The PGC specification in mouse embryos. Mouse PGCs are specified at the posterior and proximal end of the epiblast (EPI) by receiving BMP4 from extraembryonic ectoderm (EXE), a trophectoderm lineage, which later contributes to the placenta, and WNT3 from visceral endoderm (VE) and/or EPI. CER1, an antagonistic factor for BMP4 produced by anterior visceral endoderm (AVE), inhibits the formation of PGCs at the anterior EPI
25.2.1 In Vitro Gametogenesis in Mice
PGCs are a scarce and transient entity, present only at the embryonic period thereby precluding the detailed biochemical analysis requiring a large number of cells. Induction of PGCs from pluripotent cells, such as mouse ESCs (mESCs) or iPSCs (miPSCs), therefore, have been awaited to overcome such hurdles. Many of such attempts have been done since early 2000, but none of them have successfully demonstrated the contribution to functional gametes or healthy offspring, likely due to the poor induction efficiency and/or the lack of appropriate markers to isolate and characterize the in vitro derivatives [13–16]. Notably, these studies utilized markers that have poor PGC specificity or are expressed only at a late stage, thereby failing to recapitulate the early PGC development and thus the following developmental trajectories leading to mature gametes. To reconstitute the perplexed and protracted germ cell developmental processes in vitro, faithful reconstitution of such processes in a stepwise manner is of paramount importance. By using epiblast cells harboring Blimp1-mVenus (BV), Stella-Ecfp (SC) reporters to visualize the formation of early PGCs, Ohinata et al. successfully induced the BVSC (+) PGC-like state from pre-gastrulating epiblast cells in a dish by adding BMP4 and other growth factors (SCF, EGF, LIF, BMP8b) (Fig. 25.2) [8]. These cells closely resembled PGCs in vivo in both gene expression and epigenome pattern. Upon transplantation into the seminiferous tubules of c-Kit mutant (W/Wv) mice depleted of endogenous germ cells, they differentiated into functional sperm and successfully contributed to the healthy offspring after intracytoplasmic sperm injection (ICSI). In the following study, Hayashi et al. successfully induced mouse primordial germ cell-like cells (mPGCLCs) in vitro from BVSC (+) naïve mESCs or miPSCs maintained in the presence of 2i (CHIR99021, PD0325901) + LIF [17]. In this study, they first cultivated naïve mESCs or miPSCs for 2 days in the presence of Activin-A and bFGF to induce epiblast-like cells (EpiLCs), which closely resemble germline competent E 5.75 pre-gastrulating epiblast. EpiLCs were further induced into mPGCLCs by BMP4-based cytokine cocktails as above used by Ohinata et al. Notably, mPGCLCs exhibit highly similar transcriptome and epigenome profiles with in vivo PGCs at ~E9.0 and contribute to the functional spermatogenesis upon transplantation into W/Wv mice. Again, healthy pups were generated through intracytoplasmic sperm injection (ICSI) of the mPGCLC-derived sperms and transferring the resultant zygotes to foster mothers.
In a subsequent study, Hayashi et al. similarly induced female mESCs into mPGCLCs in vitro (Fig. 25.2) [18]. By culturing with somatic cells obtained from embryonic ovaries (“reconstituted ovary” method), mPGCLCs further differentiated into the oocytes that were in prophase of the first meiotic division. Upon transplantation into ovaries of immune-deficient mice, mPGCLC-derived oocytes matured into fully grown oocytes, which, through in vitro maturation (IVM) and in vitro fertilization (IVF), became zygotes and contributed to fertile offspring [18]. Together, these studies for the first time provided convincing evidence that the mouse germline can be reconstituted in vitro by using pluripotent stem cells.
