
Apart from mid-piece defects, it has also been shown that OS can cause amorphous head defect in the spermatozoa [32].
4.1.1.2 Lipid Peroxidation
Lipid peroxidation (LPO) is a consequence of OS, which leads to the oxidation of membrane lipid components. This process impairs the fluidity of the cell membrane, inactivates membrane-enzymes and increases its permeability for non-specific ions [34].
Spermatozoa are highly susceptible to OS due the presence of high amounts of polyunsaturated fatty acids in their cell membranes that weakens the methyl carbon-hydrogen bond [35]. Interestingly, Huszar et al. reported a positive relationship between creatine kinase activity (which involves in reflecting the degree of cytoplasmic extrusion) and the rate of LPO (as measured by MDA formation), indicating that creatine kinase could be used as a biomarker of OS [36].
4.1.1.3 Mitochondrial Membrane Potential (MMP)
Like all cells, spermatozoa contain mitochondria for various normal physiological processes. But unlike other cells, spermatozoa mitochondria are physically separated in a subcellular compartment known as the mid-piece, which indicates how crucial and specific its functionality is [37]. The membrane potential of the mitochondria is strictly regulated in order for it to function properly [35]. Any disruption in highly regulated function could cause the disruption and breakdown of the organelle and cell as a whole.
One of the factors that can alter mitochondrial potential is OS, which ultimately leads to the disruption in mitochondrial homeostasis. It does so by affecting the mitochondrial membrane potential (MMP), which could depict a negative correlation with functional spermatozoa parameters, such as motility and fertilization ability. Electrophilic aldehydes that are generated from OS-induced LPO, enter the mitochondria, inhibit the electron transport chain, results in the leakage of large amounts of ROS. The ROS then attack the membrane of the mitochondria and affect its integrity by altering the MMP [22].
MMP can also be affected by spermatozoa released from the germinal epithelium carrying surplus residual cytoplasm as a result of impaired spermatogenesis. This retention of residual cytoplasm has been positively correlated with increased ROS generation and has been suggested to be driven by the cytosolic enzyme glucose-6-phosphate dehydrogenase [38, 39]. In the event of elevated ROS levels, lipid metabolism and redox reaction responsible genes are also affected [40]. In a study on testicular inflammatory disease, it was reported that the MMP showed marked reduction as a result of the testicular inflammation [41]. Rapid loss of adenosine tri-phosphate (ATP) due to LPO also causes spermatozoa morphological defects, and a decrease in viability ultimately decreasing the motility [42, 43]. In contrast, no effect of MMP on spermatozoa motility has been reported, which further supports that metabolic pathways and processes involved in motility are not fully understood yet [44].
4.1.1.4 Protein Phosphorylation
The tyrosine phosphorylation of a protein is of high importance, not only because it plays a role in the activation of specific proteins but also its role in flagellar motility in the spermatozoa of many species [16, 45]. For example, the spermatozoa axoneme, as previously mentioned, is activated by phosphorylation [45–49]. Several other proteins such as hexokinase and AKAP82, which were found to be phosphorylated have also been suggested to be involved in the activation of sperm motility [50]. The phosphorylation of these proteins usually involves cAMP-dependent protein kinases [46, 47].
It has been suggested that elevated ROS levels may lead to a decrease in axonemal protein phosphorylation and thus, reduce membrane fluidity [51, 52]. Interestingly, RNS, a subclass or ROS cause a toxic effect when they interact with superoxide anions to form peroxynitrite (Fig. 4.2) [53, 54]. Peroxynitrite and its breakdown products can induce peroxidative damage and nitrosation of trysoine molecules responsible for signal transductions [55, 56]. Furthermore, it affects the kinases and phosphatases used in capacitation, thereby disrupting tyrosine phosphorylation and lowering its fertilizing potential [57]. Spermatozoa of infertile patients fail to undergo tyrosine phosphorylation, and this could suggest that OS has a role in this impairment, as it is one of the leading causes of infertility [58].


