Fig. 1
Age distribution of children with acute hematogenous osteomyelitis (AHO) and septic arthritis (SA). White bars represent AHO, and dark bars represent SA
A decrease in the incidence of osteomyelitis and septic arthritis caused by HiB was reported in 1999 in a large retrospective series of 851 cases from Canada spanning the pre- and post-HiB vaccination effort. This shift was most dramatic in the cases of septic arthritis, dropping from 41 % of culture-positive cases caused by HiB prior to the introduction of the vaccine to no reported cases after 1991 (Howard et al. 1999).
More recently, the emergence of antibiotic-resistant strains of bacteria, most importantly in the form of methicillin-resistant staphylococcus aureus (MRSA) , has presented new challenges to clinicians (Young et al. 2011). In contrast to the above reports, in 2008 Gafur et al. reported a 2.8-fold increase in the incidence of acute and subacute hematogenous osteomyelitis in the pediatric population treated at the University of Texas Southwestern between 1982 and 2002 (Gafur et al. 2008). This increase was not noted with septic arthritis. In addition, the authors noted a sharp decline in the isolation of HiB as the causative agent, from 22.6 % to 1 % over the same time period. In the discussion of their results, one potential cause of the noted increase of osteomyelitis was suggested to be the emergence of MRSA as a causative agent.
The influence of MRSA on the recent epidemiological changes is also supported by a report by Arnold et al. from 2006, where the authors reported a more than twofold increase in the incidence of acute hematogenous osteomyelitis and septic arthritis from 2000 to 2004 (Arnold et al. 2006). In that study, carried out at a single children’s hospital in Memphis, Tennessee, MRSA increased as the causative agent from 4 % to 40 % of cases and explained the overall increase in osteoarticular infections. The authors also noted a perceived increase in the severity of many infections upon presentation, as well as an increase in the rate and severity of the complications, such as deep venous thrombosis (DVT) and septic pulmonary emboli, in patients with MRSA osteoarticular infections in comparison to other causative pathogens.
It appears that after noting a significant decrease in incidence of acute and subacute hematogenous osteomyelitis in the latter half of the twentieth century, at least some centers are noting a rebound in frequency, in part due to the emergence of community-acquired MRSA (CA-MRSA) (Copley 2009). This trend may be less apparent with septic arthritis. It is also worth noting that it can be difficult to separate out the well-documented increasing use of MRI in the diagnosis of osteomyelitis from a true increase in the incidence of the disease.
In the industrialized world, chronic hematogenous osteomyelitis, defined as osteomyelitis present for greater than 3 months, has become a rare condition. However, in the developing world, the prevalence of musculoskeletal impairment due to infection has been estimated at roughly 3 %, as evidenced by a recent study performed in Rwanda (Atijosan et al. 2008). Jones et al. estimated that if this incidence is assumed to be similar among similarly developed countries, “in these countries alone approximately 12 million children are affected (Jones et al. 2011).” This represents a huge global burden of disease that uses a significant amount of limited resources in already stressed health systems in developing countries. In 2000, Bickler and Sanno-Duanda reported that 7.8 % of pediatric surgical admissions and 15.4 % of total inpatient days in Banjul, Gambia, were due to osteomyelitis (Bickler and Sanno-Duanda 2000). This exists in stark contrast to reports from the industrialized world where, in Scotland in 2001, Blyth et al. noted that “(chronic) hematogenous osteomyelitis in children in this area is becoming a rare disease” (Blyth et al. 2001).
Septic arthritis has an increased incidence in younger children, with 58 % of cases in one large study, present in those children less than 2 years of age (Goergens et al. 2005; Fig. 1). Pediatric septic arthritis has not seen the perceived increase in incidence that some authors have reported with osteomyelitis over the past decades. However, despite methicillin-sensitive Staphylococcus aureus remaining the most commonly identified pathogen in septic arthritis, there is an increasing reported incidence of CA-MRSA as the causative agent (Young et al. 2011; Vander Have et al. 2009). Mirroring the reports on osteomyelitis, an increase in the rate of complicated musculoskeletal infections involving both the bone and joint has been noted and is an important consideration in the evaluation and treatment of suspected septic arthritis (Copley 2009).
