Background
The preterm birth syndrome (delivery before 37 weeks gestation) is a major contributor to the global burden of perinatal morbidity and death. The cause of preterm birth is complex, multifactorial, and likely dependent, at least in part, on the gestational age of the fetus. Intrauterine infection is frequent in preterm deliveries that occur at <32 weeks gestation; understanding how the fetus responds to proinflammatory insult will be an important step towards early preterm birth prevention. However, animal studies of infection and inflammation in prematurity commonly use older fetuses that possess comparatively mature immune systems.
Objective
Aiming to characterize acute fetal responses to microbial agonist at a clinically relevant gestation, we used 92-day-old fetuses (62% of term) to develop a chronically catheterized sheep model of very preterm pregnancy. We hypothesized that any acute fetal systemic inflammatory responses would be driven by signaling from the tissues exposed to Escherichia coli lipopolysaccharide that is introduced into the amniotic fluid.
Study Design
Eighteen ewes that were carrying a single fetus at 92 days of gestation had recovery surgery to place fetal tracheal, jugular, and intraamniotic catheters. Animals were recovered for 24 hours before being administered either intraamniotic E coli lipopolysaccharide (n = 9) or sterile saline solution (n = 9). Samples were collected for 48 hours before euthanasia and necroscopy. Fetal inflammatory responses were characterized by microarray analysis, quantitative polymerase chain reaction, and enzyme-linked immunosorbent assay.
Results
Intraamniotic lipopolysaccharide reached the distal trachea within 2 hours. Lipopolysaccharide increased tracheal fluid interleukin-8 within 2 hours and generated a robust inflammatory response that was characterized by interleukin-6 signaling pathway activation and up-regulation of cell proliferation but no increases in inflammatory mediator expression in cord blood RNA.
Conclusions
In very preterm sheep fetuses, lipopolysaccharide stimulates inflammation in the fetal lung and fetal skin and stimulates a systemic inflammatory response that is not generated by fetal blood cells. These data argue for amniotic fluid-exposed tissues that play a key role in driving acute fetal and intrauterine inflammatory responses.
Preterm birth (PTB; delivery before 37 weeks of completed gestation) is a multi-origin syndrome that results in an excess of 1 million perinatal deaths each year. The preterm deliveries at highest risk of death and significant disability are those occurring at ≤32 weeks of gestation, most commonly in association with intrauterine infection and chorioamnionitis. Goldenberg et al have suggested that 25-40% of PTBs are due to infection, noting that the true rate, in fact, may be higher because of spuriously negative microbial culture results. An increased risk of adverse neonatal outcomes is also associated independently with an elevated cord blood plasma interleukin (IL)-6 level (>11 pg/mL), termed the fetal inflammatory response syndrome .
In the presence of microbial agonist, innate immune receptors (including the TOLL-, NOD-, and RIG-I–like receptor families) in fetal and maternal tissues drive the expression and release of proinflammatory mediators that include IL-1α/β, -6, and -8 and tumor necrosis factor (TNF)-α. Intrauterine inflammation is considered key to both the premature activation of the pathways of parturition and a number of the diseases (eg, cerebral palsy) that are associated with prematurity. As such, interventions that resolve both intrauterine infection and inflammation may prevent microbial-associated PTB and its sequelae. A number of investigators have studied responses to intrauterine infection and inflammation in primate, sheep, and rodent models of pregnancy. However, much remains to be understood with regards the tissues responsible for driving intrauterine inflammation. Of note, a significant number of previous studies in this field have been undertaken in late-gestation pregnancies.
We aimed to catheterize fetal sheep chronically to characterize the acute intrauterine inflammatory response to Escherichia coli lipopolysaccharide in very preterm fetuses. Noting that lipopolysaccharide does not cross cell-cell barriers, we hypothesized that any acute fetal systemic inflammatory responses would be driven by signaling from the tissues that are exposed to the amniotic fluid (AF).
Materials and Methods
Animals
Animal studies were approved by The University of Western Australia’s Animal Ethics Committee (approval RA/3/100/1289). Eighteen date-mated ewes with singleton fetuses at 92 days gestational age had aseptic recovery surgery to place catheters into the fetal jugular vein and trachea and 2 catheters into the AF, as described previously. After a 24-hour recovery, animals were assigned at random to receive either (1) 10 mg E coli lipopolysaccharide (O55:B5; Sigma Aldrich, St. Louis, MO) in 2-mL sterile saline solution via intraamniotic catheter (n = 9) or (2) 2-mL sterile saline solution via intraamniotic catheter (n = 9).