Fig. 4.2
Illustration of involvement of NO synthase in forming RNS and ROS, leading to the formation of lipid hydroperoxides, which could result in necrosis and apoptosis
4.1.1.5 Acrosome Reaction
Prior to binding and penetration of the oocyte zona pellucida, the spermatozoa must be able to undergo acrosome reaction; a process by which proteolytic enzymes are released from the acrosome in order to facilitate zona pellucida binding and sperm surface remodeling in preparation for sperm-oocyte fusion [59]. Controlled ROS levels are required for triggering the acrosome reaction as well as promoting the binding ability of human spermatozoa with the zona pellucida [44]. However, excessive amounts of ROS impair membrane dependent processes such as acrosome reaction and sperm-oocyte fusion [60, 61].
4.1.1.6 DNA Damage
A healthy and intact spermatozoa DNA is required for fertilization. Any alteration in DNA structure could alter or lead to loss of function of proteins [62]. An example would be alteration and deletion in mitochondrial DNA which negatively influence cellular homeostasis and leads to a decrease in spermatozoa functionality [44]. Spermatozoa and seminal plasma with low antioxidant enzyme levels caused by homeostatic imbalances have been associated with impaired spermatozoa function and DNA integrity. This usually occurs when a cell is under OS induced by ROS or RNS [23, 63].
High concentrations of NO have been found to induce detrimental effects on mitochondrial and nuclear DNA via deamination, oxidation and nitration [64–66]. Moreover, elevated levels of aneuploidy have been positively correlated with increased levels of spermatozoa DNA damage, potentially resulting in genetic abnormalities [67]. Another cause of DNA damage results from LPO induced by OS, in which the electrophilic aldehydes induce the nuclear DNA damage [22]. The type of DNA damage could be in the DNA structure as a whole or on proteins at the molecular level. This occurs in the form of base modifications, deletions, cross-links or even frame shifts [5]. In addition, RNS can also cause chromosomal rearrangements and microdeletions [68, 69]. Also, it has been observed that single stranded breaks in the DNA of spermatozoa are a result of OS, while double stranded breaks are also a result of 4-HNE exposure, a major product of LPO [70].
Although spermatozoa possess the first base excision repair (BER) enzyme for DNA damage repair, the lack of the second enzyme of the process, oxoguanine glycosylase (OGG1), is a reason for their susceptibility to OS as this damage can only be repaired by the oocyte after fertilization [71]. The intrinsic apoptotic pathway of spermatozoa does not seem to have an apparent increase in DNA fragmentation because the spermatozoa mitochondria, which are a source of ROS generation, and the nucleus, are in two different physically separated subcellular compartments [22]. As stated earlier, the integrity and correct DNA modifications are required for proper fertilization to occur [62]. Sperm DNA damage has been associated negatively with live-birth rates after IVF [72].
4.1.1.7 Sperm-oocyte Fusion
For sperm-oocyte fusion to occur, a spermatozoon must first penetrate the cervical mucous membrane in the female reproductive tract to be able to fuse with an oocyte. The capacity of spermatozoa to fuse with an oocyte is related to their ability to penetrate the cervical mucous membrane [73]. Following penetration, the spermatozoon must be able to undergo capacitation to further activate the cell. This process is triggered only when a spermatozoon detects the ionic and environmental changes in the female reproductive tract [16].
Like many reproductive physiological processes, capacitation requires a low and controlled level of ROS for sperm-oocyte interaction by increasing the membrane fluidity and fusion rates [74–76]. Physiological levels of NO, hydrogen peroxide and superoxide anions are important in the regulation of tyrosine phosphorylation that is associated with capacitation [38, 61, 77]. An elevated ROS levels in the genitourinary tract is associated with poor sperm-oocyte fusion. Disturbances such as OS-induced DNA damage, in either spermatozoa or oocyte integrity, will have an impact on their functions that will ultimately affect the chances of fusion and thus fertilization [78, 79]. OS not only damages the DNA but also causes LPO, resulting in a poor sperm-oocyte fusion and adhesion [19–21].
4.1.1.8 Fertilizing Capacity
Sperm require low (physiological) amounts of ROS for proper fertilizing ability to occur during capacitation [24]. This occurs when ROS triggers the activation of adenylate cyclase, increases intracellular calcium levels and phosphorylating protein kinases rendering them active [47, 80–82]. In a study using in vitro fertilization (IVF) as a biological end point, hyperactivation was found to be the most critical single characteristic of sperm movement that predicts the fertilizing potential of human spermatozoa [83, 84].