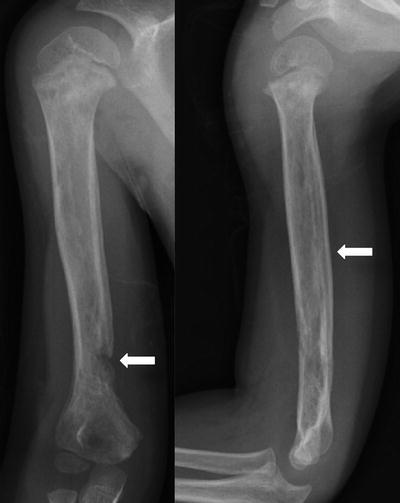
Fig. 2
Periosteal reaction and bony destruction involving the humerus seen in a combined osteoarticular infection in a 4-year-old boy
There has been a reported dramatic decrease in the proportion of septic arthritis cases caused by Haemophilus influenzae type B after the introduction of the HiB vaccine in the early 1990s (Howard et al. 1999). More recently, there has been recognition that Kingella kingae may play a very important role in pediatric septic arthritis and osteoarticular infection. In children under the age of four, K. kingae may be the most common causative pathogen (Yagupsky et al. 2011; Ceroni et al. 2010).
Osteomyelitis
Pathoanatomy
Osteomyelitis can be categorized as acute hematogenous (AHO) , subacute (SO) in those patients presenting after 2 weeks of symptoms, or chronic (CO) in those patients with symptoms for greater than 3 months (Craigen et al. 1992; Beckles et al. 2010). The direct inoculation of bone, as occurs with open fractures, as well as hematogenous spread to an area of closed bony injury, is a well-recognized mechanism for developing an infection, and many authors believe that AHO is in many cases related to minor trauma or subtle fractures. Like septic arthritis, AHO has an increased incidence in young children, with 40 % of cases in one large study present in those children less than 3 years of age, with a gender ratio of nearly 2:1 of boys to girls affected (Goergens et al. 2005; Fig. 1).
The majority of cases of osteomyelitis in the industrialized world are AHO and SO , and the pathoanatomy is closely related to the vascular anatomy of the pediatric bone. In the metaphyseal region of the long bones of children, the nutrient artery perforates the metaphysis and terminates near the physis in a series of dilated, low-flow venous sinusoids, which, after making a sharp turn, become a venous capillary network in the medullary bone. The numerous dilated metaphyseal venous capillaries have been described as “blood lakes.” The low-flow state of this anatomical region leads to the increased susceptibility of bacterial infection transferred from the blood, as the organism “finds its ideal medium for development (Trueta 1959).” As the infection is initiated, thrombosis of the venous system is the initial vascular event, followed by thrombosis of the nutrient artery. Both thrombotic events further lead to an ideal milieu for the growth of bacteria in the pediatric metaphysis.
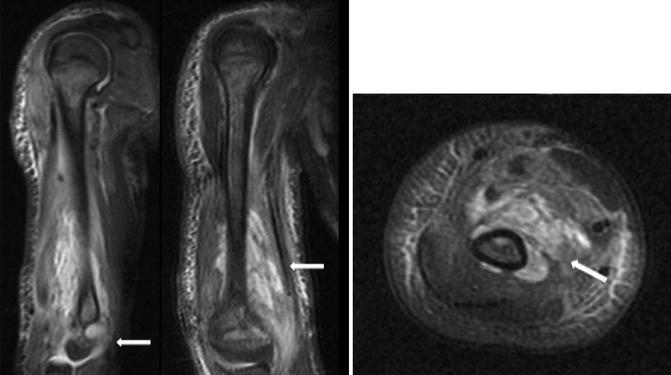
Fig. 3
MRI of the humerus with a severe osteoarticular infection
The vascular congestion and edema that occurs can lead to cortical breakthrough and elevation of the periosteum, which may then be followed by purulence in a similar tract down the diaphysis (Trueta 1959). The periosteal reaction caused by the infection then forms and can typically be seen on plain radiographs within several days. Over time, the new bone formation can lead to the formation of an involucrum, seen in chronic osteomyelitis. A sequestrum may also develop if avascular bone becomes walled off from the surrounding bone. The epiphysis garners its blood supply from a separate artery, and this supports the postulated vascular etiology of disease, in that the epiphysis and metaphysis are rarely both involved with AHO. Therefore, the physis and epiphysis are classically protected from the majority of osteomyelitis infectious processes.