AF, tracheal fluid (TF), and fetal plasma (FP) were sampled serially immediately before and 2, 4, 8, 12, 24, and 48 hours after treatment administration. Animals were euthanized with an intravenous bolus (100 mg/kg) of pentobarbitone at 48 hours. Fetal cord blood was collected for blood chemistry analysis and for RNA extraction with the use of PAXgene Blood RNA Tubes (PreAnalytiX GmbH, Hombrechtikon, Switzerland) in accordance with manufacturer’s instructions. Fetal tissues were snap frozen in liquid nitrogen for subsequent analysis; 6-9 animals were analyzed per group.
Tissue RNA preparation
RNA was isolated from fetal tissues (internal groin skin, spleen, lung right lower lobe, and frontal cortex) and mechanically disrupted with a Precellys homogenizer (Bertin Technologies, Rockville, MD) in 1-mL TRIzol (Life Technologies, Carlsbad, CA) in accordance with manufacturers’ instructions. RNA was treated with Turbo-DNase (Life Technologies) as previously reported and quantified with a QUBIT fluorometer (Life Technologies).
Quantitative polymerase chain reaction (qPCR)
Sheep-specific probes and PCR primer sets were used to perform qPCR reactions for IL-1β, -6, and -8, TNF-α, monocyte chemoattractant protein (MCP)-2, IL-10 and IL-13 with an EXPRESS One-Step SuperScript qRT-PCR Kit (all Life Technologies) that contained a 125-ng RNA template in a final volume of 20 μL, according to the manufacturer’s instructions. Cycling conditions and primer/probe sets were as described previously. Quantitation cycle (Cq) values were normalized against 18s ribosomal RNA and expressed as fold changes relative to saline solution control values.
Limulus assay
The concentration of lipopolysaccharide in the AF and TF was determined with Pierce LAL Chromogenic Endotoxin Quantitation Kits (Life Technologies) in accordance with the manufacturer’s instructions. Plates were read at a wavelength of 405 nm on an Anthos 2010 Standard Plus plate reader (Biochrom Ltd, Cambridge, UK).
Enzyme-linked immunosorbent assay (ELISA)
Quantification of IL-6 and -8 protein concentrations in AF, TF, and FP samples were performed with the use of in-house ELISAs, as previously described. TNF-α and MCP-1 levels were assayed with ELISA VetSets (Kingfisher Biotech, Saint Paul, MN) according to the manufacturer’s instructions with 1 modification: coated wells were incubated with samples overnight at 4°C. For IL-8 quantification, samples were diluted 1:10 in assay buffer. Plates were read at a wavelength of 450 nm on an Anthos 2010 Standard Plus plate reader (Biochrom Ltd). For each experiment, samples and standards were assayed in duplicate.
Microarray
Comparative transcriptomic analysis (Ovine Gene 1.0 ST 8 x 15K Array; Affymetrix, Santa Clara, CA) was performed on arterial cord blood RNA that had been collected at necroscopy from 4 lipopolysaccharide-exposed and 4 saline solution–exposed fetuses that were selected at random. All RNA samples underwent quality control assessment for purity and integrity and had an RNA integrity number value of 6.5-8.8. For each sample, 100 ng of total RNA was processed with the use of a WTPlus Kit (Affymetrix) according to the manufacturer’s instructions. Fragmented, labelled single-stranded DNA was hybridized in a GeneChip hybridization oven 640 at a final concentration of 23 ng/μL with a GeneChip Hybridization, Wash and Stain Kit (both Affymetrix) in accordance with manufacturer’s instructions. Arrays were washed and stained on a GeneChip Fluidics Station 450 and scanned on a GeneChip 3000 7G+ scanner, and GeneChip Command Console Software was used (all Affymetrix), according to the manufacturer’s instructions. Microarray data were preprocessed with the robust multi-array average algorithm, background correction, quartile normalization, and gene-level probe set summation. Genes that were expressed differentially between the 2 groups were identified by Significance Analysis of Microarrays (SAM) analysis with a false discovery rate of 0.1 with the use of BRBArrayTools (version 4.4) software (developed by Dr Richard Simon and BRB-ArrayTools development team). Gene networks were generated by Ingenuity Pathway Analysis software (Qiagen, Redwood City, CA).