Elevated ROS levels have been associated with abnormal spermatozoa development and fertilizing capacity [74]. Henkel et al. examined IVF and ICSI outcomes in patients with ROS induced spermatozoa DNA damage and showed that the women had low pregnancy rate [85]. RNS have also been implicated in poor spermatozoa function and fertilizing ability [86–88]. Another study showed that spermatozoa with a deficiency in superoxide dismutase 1 (SOD1) caused a decrease in fertilizing ability during IVF procedures. This was attributed to increased LPO, decreased ATP levels and malfunctioning of tyrosine phosphorylation [89].
Furthermore, a study conducted by Yeung et al. proposed that ROS could have another positive influence on fertilization capacity [90]. However, another study on patients with oligozoospermia undergoing ICSI concluded that patients with spermatozoa concentration below 10 × 106 sperm/mL showed significantly lower fertilization rates [91]. Interestingly enough, some studies suggested the possibility that excessive antioxidant levels could restrain spermatozoa fertilization capacity by lowering ROS levels below the physiological levels [92–94]. Despite the known negative effects of ROS, some studies have proven otherwise, that ROS may not have any negative impact on IVF and intracytoplasmic injection (ICSI) outcome [90, 95]. Establishing an ROS cut-off value for fertilization rates and pregnancy outcomes is still controversial because there is still nonconformity with the study design and protocols used for ROS measurement [96].
4.1.1.9 Apoptosis
Apoptosis is defined as programmed cell death, which is essential in an organism’s development [97]. Apoptosis is an important phenomenon for the male germ line in early development as well as in adulthood [22]; it regulates the germ cell-Sertoli cell ratio and eliminates damaged germ cells from the seminiferous tubules in response to stimuli [22].
Any disruption in spermatogenesis will cause the germ cells to default to apoptosis, namely the Fas/FasL apoptotic cascade [98]. Sertoli cells regulate the induction of apoptosis and remove about 50 % of the cells during meiosis I of spermatogenesis by marking them with Fas type apoptotic markers for phagocytosis and elimination [99–101]. However, defective or abortive apoptosis can cause cells that are destined to be eliminated to escape apoptosis and get released in the ejaculate, leading to abnormalities in the embryo after fertilization [102].
Elevated levels of RNS damage in the mitochondrial membrane induce cytochrome-c release and activate the caspase cascade for initiation of apoptosis [68, 69]. A study observed that increased levels of H2O2 in mature spermatozoa had an increase in the percentage of apoptotic spermatozoa; however, there was no significant increase in the percentage of dead sperm [103, 104]. Another study by Mahfouz et al. showed that addition of H2O2 to mature spermatozoa decreased both apoptotic and dead cells [105]. Although apoptosis is sometimes required and useful for physiological functions, OS has an impact on spermatogenesis and transduction pathways of apoptosis. As this process is not fully understood, more studies need to be conducted to further explain the mechanism of action and the pathways involved.
4.1.2 Negative Effects of Oxidative Stress in Female Reproductive System
4.1.2.1 Follicular Fluid
Follicular fluid provides an optimal microenvironment for the maturation of the oocyte before fertilization and can influence the IVF outcome parameters such as fertilization, embryo cleavage, and pregnancy rates [106]. Follicular fluid originates from the transfer of the blood plasma components, which crosses the follicular-blood barrier and from secretory contributions by granulosa and theca cells. The fluid contains steroid hormones, growth factors, leukocytes, cytokines and macrophages. All of these factors can lead to elevated ROS production [107, 108].
Adequate level of ROS is important in fertilization and implantation. Oxidative stress, on the other hand, influences the oocyte and embryo quality and thus the fertilization rate [109]. However, the existing literature regarding the impact of ROS on reproductive outcomes is controversial. A study done by Jozwik et al. reported that the concentration of oxidative stress markers such as conjugated dienes, lipid hydroperoxides and thiobarbituric acid-reactive substances in follicular fluid did not reflect the reproductive potential of oocytes [110]. In contrast, Attaran et al. reported higher levels of ROS in women who became pregnant with IVF [107], whereas Pasqualotto et al. reported elevated follicular fluid LPO in women who became pregnant versus those who did not [111].