Notably, all of the above attempts of mouse in vitro gametogenesis relied on host niche environment for terminal maturation into oocytes or spermatozoa through the transplantation of mPGCLCs into recipient mice (Fig. 25.2) [8, 17, 18]. More recently, however, several groups have reported the successful reconstitution of the latter half of oogenesis in vitro. In these studies, authors divided the culture into three steps – in vitro differentiation (IVD), in vitro growth (IVG), and IVM according to the in vivo oogenesis pathway. By careful validation of each step using the reconstituted ovaries, authors successfully reconstituted the entire process of oogenesis in vitro and generated healthy offspring by IVF of the in vitro reconstituted eggs [19–21]. These studies provide a valuable platform for analyzing the mechanisms of oogenesis in vitro and will serve as a guide for future in vitro gametogenesis in humans.
In male testes, the presence of spermatogonial stem cells with self-renewal capabilities allow for production of terminally differentiated spermatozoa for a prolonged period of time [21]. In mice, long-term culture of cells with robust spermatogonial potential, named germline stem cells (GSCs), can be established from both neonatal and adult testes [22, 23]. Recently, Ishikura et al. successfully induced GSC-like cells (GSCLCs) from male mPGCLCs by culturing with gonadal somatic cells obtained from E12.5 male embryos (Fig. 25.2) [24]. Upon transplantation, GSCLCs were capable of colonizing in the adult testis and generated functional sperm, features characteristic of GSCs. Previously, Ogawa and colleagues successfully induced sperm from GSCs through organ culture of neonatal testes into which GSCs were transplanted before culture [25]. Combining these methods together, reconstitution of the entire process of spermatogenesis in vitro will be technically feasible and achieved in the near future.
25.3 The Development of Germline in Humans and Nonhuman Primates
Precise understanding of how human germline develops in vivo is the prerequisite information for human in vitro gametogenesis. However, until very recently, human germ cell development has been poorly understood due to ethical and technical constraints in analyzing a scarce population which is only present in embryos and fetuses. To date, our knowledge of human germ cell development has been mostly attributed to the morphologic description conducted in the early to mid-twentieth century.
One of such earliest descriptions dates back to nearly a century ago, when Fuss identified the cluster of cells with unique morphologic features suggestive of human PGCs (hPGCs) at the posterior aspect of the yolk sac at ~4 weeks post conception (wpc) of human embryos [26, 27]. Subsequent series of histological and ultrastructural evaluation provided further evidence that PGCs emigrate from the yolk sac endoderm and further migrate through the hindgut endoderm and dorsal mesentery until they reach the gonads at ~5 wpc [28–30]. Recently, several groups reported transcriptome and immunohistochemical profiles of hPGCs within the gonads [31–34]. Gonadal hPGCs show expression of germ cell markers (e.g., BLIMP1, TFAP2C, NANOS3, DND1, DDX4, DAZL) and pluripotency-associated genes (e.g., POU5F1, NANOG, SALL4, LIN28A, PRDM14) similar to mPGCs. Notable divergence, however, does exist between hPGCs and mPGCs. SOX2, a core transcription factor for pluripotency and critical for germ cell development in mPGCs, is not expressed in hPGCs, which instead shows strong and persistent expression of SOX17, previously known as an endodermal marker [32, 35]. SOX17, on the other hand, is only transiently expressed in early mPGCs but not in gonadal mPGCs [36]. Epigenetic features of hPGCs in particular genome-wide methylation has also been investigated over the last few years, which showed that global demethylation occurs in hPGCs at the migratory and early gonadal stage with their genome-wide 5-methylcytosine (5mC) levels reaching ~5% at 9 wpc, similar to mPGCs [31–33].