qPCR reactions to validate microarray findings were performed on samples that were submitted for microarray analysis with the Roche Universal Probe Library (Roche Diagnostics Australia Pty Ltd, Castle Hill, NSW, Australia) in accordance with the manufacturer’s instructions. The 4 most differentially up-regulated targets by SAM analysis were selected for analysis: CCNB1 (Right Primer: cctctggaaaaggctcctg ; Left Primer: ccttaacaggctcgggttc ; Universal Probe: 36 Amplicon Size [nucleotides, nt]: 73), MT2A (Right Primer: ggatcccaactgctcctg ; Left Primer: gcgcacttgcaatctttg ; Universal Probe: 92; Amplicon Size [nt]: 78), TCN1 (Right Primer: ttgttgggattaagagtcaaagg ; Left Primer: ttatgtcttctttgatttgtccactc ; Universal Probe: 150; Amplicon Size [nt]: 63), and TOP2A (Right Primer: gaccattatcaatttggctcaga ; Left Primer: ggctgcaaaaggttcagatt ; Universal Probe: 44; Amplicon Size [nt]: 60). Cq values were normalized against glyceraldehyde-3-phosphate dehydrogenase (Right Primer: ggcctccaaggagtaaggtc ; Left Primer: tctcttcctctcgtgctcct ; Universal Probe: 23; Amplicon Size [nt]: 60) and β-Actin (Right Primer: ggacggaccctcacacatac ; Left Primer: gtgagaagcctgccaacg ; Universal Probe: 70; Amplicon Size [nt]: 61).
Statistical analysis
All qPCR values are mean ± standard deviation. Statistical tests were performed on delta Cq values, and data are reported as fold change vs control. All ELISA values are median ± interquartile range. Statistical tests were performed with SPSS Statistics for Windows (version 20.0; IBM Corporation, Armonk, NY.). Variance and distribution were assessed for normality. Mean differences between normally distributed data were tested for significance with 1-way analysis of variance with a probability value of .05. Tukey’s test was used to perform multiple post-hoc comparisons. Between-group differences in nonparametric data were tested for significance with Kruskal-Wallis 1-way analysis of variance, with a probability value of .05 accepted as significant. Rank-sum tests were used to perform multiple post-hoc comparisons that were performed with the probability value corrected for n multiple comparisons, where appropriate.
Results
Clinical observations
All surgeries were successfully completed, and there were no fetal deaths before scheduled euthanasia. No significant differences were identified in birthweight, arterial cord blood pH, pO 2 , pCO 2, lactate, glucose, or white blood cell counts (total or differential) between treatment and control groups. None of the study animals had meconium, and there was no increase in the presence of fetal edema or ascites as a result of lipopolysaccharide exposure. Compared with saline solution control, the concentration of γ-glutamyltransferase (a marker of oxidative stress) in fetal cord blood plasma that was collected at euthanasia was significantly increased ( P < .05) at necroscopy in animals that were exposed to lipopolysaccharide for 48 hours ( Table 1 ).
Group | Delivery weight, g | Male fetuses, n | Arterial fetal arterial cord blood | |||||||
---|---|---|---|---|---|---|---|---|---|---|
pH | pO 2 , mmHg | pCO 2 , mmHg | Total white blood cell count, 10 9 /L | Aspartate aminotransferase, U/L | γ-Glutamyl transpeptidase, U/L | Glutamate dehydrogenase, U/L | Total bilirubin, μmol/L | |||
Saline solution control (n = 9) | 956.0 ± 164.0 | 6 | 7.15 ± 0.04 | 14.23 ± 3.2 | 85.8 ± 8.0 | 0.93 ± 0.4 | 16.8 ± 3.8 | 8.4 ± 3.3 | 2.6 ± 1.6 | 10.1 ± 4.0 |
Lipopolysaccharide (endotoxin) from Escherichia coli (n = 9) | 850 ± 117.0 | 6 | 7.13 ± 0.04 | 13.0 ± 4.5 | 91.0 ± 6.7 | 1.1 ± 0.8 | 16.6 ± 4.9 | 15.4 ± 7.2 a | 5.5 ± 7.0 | 12.3 ± 2.6 |
Endotoxin quantification
For animals that were randomized to lipopolysaccharide exposure, the endotoxin levels in both AF and TF were below the assay limit of detection (0.1 endotoxin units (EU)/mL) immediately before lipopolysaccharide administration. Endotoxin concentrations in the AF and TF exceeded 1 EU/mL by 2 hours and remained >1 EU/mL at all subsequent time points to 48 hours.