High levels of ROS or low levels of antioxidants can affect oocyte maturation. SOD, has a crucial role in protecting the oocyte during their maturation phase by converting superoxide anions to less harmful substances like hydrogen and water [112]. Higher total antioxidant capacity (TAC) level increases fertilization potential in women undergoing IVF [113]. Deficiency of selenium-dependent glutathione peroxidase levels has also been reported to result in fertilization failure [114]. This is in agreement with studies conducted on genetically manipulated mice where a decrease in the litter size and number of litters per month was seen with deficiency of superoxide dismutase (SOD) level [115, 116]. Persistently elevated ROS level can ultimately lead to toxic effects on the embryos and impairs cleavage patterns of the embryos [117]. Borowiecka et al. evaluated the levels of lipid and protein peroxidation markers in the follicular fluid of patients undergoing IVF. Oxidative stress markers were examined in pregnant women versus those that were not. A two-fold increase in levels of protein carbonyl was reported in non-pregnant women. The study demonstrates that elevated levels of lipid and protein peroxidation levels in the fluid might have a negative impact on IVF outcome. Their findings support the idea that increased level of oxidative stress markers in follicular fluid is an important predictor of fertility outcomes [118].
Bedaiwy et al. reported higher follicular fluid TAC, fluid ROS-TAC scores and lower follicular fluid ROS levels were associated with pregnancy after ICSI [119]. Follicular fluid NO concentrations have been negatively associated with embryo quality and rate of cleavage [120]. Although ROS may serve important physiological roles within the ovary, the cyclical production of these damaging agents over years may lead to a higher cumulative risk of ovarian pathology that would probably be exacerbated under conditions of reduced antioxidant status [121].
Das et al. examined the effect of ROS on follicular fluid of women undergoing IVF and related these levels to embryo formation and quality. A favorable effect of ROS on percentage of embryo formation with ROS levels of up to 100 counted photons per second (cps) in grade II and grade III oocytes have been reported and embryo formation declines at ROS levels higher than 100cps. An overall negative correlation was reported between higher follicular fluid ROS and embryo quality [122].
Basini et al. reported that oxidative stress did not affect swine follicular growth although concentrations of hydrogen peroxide, hydroperoxides were significantly reduced during follicle growth. In addition, these authors reported significantly reduced concentrations of all enzymatic and nonenzymatic antioxidants levels with the exception of catalase during follicle development, suggesting that other factors may be involved in ROS detoxification during follicle development [123]. Similarly, during follicle maturation, the theca interna cells play an important role in protecting the developing oocyte from ROS by acting as blood-follicular barrier [124].
Antioxidants block the resumption of meiosis, whereas the generation of ROS initiates oocyte maturation in the follicle. Similarly, other investigators have shown that high levels of SOD activity in the follicular fluid are associated with a smaller success rate of IVF that are characteristic of women of advancing age [125, 126]. A positive correlation between the antioxidant capacity of the follicular fluid and that are successfully fertilized or augmented steroidogenesis has been reported [113, 127]. Apoptosis of granulosa cells can induce OS, and negatively affect the follicle development and result in poor oocyte quality in the presence of endometriosis [128].
A recent investigation examining oxidative stress and poor oocyte quality among women undergoing IVF and embryo transfer indicated that concentration of 8-OHdG (a measure of DNA oxidation) was significantly greater in the follicular fluid with a high rate (≥30 %) of degenerative oocytes [129]. Shaeer et al. measured the OS markers such as lipid peroxidation (MDA), nitrite/nitrate ratio (NO2/NO3 ratio), reduced glutathione (GSH), reduced glutathione/oxidized glutathione ratio (GSH/GSSG ratio) by high performance liquid chromatography (HPLC) in follicular fluid. The study demonstrates that follicular fluid OS is aggravated in obesity and has a negative impact on pregnancy outcome of ICSI cycles [130].
Lower follicular fluid concentration of β-carotene with associated lower IVF success rates has been reported among smokers [131], suggesting that follicular loss of β-carotene occurs in response to tobacco-related oxidative stress. Both active and passive smoking may require increased antioxidant requirements [132, 133].
In obese and infertile women, presence of oxidized low-density lipoprotein (oxLDL) and enzyme antioxidants in the follicles (intrafollicular) may be a contributory factor in reproductive disorders [134]. These investigators measured the concentrations of oxLDL and the activities of superoxide dismutase (SOD), catalase, glutathione peroxidase and glutathione reductase in the serum and follicular fluid of obese and non-obese women. A thousand-fold lower level of oxLDL was seen in the follicular fluid compared to the levels in the serum. Higher levels were seen in the catalase activity and not in the SOD levels. Elevated levels of oxLDL in the follicular fluid of obese women were associated with higher catalase activity indicating excessive oxidative stress [134].