In cases of osteomyelitis following an open fracture, the bone is directly inoculated, and the site of infection and subsequent development of infection correspond to the fracture site which is influenced by varying degrees of avascularity of the affected bone due to trauma. For closed fractures that are seeded by the bloodstream and develop osteomyelitis, a similar mechanism involving localized slowing of blood flow and/or formation of hematoma in the region is postulated to lead to infection via an analogous process to atraumatic metaphyseal osteomyelitis.
Assessment of Disorder
Children with acute and subacute osteomyelitis of the upper extremity classically present with fever, pain, and dysfunction of the affected extremity, but may exhibit a combination of only some or all of these findings. Localization of the affected region is typically possible by a thorough physical exam, as the patient can be tender to palpation, may exhibit swelling and/or erythema in the adjacent soft tissues, and/or may demonstrate pain with range of motion and/or weight bearing. At times, in particular with early acute hematogenous osteomyelitis, these findings may be subtle, without any visible changes in the surrounding soft tissues. A high index of suspicion is needed in particular in those children who are too young to adequately communicate with the physician, which is the demographic most commonly affected. This can be especially true in osteomyelitis of the upper extremity, as many times parents or caregivers note a reluctance to bear weight when the lower extremity is affected as the initial sign of pathology. This sign may be absent or delayed in the recognition of pathology in the upper extremity.
While always in the differential of musculoskeletal pain in children, the presence of osteomyelitis may still be missed by those treating physicians commonly involved prior to orthopedic consultation. Treatment for presumed cellulitis or an abscess without typical resolution can last for days before the true diagnosis of osteomyelitis is recognized and can compromise the outcome.
The initial imaging modality for suspected osteomyelitis is high-quality plain radiographs, and in addition to the bony findings, soft tissue findings should be assessed for. Evidence of a joint effusion and/or soft tissue swelling with acute osteomyelitis is important, in particular, as bony changes may not yet have occurred. When present, osseous findings can include bony destruction and/or periosteal reaction (Fig. 2).
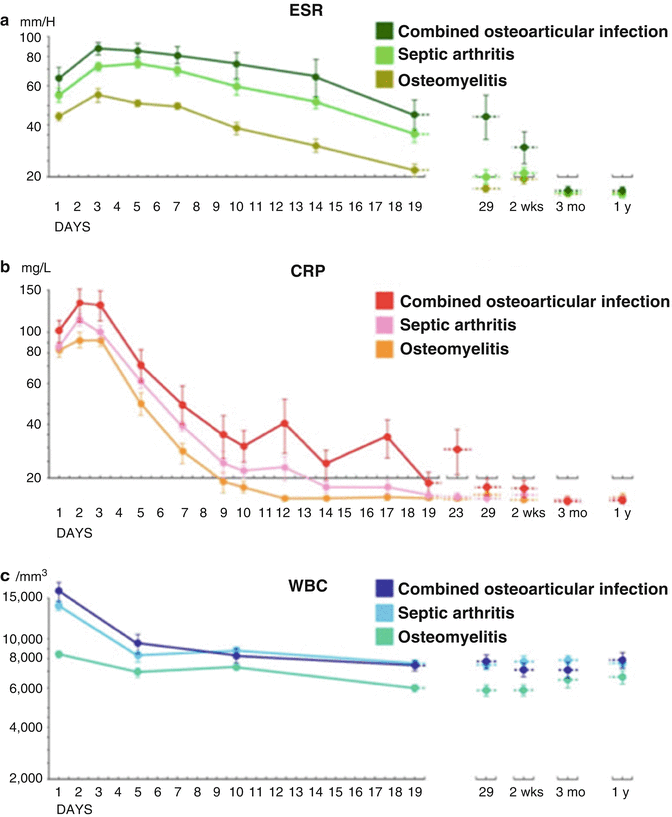
Fig. 4
Time course of laboratory values in osteomyelitis, septic arthritis, and combined osteoarticular infection
MRI with and without contrast has emerged as the most sensitive and specific imaging modality in the diagnosis of osteomyelitis in children and has largely supplanted bone scintigraphy. In 1995, Mazur et al. reported a sensitivity of 0.97 and specificity of 0.92 with contrast-enhanced MRI (Mazur et al. 1995). In a subset of their cohort, bone scintigraphy was also obtained, with a sensitivity of 0.64 and specificity of 0.71. MRI also can aid in surgical planning, as it more precisely defines the anatomical extent of the infection (Fig. 3).