ELISA
All values are median (25th-75th percentile). Statistical comparisons are relative to saline solution control samples that were collected contemporaneously at matched time points. There were no significant increases in TNF-α concentrations in AF, TF, or FP at any time point (data not shown). IL-6 concentrations were significantly increased in AF from lipopolysaccharide-exposed fetuses at 12, 24, and 48 hours, relative to saline solution controls. IL-6 concentrations were significantly increased in TF from lipopolysaccharide-exposed fetuses at 24 and 48 hours, relative to saline solution control. IL-6 concentrations were significantly increased in FP from lipopolysaccharide-exposed fetuses at 12 hours, relative to saline solution control ( Table 2 ).
Hour | Interleukin-6, pg/mL (25th-75th percentile) | |||
---|---|---|---|---|
Treatment | Amniotic fluid | Tracheal fluid | Fetal plasma | |
0 | Saline solution | 0.0 (0.0–21.8) | 84.1 (29.3–111.3) | 0.0 (0.0–18.1) |
Lipopolysaccharide | 0.0 (0.0–103.0) | 107.5 (50.7–180.6) | 17.8 (0.0–64.9) | |
2 | Saline solution | 0.0 (0.0–65.4) | 79.7 (67.5–141.2) | 99.0 (0.0–217.3) |
Lipopolysaccharide | 0.0 (0.0–71.5) | 47.8 (17.3–109.3) | 3.8 (0.0–33.0) | |
4 | Saline solution | 0.0 (0.0–3.4) | 22.0 (5.3–139.6) | 15.3 (0.0–132.1) |
Lipopolysaccharide | 42.5 (0.0–120.4) | 136.0 (32.9–174.8) | 165.7 (0.0–219.2) | |
8 | Saline solution | 0.0 (0.0–89.7) | 41.1 (19.0–88.0) | 26.2 (0.0–95.3) |
Lipopolysaccharide | 89.6 (47.8–165.0) | 158.2 (127.4–201.3) | 256.5 (26.6–757.7) | |
12 | Saline solution | 0.0 (0.0–25.4) | 98.1 (25.0–139.0) | 0.0 (0.0–34.0) |
Lipopolysaccharide | 102.7 (66.4–421.3) a | 129.5 (120.8–193.4) | 1159.7 (300.1–1342.3) a | |
24 | Saline solution | 0.0 (0.0–147.0) | 50.0 (0.0–134.4) | 95.3 (56.8–314.7) |
Lipopolysaccharide | 186.8 (145.8–619.0) a | 334.7 (225.5–406.9) a | 69.4 (44.2–405.4) | |
48 | Saline solution | 0.0 (0.0–37.4) | 100.4 (55.2–126.6) | 0.0 (0.0–88.88) |
Lipopolysaccharide | 406.3 (343.5–601.0) a | 412.2 (170.0–527.8) a | 254.3 (53.4–431.2) |
IL-8 concentrations were significantly increased in AF from lipopolysaccharide-exposed fetuses at 4, 8, 12, 24, and 48 hours, relative to saline solution control. IL-8 concentrations were significantly increased in TF from lipopolysaccharide-exposed fetuses at 2, 4, 12, 24, and 48 hours, relative to saline solution control. IL-8 concentration was significantly increased in FP from lipopolysaccharide-exposed fetuses at 12 hours, relative to saline solution control ( Table 3 ).
