Introduction
The term osteomyelitis denotes inflammation of bone and marrow but generally implies the presence of infection. Osteomyelitis is considered acute if diagnosed within 2 weeks of the onset of symptoms or subacute if symptoms have been present for more than 2 weeks at the time of presentation. Although bacteria are the most common cause, fungi, parasites, and other microorganisms also may cause osteomyelitis. These microorganisms can be introduced into bone in three ways: (1) by direct inoculation, usually traumatic, but also during surgery or due to the presence of orthopedic fixation devices; by local invasion from a contiguous focus of infection; and (3) by hematogenous delivery. In children, osteomyelitis is generally of hematogenous origin. Regardless of the route of infection, the goal of treatment is to arrest the infection and limit the extent of the injury to bone.
The incidence of osteomyelitis in normal children has been examined in several populations. Estimates have varied from 1 in 20,000 adolescent girls in New Zealand to 1 in 1000 Australian Aboriginals. Boys contract the disease 1.2 to 3.7 times more often than do girls. Osteomyelitis occurs most often in the first 2 decades of life. Approximately 25% of children with osteomyelitis are younger than 2 years old, and 50% are younger than 5 years. The incidence is increased in people with sickle-cell disease and in some other immunocompromised individuals (see the section on special populations ).
Although routine cultures fail to identify bacterial pathogens in approximately one-quarter to one-half of all cases of osteomyelitis in children, most microbiologically confirmed infections are caused by a single type of organism. When polymicrobial infections are encountered, they may reflect the spread of infection from contiguous infectious foci and most often occur in the skull, face, hands, or feet. Distal extremities compromised by vascular insufficiency or immobilized because of peripheral neuropathy also are sites of polymicrobial osteomyelitis.
Gram-positive bacteria are most often identified, especially Staphylococcus aureus and Streptococcus pyogenes ; together they account for 80% to 90% of cases in most series ( Table 55.1 ). However, these data are largely derived from studies using traditional microbiological approaches. By contrast enhanced culture techniques and PCR studies have revealed that Kingella kingae , a fastidious gram-negative organism, is an important cause of osteoarticular infections in young children, including osteomyelitis, diskitis, and septic arthritis. Reports demonstrating the importance of Kingella infections as a cause of osteoarticular infections in young children have come from countries as diverse as Israel, the United States, Australia, Switzerland, and Iceland. K. kingae osteomyelitis has been identified at a frequency equal to S. pyogenes in some series, and in several reports Kingella infections exceeded those of gram-positive pathogens.
Organisms | Nelson ( n = 296) | LaMont et al. ( n = 90) | Peltola et al. ( n = 252) | Masson et al. ( n = 95) | Goegens et al. ( n = 45) | McNeil et al. ( n = 190) |
---|---|---|---|---|---|---|
Gram-Positive Bacteria | ||||||
Staphylococcus aureus | 67 | 70 | 75 | 65 | 85 | 83 |
Coagulase-negative staphylococci | 3 | 1 | 4 | |||
Streptococcus pneumoniae | 2 | 5 | 4 | 2 | 4 | 2 |
Other streptococci | 12 | 16 | 10 | 10 | 8 | 10 |
Gram-Negative Bacteria | ||||||
Haemophilus influenzae | 4 | 8 | 10 | 1 | 0 | |
Pseudomonas aeruginosa | 3 | <1 | ||||
Salmonella spp. | 2 | 4 | ||||
Escherichia coli | <1 | |||||
Kingella kingae | <1 | 7 | ||||
Mixed or unusual organisms | 4 | 1 | 10 | 1 |
Streptococcus pneumoniae has been a frequent cause of osteomyelitis in the past, but protein conjugate vaccines have markedly reduced the frequency of invasive pneumococcal infections, including pneumococcal osteomyelitis. Similarly Haemophilus influenzae used to cause approximately 5% to 8% of cases and was found primarily in infants and younger children. Cases of H. influenzae osteomyelitis are noticeably absent from case series published since 2000, likely reflecting the impact of widespread immunization against this organism.
Even in affluent countries Salmonella spp. are occasionally a cause of osteomyelitis in immunocompetent patients, and Salmonella spp. appear to be the most common organism found in cases of osteomyelitis in patients with sickle-cell disease (see later discussion). Osteomyelitis caused by Escherichia coli and other aerobic enteric gram-negative organisms is also seen, generally in neonates and young infants. Osteomyelitis caused by Pseudomonas aeruginosa has been associated with injection drug use.
Although rare in comparison to osteomyelitis caused by aerobic bacteria, four distinct clinical entities of osteomyelitis caused by anaerobic bacteria are recognized: (1) bacteremic seeding of previously normal bones in children and young adults ; superinfection of a fracture site already infected with S. aureus ; (3) indolent (months to years after surgery) infection of a prosthetic device; and (4) contiguous chronic infection, which most often occurs in the skull and the extremities. Bacteroides spp. are found most commonly and are associated with paranasal, sinus, or mastoid infection. In most cases, a foul odor is noted when the bone is incised or the focus is opened; trauma often has been an inciting influence.
Osteomyelitis caused by fungi and other rare bacterial pathogens is discussed in greater detail later.
Hematogenous Osteomyelitis
Pathogenesis
In long tubular bones, hematogenous osteomyelitis generally begins in the metaphysis, the broad cancellous end of the bone shaft adjacent to the epiphyseal growth plate. The cartilaginous epiphyseal growth plate (the physis) is nourished by diffusion of nutrients from capillaries fed by the metaphyseal branches of a nutrient artery. In a long-standing model of disease, capillaries drain into a large sinusoidal plexus that ultimately joins the large sinusoidal veins in the bone marrow ( Fig. 55.1 ). Trauma or emboli lead to occlusion of the slow-flowing sinusoidal vessels, establishing a nidus for infection. Blood-borne bacteria can seed the poorly perfused area and proliferate. Others have suggested that the capillaries adjacent to the physis are open ended, permitting deposition of bacteria into this critical location.