An association between adverse effects of OS on oocyte meiotic spindle (MS) assembly have been demonstrated in mouse oocytes [135]. These results corroborate the findings that non-visualization of MS is fairly correlated with higher follicular levels of ROS. Women with PCOS were reported to have a number of oocytes with meiotic spindle visualization and higher mean ROS levels in follicular fluid. This indicates a poorer oocyte and thus embryo quality in these women, which may be attributed to an excessive generation of ROS and LPO, and to an impaired antioxidant defense mechanism in follicular fluid of PCOS women. In contrast, women with endometriosis were observed to have a low follicular fluid oxidative stress status and good meiotic spindle imaging results, suggesting a possible role of endometrial receptivity rather than oocyte quality accounting for low pregnancy rates in these women [136].
In the existing literature, some studies suggest optimum level of follicular fluid ROS to be a marker for predicting success in IVF cycles [137], others have demonstrated a toxic effect of high ROS levels in follicular fluid on oocytes and lower pregnancy rates [113, 138]. Recent studies also indicate high follicular fluid ROS levels tend to decrease the fertilization potential of oocytes; furthermore, a higher percentage of good quality embryos were observed corresponding to ROS levels <100 cps [139].
4.1.2.2 Peritoneal Fluid
Peritoneal environment and associated peritoneal fluid have a complex role in OS induced female infertility. Reactive oxygen species (ROS) are produced by peritoneal fluid mononuclear leucocytes in endometriosis patients [140]. Production of ROS is known to increase after activation of immune cells, especially polymorphonuclear leukocytes and macrophages. Consequently, ROS are likely to be important mediators in the etiopathogenesis of endometriosis [140, 141].
Murphy et al. reported increased concentrations of oxidized low density lipoproteins in the peritoneal fluid of women in whom the disease was developing [142, 143]. Oxidative modification of these molecules involves peroxidation of the lipid component, which leads to release of aldehydes, such as MDA, and reaction with lysine residues of proteins [144]. It has been hypothesized that endometriosis is associated with ROS in the peritoneal and tubal fluid, adversely affecting sperm motility and function [145], and increasing the growth and adhesion of endometrial cells in the peritoneal cavity [142, 146]. Sperm motility has been negatively correlated with peritoneal fluid volume [147, 148], suggesting the possibility that increased fluid volume may be a causative factor in endometriosis-associated infertility. Zeller et al. observed a significant increase in ROS production by resting peritoneal macrophages in peritoneal fluid samples collected during mid-luteal phase of menstruation in women with endometriosis compared with fertile controls [140]. It is hypothesized that peritoneal fluid diffuses into the fallopian tubes where it may cause damage to sperm, as they are known to be sensitive to OS [149]. Peritoneal fluid of women with idiopathic infertility demonstrated a higher concentration of ROS compared with fertile controls, but these levels were not significantly different from women with endometriosis [148].
In the presence of macrophages, cytokines are released in the peritoneal fluid due to inflammation. Cytokines such as interleukin (IL) 1,2,5,8 and 10 and tumor necrosis factor (TNF) may also be implicated in infertile patients with endometriosis [141]. Significantly lower levels of vitamin E have been reported in the peritoneal fluid of women with endometriosis, as a result of the increased OS [150]. OS is also known to be the contributing factor in the formation of intraperitoneal adhesions; one of the key factors implicated in infertility associated with endometriosis [151].
Peritoneal fluid of women with endometriosis-induced infertility has been found to have elevated ROS and/or poor antioxidant defense mechanisms [152–154]. While some studies failed to observe increased OS in the peritoneal fluid of patients with endometriosis [148, 155, 156], others have reported increased levels of OS markers in women with infertility [142, 150, 157–159]. High concentrations of MDA, pro-inflammatory cytokines (IL-6, TNF-alpha, and IL-beta), angiogenic factors (IL-8 and VEGF), monocyte chemo-attractant protein-1 [160], and ox-LDL [161] have all been reported in the peritoneal fluid of infertile patients. Pro-inflammatory and chemotactic cytokines play a central role in the recruitment and activation of phagocytic cells, which are the main producers of both ROS and RNS [160]. The elevated levels of ROS have detrimental effects on gametes. Mansour et al. demonstrated that oocytes incubated with peritoneal fluid of women with endometriosis exhibited increased DNA damage [162]. A concurrent study by the same group showed that even spermatozoa co-incubated with peritoneal fluid of women with endometriosis exhibited increased DNA fragmentation [162, 163].