Hour | Interleukin-8, pg/mL (25th-75th percentile) | |||
---|---|---|---|---|
Treatment | Amniotic fluid | Tracheal fluid | Fetal plasma | |
0 | Saline solution | 464.0 (223.0–532.9) | 337.7 (29.8–728.66) | 1007 (764.8–1175.8) |
Lipopolysaccharide | 275.7 (175.1–346.1) | 362.6 (326.2–1030.5) | 1412.9 (1051.3–1858.1) | |
2 | Saline solution | 217.6 (104.5–606.5) | 220.9 (107.0–569.7) | 952.4 (678.7–1498.3) |
Lipopolysaccharide | 432.1 (192.5–812.3) | 1012.0 (705.2–1138.3) a | 1867.5 (1657.7–1965.6) | |
4 | Saline solution | 169.6 (0.0–524.6) | 418.8 (69.2–663.3) | 1004.6 (874.3–1161.0) |
Lipopolysaccharide | 1910.1 (1702.0–2529.3) a | 1904.8 (1691.5–2029.4) a | 1246.2 (1118.6–1738.2) | |
8 | Saline solution | 315.3 (253.5–795.8) | 472.7 (184.9–802.2) | 1229.2 (628.0–1706.6) |
Lipopolysaccharide | 4976.2 (4283.6–6148.7) a | 2425.9 (2111.3–2551.2) a | 1142.4 (512.9–1827.0) | |
12 | Saline solution | 257.9 (68.0–475.3) | 507.4 (212.9–989.3) | 890.8 (427.8–1246.2) |
Lipopolysaccharide | 9389.4 (8574.1–12020.6) a | 3265.8 (2665.2–5105.9) a | 2119.4 (1512.0–2313.3) a | |
24 | Saline solution | 303.2 (150.8–728.4) | 404.2 (133.2–1057.1) | 796.5 (634.3–1140.9) |
Lipopolysaccharide | 22430.5 (19787.1–27368.7) a | 13374.4 (11781.8–13795.3) a | 1409.3 (992.6–1731.0) | |
48 | Saline solution | 764.8 (673.2–2440.2) | 785.7 (499.6–1301.4) | — |
Lipopolysaccharide | 73963.9 (55876.0–89334.6) a | 43904.8 (16867.8–101299.6) a | — |
MCP-1 concentrations were significantly increased in AF from lipopolysaccharide-exposed fetuses at 4, 8, 12, 24, and 48 hours, relative to saline solution control. MCP-1 concentrations were significantly increased in TF from lipopolysaccharide-exposed fetuses at 8, 12, 24, and 48 hours, relative to saline solution control. MCP-1 concentrations were significantly increased in FP from lipopolysaccharide-exposed fetuses at 4, 8, 12, 24, and 48 hours, relative to saline solution control ( Table 4 ).