The high frequency of S. aureus in osteomyelitis may reflect specific pathogenic properties of the organisms, including the ability to adhere to type I collagen of bone fibrils. When S. aureus binds to collagen, bacterial replication gives rise to microcolonies surrounded by a glycocalyx. Continued injury, elicited by S. aureus exoproducts and the host cellular inflammatory response to the injury, causes the accumulation of exudate under pressure. The pressure compresses blood vessels of bone and produces focal bone necrosis. The low ratio of surface area to mass, combined with the blood vessel anatomy described earlier, interferes with reabsorption of necrotic cortical bone and the effectiveness of host defense mechanisms. The very early stages of osteomyelitis may be aborted by administration of appropriate chemotherapy. In the absence of therapy, necrosis of cortical bone and marrow continues. The exudate under pressure is forced through the Haversian systems and Volkmann canals and into the cortex (see Fig. 55.1 ).
Beginning in the late 1990s, community-acquired methicillin-resistant S. aureus (CA-MRSA) isolates became a common cause of musculoskeletal infections in the United States and other countries. Most of these CA-MRSA isolates from cases of osteomyelitis carry genes encoding the Panton-Valentine leukocidin (pvl). Pvl-positive CA-MRSA has been associated frequently with sepsis, venous thrombosis, adjacent myositis, and polyostotic disease, suggesting that this or other factors contribute to the development and severity of hematogenous osteomyelitis. However, it is important to note that similar patterns of disease are seen in pvl-negative organisms. Moreover multiple virulence factors are likely involved in the pathogenesis of osteomyelitis, and this remains an area of avid investigation.
Signs and Symptoms
The bacteremic phase of hematogenous osteomyelitis may be entirely subclinical and associated only with malaise and low-grade fever, or it may be characterized by severe constitutional symptoms with high fevers (39°C to 40°C). Subsequent clinical manifestations of osteomyelitis are not related to the severity of initial constitutional signs of infection but are influenced by the age of the child and the etiologic agent ( Fig. 55.2 ).

In newborns, the thin cortex and loosely attached periosteum are poor barriers to the spread of infection. Consequently the purulence rapidly ruptures through both of these structures into the contiguous muscle bed. With progression of the infection, the purulent material often dissects the muscle bundles, with the swollen, discolored limb taking the appearance of a sausage. In addition, the capsule of the diarthrodial joints frequently extends to or is slightly distal to the epiphyseal plate. These anatomic characteristics facilitate extension of infections arising from metaphysis.
In older infants, the cortex (see Fig. 55.2 ) is thicker, and the periosteum is slightly denser. Consequently the infection spreads less often to the soft tissues of the extremity. Subperiosteal abscess and contiguous edema readily develop, generally at the metaphysis, where the cortex is the thinnest. The nutrient metaphyseal capillaries present at birth that cross the growth plate in infants are atrophic by 18 months of age, but this does not appear to alter the risk of developing septic arthritis at adjacent joints in older children, compared to infants, as once proposed.
In children and adolescents (4 to 16 years old), the metaphyseal cortex is considerably thicker, with a dense, fibrous periosteum. The pathogenesis of the infection is the same in this age group, but the infection rarely ruptures and spreads to the outer cortical lamellae. As a result, the signs and symptoms of osteomyelitis in these older children and adolescents usually are more focal.
A newborn with osteomyelitis usually is irritable and displays evidence of pain when the affected extremity is touched or moved. Pseudoparalysis may occur, and if the disease remains untreated, massive swelling of the extremity may be seen. Obtaining a plain radiograph is particularly valuable in newborns; most have changes consistent with osteomyelitis on the initial radiograph, including soft-tissue swelling, periosteal changes, and lytic lesions of bone. In infants and young children, pain is usually accompanied by limping because osteomyelitis occurs more commonly in the lower extremities. The child often refuses to use the affected extremity and displays variable constitutional symptoms.
In older children and adolescents, less restriction of function of the extremity is found compared with infants and young children. Point tenderness is often sharply circumscribed and may be found only as a small area of discomfort at rest.
Most commonly, tubular bones are involved, but osteomyelitis occurs throughout the skeleton ( Table 55.2 ). The hallmark of the disease is the focal nature of symptoms; point tenderness and well-localized pain suggest the diagnosis. Percussion of the long bone away from the area of point tenderness may elicit pain at the site of osteomyelitis in older children and adolescents.
Location | % |
---|---|
Tubular Bone | |
Femur | 25 |
Tibia | 24 |
Humerus | 13 |
Phalanges | 5 |
Fibula | 4 |
Radius | 4 |
Ulna | 2 |
Metatarsal | 2 |
Clavicle | 0.5 |
Metacarpal | 0.5 |
Cuboidal | |
Calcaneus | 5 |
Talus | 0.8 |
Carpals | 0.5 |
Cuneiform | 0.5 |
Cuboid | 0.3 |
Irregular | |
Ischium | 4 |
Ilium | 2 |
Vertebra | 2 |
Pubis | 0.8 |
Sacrum | 0.8 |
Flat | |
Skull | 1 |
Rib | 0.5 |
Sternum | 0.5 |
Scapula | 0.5 |
Maxilla | 0.3 |
Mandible | 0.3 |
The clinical features of osteomyelitis are also influenced by the organisms involved. Osteomyelitis caused by CA-MRSA seems to be more complex and severe than are infections caused by methicillin-susceptible S. aureus (MSSA). Life-threatening infections caused by CA-MRSA have been reported in adolescents, and polyostotic disease is more common (15% in one more recent series compared with 2% in a large series that preceded the emergence of CA-MRSA). Myositis, pyomyositis, intraosseous and subperiosteal abscesses, pathologic fractures, and septic thrombophlebitis also seem to occur more frequently with CA-MRSA than with MSSA. Osteoarticular infections caused by Kingella spp. nearly always arise in children under 36 months of age and usually have an indolent course, with limb pain often present for longer than a week before initial medical evaluation is made; complications and sequelae of osteomyelitis are rare, but Brodie abscess and periosteal abscesses have been described with Kingella . Osteomyelitis caused by H. influenzae seems to occur primarily in the upper extremities.