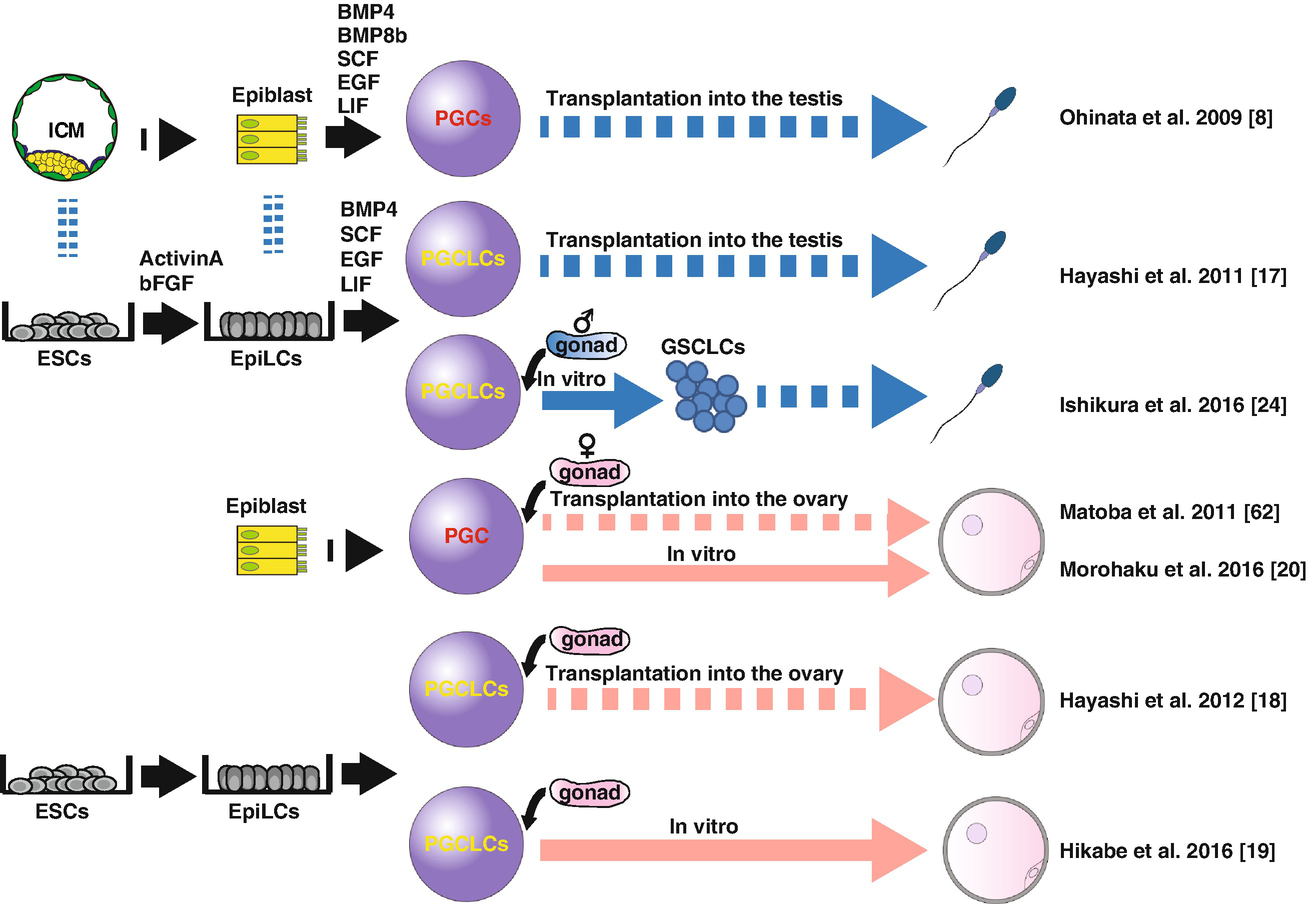
Functionally validated in vitro gametogenesis in mice. First successful demonstration of the reconstitution of mouse PGC development dates back to 2009, when Ohinata et al. showed that epiblasts at E6.0 can be induced ex vivo into PGC-like cells (PGCLCs) by culturing in the presence of BMP4, BMP8b, SCF, EGF, and LIF, which matured into functional sperm upon transplantation into the neonatal testis [8]. In subsequent studies, ESCs were used as a starting material to induce epiblast-like cells (EpiLCs) through the monolayer culture in the presence of Activin-A and bFGF, which were in turn induced into PGCLCs [17]. PGCLCs similarly contribute to functional spermatogenesis upon transplantation. In 2011, Matoba et al. developed a method in which PGCs were induced ex vivo into mature oocytes through ectopic transplantation into kidney capsules [62]. With minor modification of this method, Hayashi et al. successfully induced fertile oocytes derived from PGCLCs [18]. More recently, the latter