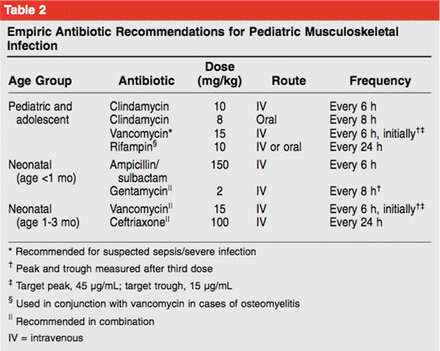
Fig. 5
MRI of the humerus with a severe osteoarticular infection
Bone scintigraphy may still be advantageous in suspected cases of osteomyelitis where the physical exam does not localize the process or in cases where multifocal osteomyelitis is suspected. In addition, ultrasound has been reported to be a viable mechanism to detect osteomyelitis prior to any plain radiographic findings being present (Riebel et al. 1996; Mah et al. 1994). However, where MRI is readily available, it has largely supplanted the use of both ultrasound and bone scintigraphy due to the accuracy and anatomic detail it affords. In addition, it is one of the first pieces of information that guides treatment.
Laboratory studies that should be routinely obtained during the initial evaluation of osteomyelitis include complete blood cell count with differential (CBC), erythrocyte sedimentation rate (ESR), and C-reactive protein (CRP). An essential aspect in the interpretation of the laboratory studies is the consistent finding that the CBC can be normal in up to 80 % of children with osteomyelitis, and therefore the laboratory workup should be focused on the ESR and CRP (Pääkkönen et al. 2009). Multiple reports have noted the increase in both ESR and CRP in a very high percentage of children with acute osteomyelitis upon admission to the hospital (Pääkkönen et al. 2009; Khachatourians et al. 2003; Roine et al. 1995). In 1995, Unkila-Kallio et al. reported elevations of ESR and CRP upon admission in bacteriologically confirmed AHO in 92 % and 98 % of children, respectively. The mean values of the ESR and CRP were 45 mm/h and 71 mg/L, respectively. Similar rates of sensitivity and specificity have been reported by other authors more recently, with rates nearing 100 % when ESR and CRP are analyzed together (Pääkkönen et al. 2009). The CRP and ESR can therefore be very useful to the clinician for several reasons, including ruling out osteomyelitis, tracking the response to treatment, and making the decision to stop antibiotic therapy (Fig. 4).
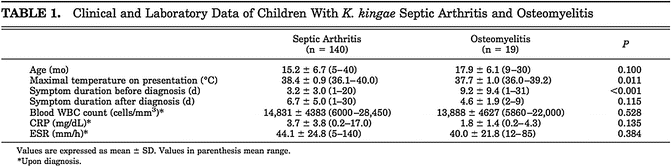
Fig. 6
Clinical and laboratory data of children with K. Kingae infection
Blood cultures should also be obtained, and, when possible, a tissue sample through aspiration should be obtained prior to the administration of antibiotics in order to facilitate accurate treatment of the causative organism. While these tests are standard in the evaluation of pediatric osteomyelitis, it is important to note that a negative culture result from the blood, aspiration, or direct biopsy is not uncommon. Arnold et al. noted a 49 % positive culture result (from blood, bone, or joint aspirate) in their series of 89 patients with osteomyelitis, which is similar to the report from Georgens et al. where they noted a rate of 45 % (Goergens et al. 2005; Arnold et al. 2006). For complex osteoarticular infections, however, the rate of positive blood cultures was 82 % (Goergens et al. 2005; Arnold et al. 2006). Persistently positive blood cultures (3 or more days) have been associated with MRSA infection (Arnold et al. 2006).
The most common causative pathogens in the postvaccination era are overwhelmingly varieties of Staph aureus (MSSA, MRSA, and coagulase negative), but other pathogens including Kingella kingae, Salmonella group D, Enterobacter, and Pseudomonas species have all been reported (Copley et al. 2013).