Culture-negative osteomyelitis is generally milder than diseases with microbiologically confirmed etiologies. In one series comparing 45 culture-positive patients with 40 patients with culture-negative osteomyelitis, symptoms were of longer duration, and overlying skin changes were seen less frequently in patients with negative disease. Treatment with β-lactam antibiotics generally was successful and associated with skeletal sequelae in only one case. Whether these features of culture-negative osteomyelitis suggest a more effective host defense or that these are uniformly caused by a less virulent and more fastidious pathogen, such as K. kingae , remains unclear.
Differential Diagnosis
Infectious osteomyelitis can be confused with many other conditions associated with musculoskeletal pain, fever, and signs of local inflammation. The differential diagnosis includes fracture, systemic infectious and noninfectious inflammatory diseases, and benign and malignant tumors of bone ( Box 55.1 ).
-
Fractures
-
Thrombophlebitis
-
Scurvy
-
Septicemia
-
Cellulitis
-
Septic bursitis
-
Myositis
-
Pyomyositis
-
Rheumatic fever
-
Toxic synovitis
-
Reactive arthritis
-
Complex regional pain syndrome
-
Chronic recurrent multifocal osteomyelitis
-
Osteoid osteoma
-
Langerhans cell histiocytosis
-
Leukemia
-
Ewing sarcoma
-
Malignant primary bone tumors
-
Bone infarction (sickle-cell or Gaucher disease)
Chronic recurrent multifocal osteomyelitis (CRMO) merits special attention in the differential diagnosis. As originally described by Giedion and coworkers CRMO is a chronic illness generally characterized by multiple chronic, focal, inflammatory lesions in bone, with periodic exacerbation and remission and moderate bone pain. It most commonly occurs in girls, with a mean age of onset of 10 years. Many of the cases are in children of northern European origin. Most patients present with localized, multifocal bone pain of gradual onset; only 20% to 50% have fever, but most have an increased erythrocyte sedimentation rate (ESR) or increased C-reactive protein (CRP). The osseous lesions occur primarily in the distal femoral, distal tibial, and proximal tibial regions, although pelvic and clavicular lesions are common, and vertebral lesions are seen in approximately one-sixth to one-quarter of cases. Patients may have 1 to 20 lesions at a time. Biopsy typically reveals a nonspecific chronic inflammatory process, and organisms seldom are identified histologically or by culture; antibiotics bring no improvement in symptoms, Approximately 10% to 20% of patients have a pustular eruption of the palms and soles at the same time that they come to medical attention with bone lesions; this condition is termed pustulosis palmaris et plantaris.
CRMO is a sporadic illness in most cases, but it may also be found as part of syndromic illnesses. Majeed syndrome, for example, is an autosomal recessive life-long disorder encompassing of CRMO, congenital dyserythropoietic anemia, and transient inflammatory dermatosis. It may also occur in the rare patients with deficient interleukin-1 receptor antagonist and in the setting of other inflammatory disorders.
The long-term outlook in children with sporadic CRMO disease generally is good, although numerous relapses may occur. Glucocorticoids and nonsteroidal antiinflammatory drugs are often used as initial therapy, although recurrences are common. Treatment with interferon-γ and tumor necrosis factor-α blocking agents, bisphosphonates, and other agents have been associated with long term responses.
Diagnosis
The diagnosis of osteomyelitis generally is suggested by the presence of fever, focal skeletal pain, warmth, swelling, and a limp or refusal to use an extremity, particularly when accompanied by elevated serum acute-phase reactants (CRP or ESR). In an otherwise healthy individual, the diagnosis is probable when a patient has fever, elevated ESR or CRP, or a positive blood culture plus one or more of the following: abnormal imaging studies (plain radiograph, magnetic resonance imaging [MRI], or computed tomography [CT]) with typical signs of inflammation, injury or repair of bone (see later discussion), scintigraphy indicative of increased bone turnover, or physical findings consistent with osteomyelitis. The diagnosis is confirmed by identification of organisms by culture or Gram stain in an aspirate of bone or by histopathologic evidence of inflammation in surgical specimens of bone.
Microbiology
The cornerstone of the diagnosis of osteomyelitis is isolation of bacteria or other microbes from bone or from anatomic structures contiguous to bone. Overall such cultures (aspirates or surgical specimens from bone debridement, subperiosteal exudate samples, or joint fluid) provide a bacteriologic diagnosis in 66% to 82% of cases. Blood cultures yield an organism in about half of cases (31% to 74% of patients in several series). Recovery of Kingella in culture is enhanced by placing aspirates of fluid into blood cultures bottles. PCR detection of bacterial DNA is likely to become more commonly used in view of its sensitivity and improved detection of fastidious pathogens such as Kingella .