Patients with endometriosis tend to have lower pregnancy rates than those without the disease. In addition to the toxic effect on spermatozoa, low oocyte and embryo quality may be mediated by ROS and this may contribute to the subfertility experienced by patients with endometriosis [164]. Intraperitoneal administration of melatonin, a potent scavenger of free radicals, has been shown to cause regression of endometriotic lesions in rat endometriosis explant models [165–167] by neutralizing OS [168, 169].
4.1.2.3 Hydrosalpingeal Fluid
Hydrosalpingeal fluid (HSF) is fluid that is produced in an inflamed fallopian tube, either unilaterally, or bilaterally [170]. Hydrosalpinx is caused by occlusion of the fimbriated end of the fallopian tube near the ovary, and retention of HSF in the site, followed by swelling and distension triggers the loss of its anatomical shape and its functionality. Hydrosalpinx represents a post-inflammatory state of OS. It exhibits elevated production of ROS and MDA levels and low total antioxidant capacity [171]. The tubal fluid originating from the hydrosalpinx has been shown in a murine experimental model to have embryotoxic properties. The development of blastocysts was inversely correlated to the percentage of hydrosalpingeal fluid added to the murine two-cell embryo culture when compared with controls without HSF. These correlated positively with the production of ROS [171]. Several possible mechanisms for the embryotoxic properties of the hydrosalpingeal fluid have been suggested, including presence of microorganisms, endotoxins, cytokines, OS and lack of nutrients [172].
Bedaiwy et al. hypothesized that blastocyst maturation is dependent on low levels of ROS in hydrosalpingeal fluid, and increased concentration of the above mentioned parameters (except LPO, which did not show any significant change) are indicative of improvement in blastocyst growth [171]. Several research studies have examined the mechanisms by which the HSF exerts embryotoxicity. Some of the proposed mechanisms for hydrosalpingeal embryotoxicity include oxidative stress, toxins in the fluid, altered endometrial receptivity or the embryos are washed away. Chan et al. reported the mechanistic pathways by which hydrosalpingeal fluid exerts toxicity using the rat embryo model. They investigated the impact of hydrosalpinx fluid on rat embryos by assessing the levels of 8-iso prostaglandin f2-alpha levels and protein content of mouse embryos. They did not find a correlation between higher levels of OS markers and morphologic abnormalities in the embryos [173]. Bedaiwy et al. aspirated hydrosalpingeal fluid from 11 infertile women with confirmed hydrosalpinges. ROS and enzymatic TAC (both measured by chemiluminescence), and lipid peroxidation (measured by TBARS) were quantified. 2-cell mouse embryos were incubated with 25, 50 or 75 % hydrosalpingeal fluid and observed for blastocyst development. Blastocyst development was inversely correlated with concentration of hydrosalpingeal fluid. It was demonstrated in embryos cultured in 75 % hydrosalpingeal fluid compared to control embryos. Lipid peroxidation was not significantly related to blastocyst development, and TAC was detectable in only 18 % of samples, although TAC was at a level unlikely to affect blastocyst development. Blastocyst development was positively correlated with ROS concentration, but overall low ROS concentration was suggestive of healthy endosalpinx rather than a concentration potentially deleterious to the developing embryos [171].
Embryotoxicity is normally associated with the presence of hydrosalpingeal fluid that is not necessarily caused by an excess of ROS. The excess ROS levels detected in hydrosalpingeal fluid may originate as byproducts from other natural physiological processes such as cellular respiration. Higher levels of ROS may also be generated by the inflammatory response resulting from chronic salpingitis [174]. The definite reasons for embryotoxicity associated with hydrosalpinx are not completely identified and the recommendations are to surgically remove hydrosalpinx before IVF.