Kingella osteoarticular infection and its detection deserve a special note as an emerging pathogen in pediatric osteoarticular infections. In children less than 4 years of age, osteoarticular infection with Kingella has increasingly been recognized as an important and frequent pathogen – possibly the most common bacteria responsible for the infection in this age group. The clinical presentation and laboratory investigations can be mild and normal, respectively. Furthermore, Kingella has historically been a difficult pathogen to culture on solid medium, even when suspected. Newer polymerase chain reaction (PCR) techniques have been developed to allow for more accurate identification of this pathogen and have been applied clinically at some centers. In 2010, Ceroni et al. reported that 82.1 % of cases of pediatric osteoarticular infections in children less than 4 years of age were caused by Kingella, as determined by PCR identification (Ceroni et al. 2010). In each of these cases, traditional methods of staining and cultures were all negative. The authors’ findings led them to state, “Kingella kingae is an emerging pathogen that may be recognized as the most common bacteria responsible for osteoarticular infections (OAI) in young children.” Laboratory and specimen collection protocols that routinely attempt identification of this fastidious and common organism may alter the current treatment algorithms at many institutions (Yagupsky et al. 2011).
While rare in comparison to hematogenous osteomyelitis, direct inoculation of bone occurring as a result of open fractures, soft tissue trauma, or surgical interventions can lead to the development of osteomyelitis. In addition, closed fractures or minor injuries to the upper extremity may predispose the affected bone to the vascular stasis that underlies the development of spontaneous hematogenous osteomyelitis. Appropriate urgent management of open fractures with debridement and irrigation, parenteral antibiotics, and modern surgical care has led to a decreased incidence of osteomyelitis associated with open fractures in children.
A special note should also be made about chronic recurrent multifocal osteomyelitis (CRMO) – a rare, noninfectious condition characterized by the presence of bony lytic lesions that can affect one to multiple locations around the body. In isolation, lesions may mimic AHO in regard to their presentation (pain, swelling, redness), but typically multiple sites are affected. The differentiation of CRMO from AHO early in the course is important, as the recommended treatments for each disorder are significantly different.
Treatment Options
Nonoperative
In AHO or SHO, after the above workup is complete (laboratory, radiographs, and MRI), the decisions for bone aspiration and/or the need for surgical treatment are made. In most cases, bone aspiration is recommended to guide the selection of antibiotics. For children with uncomplicated acute or subacute hematogenous osteomyelitis, parenteral antibiotics remain the mainstay of treatment (Table 1). After bone biopsy and blood cultures are obtained, empiric parenteral antibiotics are initiated. Guidelines for empiric treatment are typically dependent on the age of the patient and the epidemiology of infectious agents in the locality of treatment (Fig. 5; Copley 2009). For instance, in areas with a high incidence of community-acquired MRSA, selecting an antibiotic regimen that is effective against this pathogen is advisable (Copley 2009).
Table 1
Indications/contraindications for nonoperative treatment of osteomyelitis
Osteomyelitis nonoperative treatment | |
---|---|
Indications |
Contraindications |
Mild to moderate acute and subacute hematogenous infection |
Failed nonoperative management |
Chronic osteomyelitis | |
Significant abscess |
The duration of antibiotic treatment as well as the transition from parenteral to oral antibiotics remains an area of debate at this time. The duration of treatment varies among the developed countries of the world, with Finland averaging 3 weeks and Germany 8 weeks. In general, the duration of antibiotic treatment is guided by clinical response and normalization of serum inflammatory markers (CRP, ESR). Traditionally, non-infant AHO has been treated with a prolonged course of intravenous antibiotics (2–4 weeks) followed by another 2–8 weeks of oral antibiotics for most presentations of acute hematogenous osteomyelitis. However, recently published data has led to a general trend toward a shorter duration of both intravenous (IV) and oral treatments at some centers (Pääkkönen and Peltola 2011).