In neonates, needle aspiration of soft tissue or incision and drainage of bone may yield the offending organism. In infants and young children, subperiosteal needle aspiration can be done if the point tenderness is localized. In older children and adolescents, noninvasive culturing of the bone is less rewarding. Performing open procedures to obtain material for culture is somewhat controversial because some orthopedic surgeons consider the risk of causing epiphyseal damage and subsequent length discrepancy secondary to the procedure too great. However, in two recent reports, cultures of bony lesions were positive more than 80% of the time even when antibiotics were given prior to the diagnostic procedure and frequently led to changes in antimicrobial therapy.
Radiology
Plain radiographs.
Conventional radiographs are crucial in establishing the diagnosis of pediatric osteomyelitis and always should be obtained. Because bone density must decrease 50% to be detected by radiographs, changes in the less ossified bones of neonates are detected more readily than are changes in older children. In contrast, Waldvogel and Papageorgiou found in adults that plain radiographs were of no diagnostic value in 23% and were misleading in an additional 16%.
Radiographic changes occur in three stages. The first stage, which occurs approximately 3 days after the onset of symptoms, is the formation of a small area of localized, deep soft tissue swelling, usually in the region of the metaphysis ( Fig. 55.3 ). Consequently when diagnosis of osteomyelitis is sought soon after onset of symptoms, examination of the radiograph should be directed to the soft tissue rather than the bone. During the second stage, which occurs 3 to 7 days after the onset of symptoms, swelling of the muscles with obliteration of the interposed translucent fat planes can be noted. It is caused by continued spread of edema fluid and can progress, particularly in neonates and young infants, to superficial soft tissue edema; the skin may acquire an “orange peel” texture.

Radiographic evidence of bone destruction usually is not detected until 10 to 21 days after the onset of symptoms. The first changes detected include subperiosteal bone resorption, areas of bone destruction, and periosteal new bone formation. The variability depends on the specific bone involved; generally long tubular bones tend to show bony changes 2 to 3 weeks earlier than membranous or irregular bones.
Magnetic resonance imaging.
MRI has become the imaging modality of choice when additional imaging is needed beyond plain radiographs and has sensitivity in excess of 90%. Other major advantages over CT and plain radiographs are MRI that it accurately delineates subperiosteal or soft tissue collections of pus that might require surgical drainage without using ionizing radiation, and it can identify sinus tracts for removal ( Fig. 55.4 ).

In acute osteomyelitis, bone marrow edema caused by the accumulation of purulent material leads to decreased signal on T1-weighted images. On T2-weighted images of the same area, increased signal is seen. Fat-suppression sequences, including short-tau inversion recovery, decrease the signal from fat. Inversion recovery sequences allow more sensitive detection of bone marrow edema. In most cases, gadolinium contrast is not needed for the diagnosis of osteomyelitis, but enhancement may reveal small abscesses that would otherwise be missed. MRI may have a particular advantage in establishing the diagnosis of spinal osteomyelitis because the vertebral body and the adjacent disk are readily distinguished. Loss of this border is one of the first abnormalities detected by MRI in spinal osteomyelitis.
The need for sedation in most infants and children and the cost of MRI are the major factors that continue to impede its use. In addition, infarction and other processes can alter the appearance of bone marrow and lower the specificity of MRI and must be considered in the interpretation of the imaging study.
Radionuclide imaging.
Radionuclide scanning has been used for decades in the evaluation of suspected osteomyelitis. Despite the fact that bone scanning involves exposure to ionizing radiation, it continues to be used widely because of its ready availability and utility in detecting multifocal disease. Bone imaging employing technetium 99m ( 99m Tc) diphosphonate scintigraphy is used most frequently.
Most institutions perform a three-phase bone scan for evaluation of infection. Shortly after injection (2 to 5 seconds), a nuclear angiogram (flow phase) of the area of suspected osteomyelitis is obtained. The second phase (the blood pool phase) consists of a single image obtained 5 to 10 minutes after injection. The third image is obtained 2 to 4 hours after injection. In this later phase, the specificity of the diphosphonate compounds for the bone is revealed: the phosphate adduct is adsorbed to the surface of the hydroxyapatite crystal in bone, and 99m Tc becomes concentrated in the cement line located at the junction of osteoid and mineralized bone. Anything increasing local blood flow to the area, particularly if accompanied by inflammation, results in increased general uptake in the first two phases, but osteomyelitis results in focal uptake in the third phase, with the intensity of the signal detected reflecting the level of osteoblastic activity.
Acute osteomyelitis in children is often diagnosed by a 99m Tc scan and treated successfully before bone changes are detected by plain radiographs (see Fig. 55.4 ). With sensitivity previously reported to be as high as 95%, bone scanning has until recently been a reliable tool in establishing the diagnosis of osteomyelitis. Unfortunately, in one recent report, bone scintigraphy detected only osteomyelitis caused by CA-MRSA cases in 53% (26/49) of children. This apparent lack of sensitivity for CA-MRSA may reflect the acuity of disease caused by this pathogen because changes in radionuclide scans are more characteristic when patients have had an illness of longer duration. In addition to this apparent lower sensitivity for disease caused by CA-MRSA, the reported sensitivity of bone scans in neonates has varied from as little as 31% to as high as 90%. False-negative bone scans in newborns conceivably result from limited spatial resolution, the paucity of mineralization in neonates’ bones, or ischemia of bone.
Older infants with osteomyelitis and a nondiagnostic 99m Tc bone scan also have been described. In such instances, scans employing gallium 67 ( 67 Ga) scan may be valuable. Gallium 67 is a transition metal that, similar to iron, is bound to plasma proteins; the unbound portion (10% to 25%) is excreted in urine. It localizes in inflammatory foci because of increased capillary permeability (leaking plasma proteins), in vivo leukocyte labeling, binding to lactoferrin in the lesion, and perhaps direct bacterial uptake. Because of slower elimination of gallium from blood, its uptake in an inflammatory focus depends less on blood flow. Delayed elimination often results in poor contrast of bone to soft tissue, however, and delays making a reliable interpretation for 24 to 72 hours after injection. Combined evaluation with 67 Ga imaging and 99m Tc bone scanning may lead to greater diagnostic certainty when the studies are not conclusively diagnostic. Despite these successes, 67 Ga scans are seldom employed because repeating MRI or plain radiographs and clinical improvement during therapy usually are sufficient to resolve initial diagnostic uncertainties.