4.1.2.4 Oocyte Quality and DNA Oxidation
A mature competent oocyte is required to achieve fertilization. In order to accomplish this, the oocyte needs to be nurtured in an optimal microenvironment. Among several factors attributing to detrimental influences of cellular damage to the oocyte and the cellular response to protect it, the mechanism of ROS induced damage is important (Fig. 4.3). 8-Oxo-2′-deoxyguanosine (8-oxo-dG) is one of the products of DNA oxidation, and any increase in its level is indicative of OS [175]. A correlation was noted between oocyte quality and the level of 8-OHdG in granulosa cells in patients undergoing IVF-ET and ICSI. A negative relationship was reported between fertilization rates and OS from granulosa cell resulting in increased level of 8-OHdG in both cumulus and granulosa cells. Similarly, a negative correlation was seen between 8-OHdG and production of good embryos [176]. Higher levels of 8-OHdG have been reported in patients with endometriosis when compared with other categories of infertility such as tubal factor, male factor and unknown. Bedaiway et al. conducted a study to find the correlation between the early embryonic development and the level of ROS in culture media on day 1 after insemination. High level of ROS associated with a low fertilization were reported in day 1, and low blastocyst rates on day 3, and lower pregnancy rates on day 5 day and high embryonic fragmentation rates with ICSI but not with IVF [120]. Addition of vitamins E and C, especially vitamin C was found to improve blastocyst development [177]. In another study, LPO levels were examined and elevated ROS level in follicular fluid did not result in oocyte fertilization supporting the hypothesis that higher LPO levels and lower level of TAC result in reduced fertilization rate due to reduced availability of antioxidants [113].


Fig. 4.3
Illustration of different internal and external factors that could have detrimental effects on sperm and oocytes
Guanine is more prone to oxidative damage. Oxidation of guanine leads to the formation of 7, 8-dihydro-8-oxoguanine (8-oxo-G), which is one of the major oxidative DNA adducts. It is known that 8-oxo-G is considered as a biomarker for OS in DNA [178]. In a study done by de Carvalho et al., [179] the histological levels of hydroxyl-2-deoxyguanosine were examined in biopsy specimens from healthy biopsy specimens including cervix, tubes, uterus, peritoneum, and topic endometrium in secretory phase and compared with benign gynecological biopsy tissues including hydrosalpinges, leiomyoma, adenomyosis and tubal cysts. Oxidative DNA damage was confirmed in these benign gynecologic disorders by the presence of high DNA damage as demonstrated by immunohistochemical staining of 8-hydroxy-2-deoxyguanosine. These authors concluded that hydrosalpinges, leiomyoma, and adenomyosis exhibit the highest amounts of oxidative DNA damage in the pelvic cavity [179].
Oocyte quality has been assessed by visualization of meiotic spindle and ROS levels. Aspirated follicular fluid from mature oocytes was examined in study patients with endometriosis, PCOS and tubal infertility. Following ovarian stimulation (long stimulation protocol), higher rates of meiotic spindle visualization were seen in mature oocytes and these were associated with lower ROS levels [180]. Oocytes with higher rates of spindle visualization yielded good quality (grade 1) embryos within each subgroup of patients. Disorganization of the meiotic spindles could result in chromosomal dispersion, failure of normal fertilization, chromosomal disjunction and karyotypic abnormalities in the embryo. Most studies on microtubular damage of oocytes have documented the sensitivity of the MII spindle to OS induced by cryopreservation. This increased buildup of ROS results in depolymerization of the microtubules, which are more prone to OS compared with variations in the chromosomal alignment. This may explain the poor oocyte quality and low fertilization and poor pregnancy outcomes both in vitro and in patients with endometriosis. These changes were validated using hydrogen peroxide as an exogenous inducer of ROS and also with the addition of 200 ng/mL of TNF-α. The effects of TNF-alpha inhibitors on microtubular structure and chromosomal alignments aimed at reducing alterations provoked by the cytokine and ROS should be examined cautiously. This is important when studying the potential benefits of combining such inhibitors with antioxidant(s) in an effort to enhance oocyte quality [181, 182].