In 2009, a large retrospective study comparing prolonged IV therapy to oral antimicrobial therapy in acute osteomyelitis in children found no significant differences in the rate of treatment failure (Zaoutis et al. 2009). In a prospective, randomized trial of short versus longer course antibiotic treatment of culture-positive AHO in 131 children published in 2010, Peltola et al. noted no difference in outcome between a 20- and a 30-day course of antibiotics, of which the first 2–4 days were parenteral (Peltola et al. 2010). In this study, the authors noted no MRSA in the report and treated children with clindamycin or a first-generation cephalosporin with a 98 % rate of success in each group.
With the move toward shorter-course regimens, some authors have cautioned that while shorter-course treatments may be appropriate for the majority of uncomplicated situations, each case of AHO remains unique and can prove to be a serious, life-threatening entity (Pääkkönen and Peltola 2011). In particular, the optimal treatment duration for MRSA infections or AHO caused by other pathogens such as Kingella or Salmonella remains a matter of debate, with short-course treatments not universally accepted by most clinicians for those pathogens.
Operative
In AHO and SHO, the decision for operative intervention is made on a case-by-case basis by the treating surgeon after a careful assessment of all the available clinical information. Surgery rates vary widely in the peer-reviewed literature, with no agreed-upon criteria for surgical intervention in many cases. However, there are certain instances where there is relative consensus (Table 2).
Table 2
Indications/contraindications for operative treatment of osteomyelitis
Osteomyelitis operative treatment | |
---|---|
Indications |
Contraindications |
Failed nonoperative treatment |
Uncomplicated acute and subacute hematogenous osteomyelitis |
Chronic osteomyelitis |
Resolving clinical picture with nonoperative management |
Combined osteoarticular infection |
|
Significant abscess |
The complexity of the infection (isolated versus extensive osteomyelitis, osteoarticular involvement), the degree of systemic illness of the patient, the degree of inflammatory marker elevation, the prevalence of MRSA in the community, and the lack of adequate response to antibiotic treatment are all factors that one may weigh when considering operative intervention. In general, the presence of abscess has been an indication for surgery, although small collections of infection may be able to be treated without debridement.
When surgery is chosen, the approach should be planned to allow for debridement of the entire extent of the infection. The surgical approach will vary widely between patients depending on the location of the infection, and we have found MRI to be particularly useful in clarifying the most direct route to access the involved osseous region (Table 3). Debridement of all soft tissue fluid collections, adjacent joints where infection is suspected, and periosteal and/or bony abscesses are recommended. As noted above, many cases of AHO involve primarily the metaphyseal region of the long bones, and the creation of a bony window is typically needed to gain access to the main portion of the infection. As with the determination for initial surgical intervention, similar variables are weighed in making the decision for repeat surgery.
Table 3
Surgical planning for osteomyelitis
Surgical planning – osteomyelitis | |
---|---|
Positioning |
Supine with a radiolucent hand table |
Patient with operative arm at the edge of the bed | |
Sterile tourniquet on upper arm for nearly all infections, excluding those in the proximal humerus | |
Mini-fluoroscopy positioned at the end of the hand table | |
Equipment |
Standard hand set including osteotomes and curettes |
Other |
Culture swabs and receptacles for tissue samples (for culture and pathology) |
Hold preoperative antibiotics until cultures have been taken | |
Gravity exsanguination to minimize the chance of spread of bacteria |
The treatment of chronic osteomyelitis remains a challenge to even the most experienced practitioner. The principals of surgery for chronic osteomyelitis in the upper extremity include thorough and extensive debridement of the involved bone and soft tissue, removal of any foreign materials including prior implants, excision of involucrum and/or sequestrum if present, stabilization with methods (i.e., external fixation, splinting, casting) that do not cause further contamination or introduction of foreign materials (when possible), and wound care that in many cases relies on healing by secondary intention. Long-term treatment with targeted intravenous antibiotics with or without local antibiotic cement bead augmentation is also recommended by many.