Numerous studies have been conducted to examine the utility of indium 111–labeled leukocyte scans for the diagnosis of osteomyelitis. This method involves removing leukocytes and injecting them back into the patient after in vitro labeling. It has a reported sensitivity of 86% and appears to be most useful for the detection of lesions in long bones. False-positive scans can result from a variety of processes, including fracture and infarction. Enthusiasm for this modality also is limited by the higher organ absorption of the radiation dose. Numerous other scintigraphic methods for the detection of osteomyelitis, including 99m Tc hexamethylpropyleneamine oxime–labeled leukocytes and labeled monoclonal antibodies, have been examined. At present, these alternative methods do not offer any advantage over MRI and older radionuclide imaging approaches.
Computed tomography.
CT is used occasionally in the diagnosis and management of osteomyelitis because it provides excellent definition of cortical bone and high spatial resolution. CT abnormalities commonly found in osteomyelitis include increased density of bone marrow caused by the accumulation of purulent material and periosteal new bone formation and purulence. CT is particularly useful in detecting sequestra and delineating subperiosteal abscesses. It previously has been used to define infections of the spine, but MRI has largely replaced CT for this indication.
Treatment of Acute Hematogenous Osteomyelitis
Surgical Intervention
The need for surgical therapy must be considered immediately when osteomyelitis is diagnosed. Reports of soft tissue, subperiosteal, and intramedullary abscesses have become common in the early 21st century, mirroring the increased prevalence of skin, soft tissue, and musculoskeletal infections caused by CA-MRSA. MRI, ultrasound, and CT imaging may prove useful in assessing these purulent foci. Drainage of these sites by surgical or interventional radiology techniques also provides the opportunity to obtain cultures to confirm a microbiologic diagnosis, thus facilitating selection of the most readily tolerated antimicrobial agent. Sequestra should be removed if present. If contiguous infectious foci are present, they should be debrided adequately and treated with effective antimicrobial therapy. Immobilization of the affected extremity or splinting may afford relief from pain and sometimes is used to prevent the development of pathologic fractures when extensive bone involvement is detected by plain radiography.
Antimicrobial Therapy
The initial therapy should have potent activity against S. aureus and group A streptococci because these pathogens represent the primary causes of osteomyelitis. Acute bacterial hematogenous osteomyelitis should be treated initially with parenteral antiinfective agents in view of the high mortality rate in S. aureus osteomyelitis seen in the preantibiotic era and more recent reports of fulminant disease with CA-MRSA.
The choice of initial therapy has become more complex due to the marked increase in prevalence of CA-MRSA in the United States and other countries since 2000. In areas where most (≥90%) S. aureus isolates remain methicillin-susceptible, initial therapy may consist of a penicillinase-resistant, semisynthetic penicillin, such as nafcillin or oxacillin, administered parenterally in a dosage of 150 to 200 mg/kg per day in four divided doses. Cefazolin, a first-generation cephalosporin, and cefuroxime, a second-generation cephalosporin, have also been used for empiric therapy with good results and may be preferred in infants and young children under 36 months of age if Kingella kingae is strongly suspected based on clinical features. In areas where CA-MRSA is common, either vancomycin or clindamycin (if >90% of CA-MRSA isolates are clindamycin susceptible) should be included in the initial empiric therapy for CA-MRSA. Currently most isolates of CA-MRSA remain susceptible to clindamycin, but clinical laboratories should screen for inducible macrolide-lincosamide-streptogramin resistance using the D-test or similar methodology. In children who are severely ill at presentation, some specialists advise initiating therapy with both vancomycin and oxacillin or cefazolin, in view of the superior activity of these antistaphylococcal β-lactam antibiotics compared to vancomycin for MSSA.
Although protein conjugate vaccines have largely eliminated H. influenzae type B invasive infections in the United States, antimicrobial coverage for this possibility should be considered for younger children who have not yet completed their immunization series. In these circumstances, addition of a third-generation cephalosporin (ceftriaxone or cefotaxime) to the empiric antistaphylococcal agent (vancomycin, clindamycin, oxacillin, or nafcillin) or the use of a fourth-generation cephalosporin such as cefepime might be warranted. Other agents may be administered when epidemiologic factors suggest the possible presence of other pathogens: third- or fourth-generation cephalosporins for P. aeruginosa, Salmonella species, or other enteric gram-negative organisms, and clindamycin for suspected anaerobic infections.
When an organism is isolated or identified by other means, antimicrobial therapy can be chosen with greater specificity. Staphylococci should be treated with penicillin G if the organisms are susceptible to this antibiotic. In most cases, staphylococci must be treated with a penicillinase-resistant penicillin (oxacillin or nafcillin), clindamycin, or vancomycin. Ceftriaxone also has proved successful in the treatment of most cases of bone infections caused by MSSA. Oxazolidinone (linezolid) and streptogramin (quinupristin-dalfopristin) drugs have been used successfully for the treatment of osteomyelitis with MRSA and MSSA and for the treatment of vancomycin-resistant enterococci, but this experience is limited.