Hour | Monocyte chemoattractant protein-1, pg/mL (25th-75th percentile) | |||
---|---|---|---|---|
Treatment | Amniotic fluid | Tracheal fluid | Fetal plasma | |
0 | Saline solution | 41.8 (21.7–59.6) | 12.6 (6.3–48.9) | 4.0 (0.0–26.0) |
Lipopolysaccharide | 95.6 (35.2–122.5) | 29.4 (27.5–50.5) | 12.0 (7.5–15.0) | |
2 | Saline solution | 41.8 (26.5–55.3) | 20.0 (4.2–38.5) | 8.0 (5.0–29.0) |
Lipopolysaccharide | 77.7 (41.8–97.9) | 79.9 (69.9–85.0) | 31.0 (22.5–32.0) | |
4 | Saline solution | 37.4 (19.4–57.7) | 24.0 (2.1–106.5) | 4.0 (3.0–24.0) |
Lipopolysaccharide | 91.1 (79.6–120.3) a | 75.7 (63.3–123.8) | 99.8 (83.9–99.8) a | |
8 | Saline solution | 72.6 (37.4–106.8) | 33.6 (11.0–48.9) | 8.0 (2.0–25.0) |
Lipopolysaccharide | 127.0 (117.0–151.6) a | 109.3 (74.7–132.4) a | 133.7 (108.8–142.3) a | |
12 | Saline solution | 59.8 (46.3–88.2) | 40.0 (10.5–50.0) | 8.0 (0.5–18.5) |
Lipopolysaccharide | 158.4 (111.3–207.7) a | 145.8 (108.0–148.5) a | 147.7 (79.9–153.7) a | |
24 | Saline solution | 68.7 (64.1–84.4) | 16.8 (12.2–78.5) | 15.0 (5.0–20.5) |
Lipopolysaccharide | 234.6 (187.5–241.3) a | 168.1 (147.5–197.6) a | 97.8 (67.4–127.8) a | |
48 | Saline solution | 95.6 (71.0–132.5) | 92.5 (83.8–117.7) | 4.0 (0.0–12.0) |
Lipopolysaccharide | 257.0 (247.6–279.4) a | 190.3 (146.3–248.0) a | 33.9 (30.9–63.9) a |
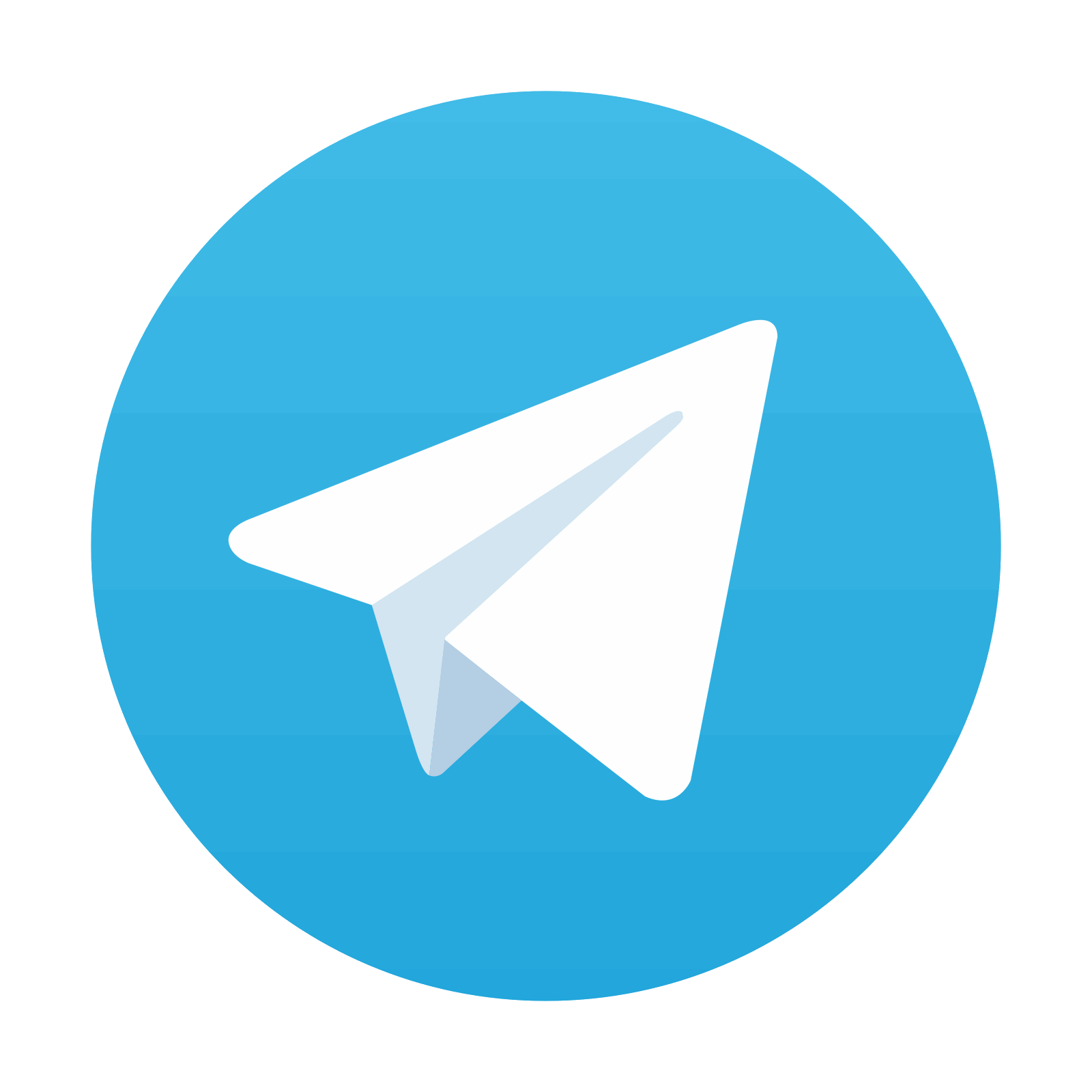
Stay updated, free articles. Join our Telegram channel

Full access? Get Clinical Tree
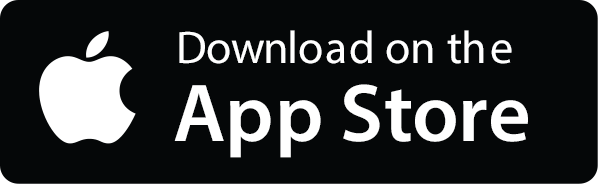
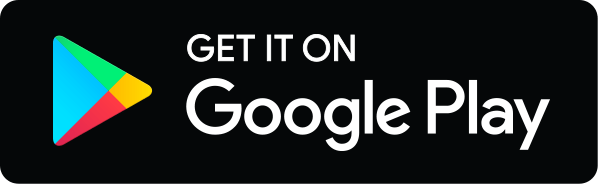