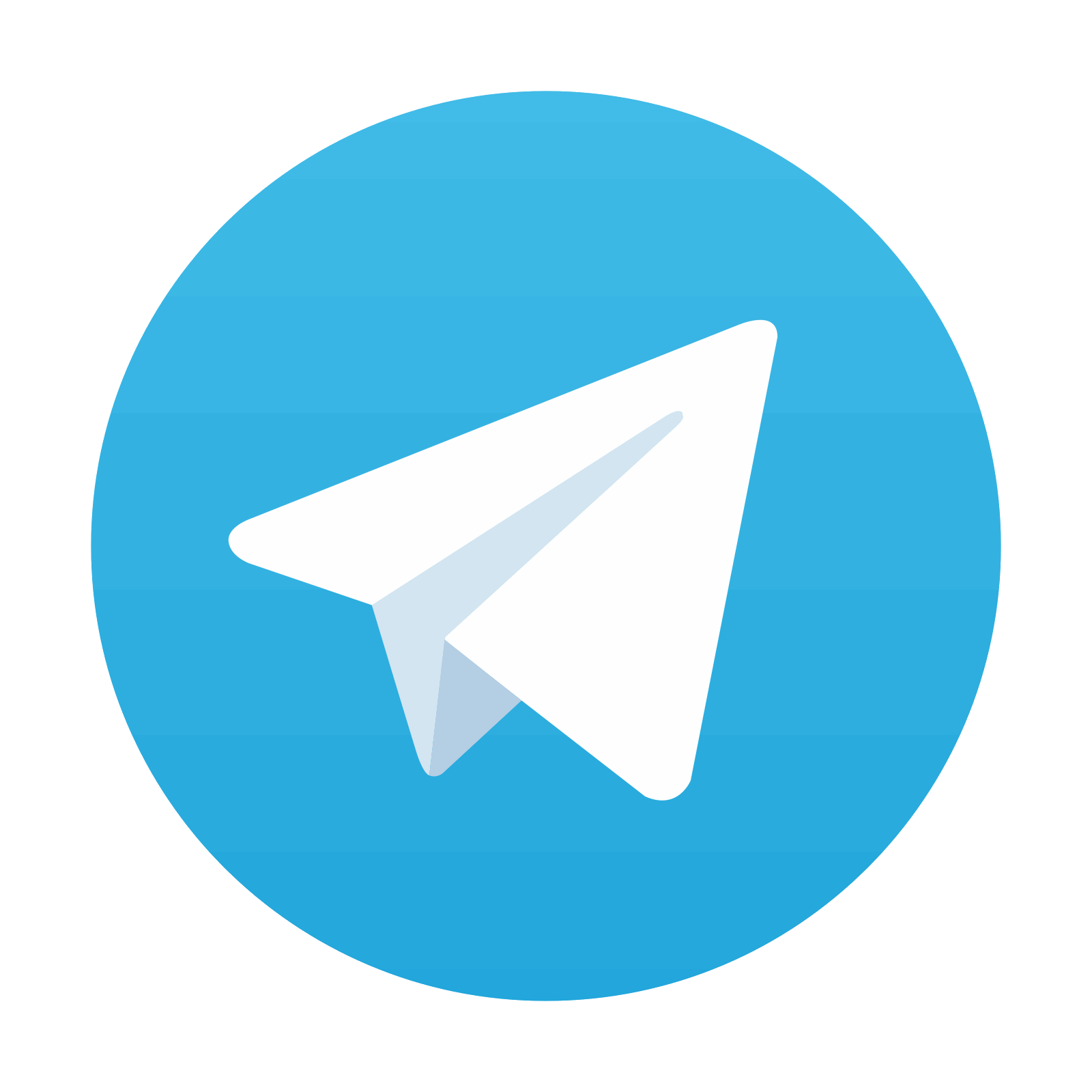
Stay updated, free articles. Join our Telegram channel

Full access? Get Clinical Tree
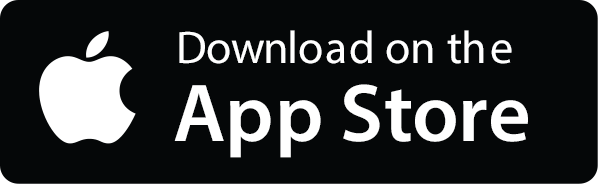
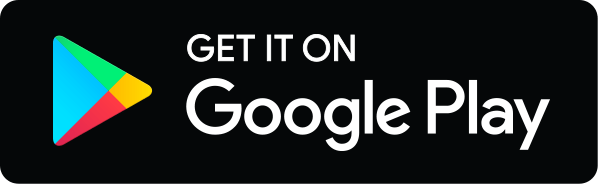