Trimethoprim-sulfamethoxazole (cotrimoxazole) and the lipophilic tetracyclines (minocycline and doxycycline) are readily absorbed by the oral route and have been used successfully in the treatment of osteomyelitis in children and adults. One study reported consistent success in 20 children treated with cotrimoxazole, including several children with CA-MRSA infections. However, experience remains limited with cotrimoxazole or tetracycline therapy for osteomyelitis, especially for treatment of CA-MRSA infections in which pneumonia and bacteremia are common, and the potential for dental staining with tetracyclines must be kept in mind. Daptomycin, a bactericidal lipopeptide antimicrobial agent, has excellent activity against CA-MRSA, but pediatric pharmacokinetic data and clinical experience are limited. Moreover daptomycin has poor activity in lung tissue, adding to concerns about the utility of daptomycin for the treatment of CA-MRSA osteomyelitis.
Osteomyelitis caused by S. pneumoniae strains with decreased susceptibility to penicillin have been managed successfully with a variety of agents, including ceftriaxone, vancomycin, and clindamycin. β-lactam antibiotics, including oxacillin, nafcillin, and cephalosporins, have been used successfully in the treatment of K. kingae infection, although cefazolin may be preferable to antistaphylococcal penicillins because the latter have relatively lower activity.
Decades of experience and clinical investigation have led to wide acceptance of sequential use of the intravenous and oral routes of administration of antibiotics to treat pediatric osteomyelitis, which previously was controversial. Completing treatment with oral therapy avoids the cost, pain, inconvenience, and well-known complications of long-term administration of intravenous antibiotics. The relative safety of central venous catheters has not obviated these concerns. To the contrary, local skin infections, bacteremia, and malfunctions seem to be common occurrences when centrally placed catheters are used for osteomyelitis treatment, especially for young children.
Oral therapy is most likely to succeed, and oral therapy is an acceptable option when the following criteria are met: an organism has been identified, the patient has the ability to swallow and retain an appropriate medication, and the patient has a clear clinical response to intravenously administered antibiotics. In most series in which oral therapy has been evaluated, treatment was continued with intravenous antibiotics until the patient was afebrile, until local signs and symptoms of infection were reduced considerably, and until the patient was maintaining caloric and fluid balances by the oral route. Several studies have supported that success with oral therapy is highly likely when these clinical targets are achieved, the peripheral leukocyte count has normalized (if initially abnormal), and there has been a marked decrease in the serum concentration of CRP. A review of case series employing sequential therapy affirmed these recommendations and found no evidence that a fixed period of intravenous therapy is beneficial or essential: a transition to oral therapy within 7 days of diagnosis seemed to be equal in outcome to therapy with a fixed initial period of parenteral therapy.
When oral therapy is begun, most antibiotics administered orally for osteomyelitis must be given in doses higher than those used for the treatment of other infections. Specific antibiotics and the recommended starting doses are listed in Table 55.3 . Dosages of β-lactam antibiotics often can be increased to 150 to 200 mg/kg per day without having serious adverse side effects. Diarrhea, an infrequent complication of high-dose oral β-lactam therapy, can be mitigated by a reduction in dose and the administration of probenecid (40 mg/kg per day every 6 hours; maximal dose 2 g/day). Clindamycin readily achieves high bone levels and does not require higher dose therapy when given orally.
Drug | Dose |
---|---|
Amoxicillin | 100 mg/kg/day divided into 4 doses |
Cephalexin | 150 mg/kg/day divided into 4 doses |
Chloramphenicol | 75 mg/kg/day divided into 3 doses |
Clindamycin | 40 mg/kg/day divided into 4 doses |
Dicloxacillin | 100 mg/kg/day divided into 4 doses |
Linezolid | Age <12 y: 30 mg/kg per day divided into 3 doses; maximum dose 1.8 g/day Age ≥12 y: 600 mg twice per day |
Penicillin V | 100 mg/kg/day divided into 4 doses |
Trimethoprim-sulfamethoxazole | 16 mg/kg/day (for trimethoprim component) |
The assumption implicit in successful oral therapy is that the antibiotic reaches an effective concentration at the focus of infection. Compliance with the prescribed dose and frequency and absorption into the bloodstream are necessary to fulfill this assumption. Patient (or parent) education and a continuing time commitment by a physician or nurse are essential to maintaining the compliance required for successful treatment. Although not commonly employed, therapeutic drug monitoring (TDM) may be useful to show that adequate absorption of orally administered antibiotics is occurring. This recommendation stems from the observation that rare patients have inadequate serum levels despite receiving high oral dosages. TDM also can be used to document adherence to the therapeutic regimen. TDM was initially performed by measuring serum bactericidal activity, with a peak serum bactericidal titer of 1 : 8 or greater sought. However, this assay is confounded by other antibiotics present in the serum sample (e.g., nafcillin administered intravenously would interfere with evaluating the oral bioavailability of oral dicloxacillin). Chemical assays that detect the specific antimicrobial agent circumvent this problem. For example, dicloxacillin can be administered orally and the dose adjusted based on dicloxacillin measurements before the intravenous administration of another β-lactam has been discontinued. This procedure avoids several days of inadequate therapy should the dosage of the oral agent need to be adjusted.
In most cases of uncomplicated disease, clinical improvement is evident within 3 to 7 days of the initiation of appropriate therapy. To help show that effective therapy is under way, it is helpful to monitor acute-phase reactants. Although CRP and ESR typically increase during the first 2 days of therapy, CRP then begins to decrease rapidly (with an approximate half-life of 1 to 2 days) and typically returns to normal in 7 to 10 days. CRP returns to normal levels in blood more rapidly in children with an uneventful clinical course than in children who ultimately require repeated surgical drainage. A slow decline in serum CRP to normal levels has been associated with more extensive radiographic changes or persistent symptoms 1 to 2 months after discharge from the hospital. Higher peak CRP levels may also indicate the presence of concomitant septic arthritis and adjacent osteomyelitis. Similar to CRP, ESR generally increases during the first several days but declines in the weeks that follow. Failure of ESR to decrease during the second week of treatment may indicate a need for surgical drainage or the development of chronic osteomyelitis.