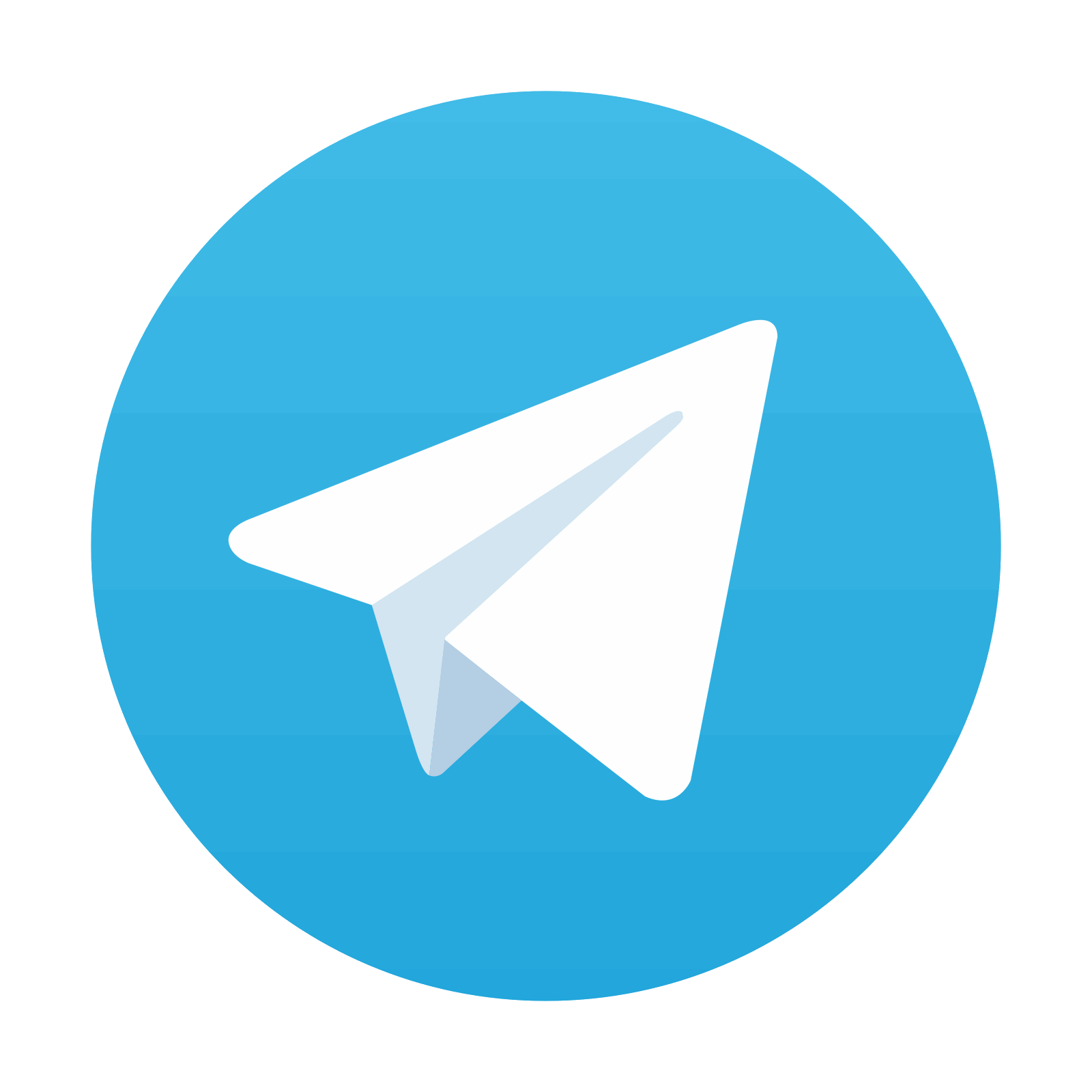
Stay updated, free articles. Join our Telegram channel

Full access? Get Clinical Tree
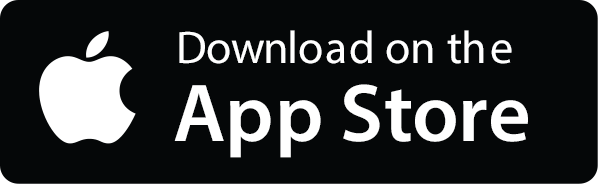
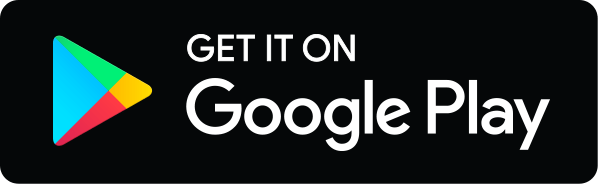