Prolonged antibiotic therapy is essential for successful treatment of acute osteomyelitis. In one early report of 45 cases of osteomyelitis, four treatment failures occurred; all had been treated for 10 days or less. Likewise Dich and colleagues noted a 19% failure rate in 37 patients treated for 3 weeks or less; the rate was 2% in 48 patients treated for 21 to 50 days. Blockey and Watson provided similar data. Thus, 3 weeks or more appears to be the minimal duration of therapy for hematogenous osteomyelitis to achieve a low rate of recurrence. A shorter period of therapy was sufficient for most patients in one widely cited study conducted in Finland, but no cases of MRSA were identified in this series of 252 patients. In contrast to this, CA-MRSA infections are common in the United States and many other countries. A conservative but individualized approach is to administer antibiotics until ESR and CRP are both within the normal range, which usually requires 4 to 6 weeks of treatment.
Special Manifestations of Hematogenous Osteomyelitis
Brodie Abscess
Osteomyelitis occasionally is indolent in presentation. Perhaps the best-defined example is subacute osteomyelitis with the development of a localized and well-contained intraosseous abscess (Brodie abscess). These lesions are most often identified in adolescents with complaints of long bone pain and tenderness and generally occur in the tibia or femur. Fever is generally absent, and the ESR usually is normal at the time of presentation. A bony defect with sclerotic margins is detected by plain radiography in most patients. A distinctive “target” lesion has been described in MRI studies of Brodie abscesses. Concentric layers are seen and reflect a central abscess cavity surrounded by an inner ring of granulation tissue, an outer ring of fibrotic reaction, and a peripheral rim of endosteal reaction that is hypointense on T1-weighted images.
S. aureus and other gram-positive cocci (including β-hemolytic streptococci and Propionibaceterium acnes ) are generally the pathogens identified, but a variety of gram-negative organisms, including H. influenza, Pseudomonas aeruginosa, Salmonella typhi, Klebsiella species, and Kingella kingae have also been isolated from these lesions. Treatment consists of surgical drainage and curettage of the lesions followed by antimicrobial therapy, as for other forms of hematogenous osteomyelitis. Bone grafting may be needed for larger lesions. The prognosis generally is good, although deformities occur in some cases.
Osteomyelitis in Patients After Closed Fractures
Acute hematogenous osteomyelitis sometimes occurs in closed fractures of tubular bones. A clue to diagnosis is the resumption of pain after the initial postfracture pain has subsided, usually 1 to 6 weeks after the injury occurs. The pain differs from that associated with the fracture by being progressive and not being relieved by immobilization. Patients are febrile and may be thought to have another focus of infection before osteomyelitis is discovered. When the cast is removed, local erythema, fluctuance, and warmth are apparent and out of proportion to the normal healing of fracture. Although usually caused by S. aureus , anaerobic superinfection of staphylococcal osteomyelitis at the fracture site has been described. Successful therapy requires adequate debridement, administration of appropriate antibiotics, and external fixation in some cases.
Epiphyseal and Apophyseal Osteomyelitis
Rarely, hematogenous osteomyelitis may arise in the epiphyses or, more rarely, apophyses (e.g., the greater trochanter of the femur) of the tubular bones of young children. Although the pathogenesis is unclear, it may involve delivery of microorganisms to the epiphyses by transphyseal vessels in some children. After the child reaches 15 to 18 months of age, these vessels are atrophic. In older children, primary epiphyseal infections have been hypothesized to result from delivery of bacteria via venous sinusoids or by terminal branches of the epiphyseal arteries.
Hematogenous epiphyseal osteomyelitis may be acute or subacute. At the time of presentation, septic arthritis initially may be diagnosed when swelling of the related joint occurs and elevated synovial leukocytes are identified by diagnostic aspiration.
In cases with a more indolent course, pain, limp, or other symptoms prompt an evaluation for the possibility of an osteoarticular infection. In these cases, white blood cell counts are usually normal, and ESR and CRP are abnormal in about 50% of children. The correct diagnosis is usually established when a radionuclide bone scan shows evidence of increased bone turnover or plain radiographs taken weeks later reveal lytic changes characteristic of osteomyelitis.
S. aureus is the pathogen most often found; one recent series noted that Kingella kingae is frequently found in young children through the use of polymerase chain reaction (PCR) and more sensitive culture techniques. Administration of appropriate therapy, as for other cases of acute osteomyelitis, has been followed by complete recovery without apparent sequelae 2 to 6 years after diagnosis.
Involvement of Nontubular Bones
Less than 20% of all cases of osteomyelitis involve nontubular bones. Infection of the calcaneus is the most common. Osteomyelitis in the other cuboidal bones rarely occurs ( Table 55.2 ). In patients with hematogenous infection of the calcaneus, most present after 7 days of symptoms. Destruction occurs just under the epiphyseal line in the metaphysis posteriorly and medially, where the blood supply is greatest. It is present in all patients, in addition to destruction of the adjacent epiphysis, particularly in its middle to superior portion. Periosteal new bone formation occurs very late, with 3 to 4 months required for reossification. Osteomyelitis of the calcaneus is treated in much the same fashion as infections involving tubular bones, although complications have been frequently described, and recurrences may be more common than in osteomyelitis of other bones of children (5% in one series).
Almost equal in frequency to infection of the calcaneus is infection of the bones of the pelvis. Of the bones of the pelvis, the ischium is involved most commonly. The next most frequently involved bone is the ilium, followed by the sacroiliac joint. The pubis is involved in only 20% of cases of pelvic osteomyelitis. Pelvic osteomyelitis causes an increase in the ESR in nearly all patients, and two-thirds have a peripheral leukocyte count greater than 10,000 cells/mm 3 . The most common organism causing pelvic osteomyelitis is S. aureus, which is isolated from either blood or an aspirate from the bone lesions in approximately 80% of cases.
Establishing the diagnosis of pelvic osteomyelitis is often difficult. Most patients are judged to have disease in the hip at the time that medical attention is sought. Most often, patients with pelvic osteomyelitis have hip pain and a gait abnormality but allow their hips to be put through a passive range of motion. Point tenderness at the site of the lesion can be elicited in approximately 50% of these patients. Sacroiliitis frequently is difficult to identify by clinical examination. Pressing down on the pelvis, which stresses the sacroiliac joint, produces local pain. Tenderness in the buttocks or the sciatic notch, if present, is an important diagnostic finding. Pelvic osteomyelitis can mimic appendicitis and urinary tract infection. It occurs more frequently in individuals with inflammatory bowel disease. In most patients, plain films of the pelvis are normal, whereas MRI is highly sensitive in identifying evidence of infection. Gadolinium enhancement improves the recognition of sacroiliac joint involvement. Technetium 99m bone scans are also useful and indicate the diagnosis in approximately 90% of cases. Antibiotic therapy alone is adequate in most cases of pelvic osteomyelitis. Surgery is indicated only when a lack of response to antimicrobial therapy occurs. Osteomyelitis in the pelvic bones has a uniformly good prognosis; chronic infection and sequelae are rare events.
Hematogenous osteomyelitis of flat bones occurs rarely and has been described in the skull, ribs, sternum, and scapula.
Spinal Osteomyelitis
Spinal osteomyelitis can involve either the intervertebral disk or the vertebral bodies. Conceptualizing these infections as different entities is worthwhile because of the different pathophysiology and prognosis.
Diskitis
The intervertebral disk consists of three components: the paired cartilaginous articular endplates, the fibrous ring (annulus fibrosus), and the nucleus pulposus. The axial vessels that parallel the fetal notochord atrophy by birth, with the avascular, mucilaginous nucleus pulposus remaining. The disk has two arterial supplies: periosteal vessels and vessels descending from the central portion of the vertebral body. The vessel from the central portion of the adjacent vertebra begins to atrophy in the first year and is obliterated completely by the time the child is 10 years old. This condition leaves only the capillary network in the annulus fibrosus, which is derived from the terminal radial ramifications of the periosteal vessels. If loss of this vascular supply is precipitous, idiopathic disk necrosis ensues, usually manifested as asymptomatic calcification. If bacteremia occurs during loss of the blood supply, however, infection of an intervertebral disk may occur.
Most children with diskitis are younger than 3 years of age and have had symptoms for several weeks. Most cases of diskitis involve the thoracolumbar region, and the disease comes to medical attention with the patient’s refusal to walk. Back pain and a progressive limp may also be present. Nonambulatory infants often become irritable and refuse to sit or crawl. On examination, the most striking feature is percussion tenderness over the contiguous spine; hip pain and stiffness with loss of lordosis of the lower part of the back are observed. Occasionally compression of the spine produces pain at the infected disk. Lesions higher in the spine (T8 to L1) can mimic gastrointestinal disease with abdominal pain, ileus, and vomiting, but the most important entities in the differential diagnosis are vertebral osteomyelitis and spinal or paraspinal tumors. Cervical diskitis has also been reported in children as well; limited neck movement or cervical pain appear to be characteristic.
Fever generally is absent or low grade. Peripheral leukocytosis is present in one-third of patients, and virtually all have an increased ESR. A few patients undergoing biopsy have cultures that grow microorganisms. Most commonly, S. aureus is recovered, but K. kingae is being increasingly recognized as a cause of diskitis in children. Rarely, pneumococci and gram-negative bacilli are also identified in cultures of blood or invasive sampling. Initial evaluation for suspected diskitis usually involves careful elicitation of the history and physical examination, a complete blood count, determination of the ESR and serum CRP concentrations, a blood culture, and plain lateral radiographs of the lumbosacral spine.
Typical plain radiographic findings are shown in Fig. 55.5 . The first finding is narrowing of the disk space, usually not detectable until 2 to 4 weeks after the onset of symptoms. Frequently this narrowing is overlooked if loss of the normal progressive (from cephalad to caudad) increase in disk width is not appreciated. It is followed by destruction of the adjacent cartilaginous vertebral endplates and may subsequently be followed by herniation of the disk into the vertebral body. Rarely compression or wedging of the vertebral body is noted. In all individuals, reactive bone proliferation is a rare finding, as are paravertebral soft tissue masses.

Full access? Get Clinical Tree
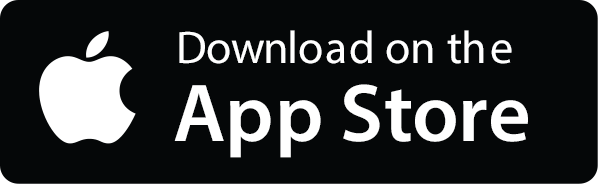
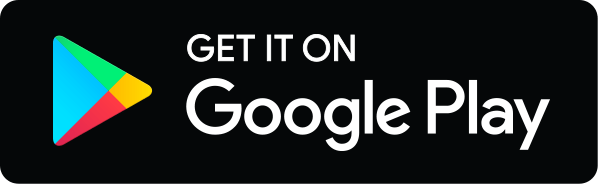