oogenesis processes were recapitulated in vitro first using PGCs ex vivo [20] then PGCLCs induced from mESCs [19]. These studies used the “reconstituted ovary” by which germ cells were cultured together with gonadal somatic cells obtained from E12.5 ovaries. Male spermatogenesis has also been partially reconstituted; germline stem cell-like cells (GSCLCs) were induced from PGCLCs through reconstituted testis, which, upon transplantation into recipient testis, contributed to the fertile spermatozoa [24]
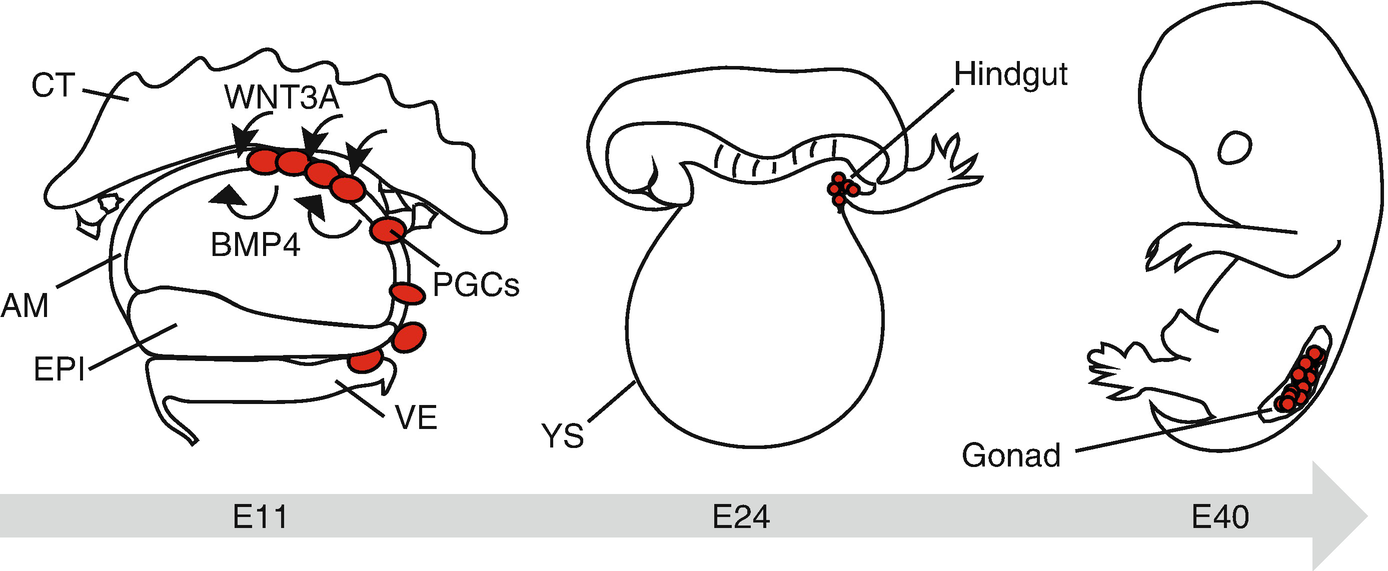
Primate germ cell development in vivo. Cynomolgus PGCs (cyPGCs) first appear at the dorsal aspect of the nascent amnion at E11. BMP4 and WNT3A, critical specifiers of germ cell fate, are expressed in the nascent amnion (AM) and cytotrophoblast (CT), respectively. cyPGCs subsequently move into the visceral endoderm (VE) through the posterior AM. Note that cyPGCs are not identified within the epiblast (EPI). cyPGCs thereafter form the loose cluster at the posterior end of the yolk sac endoderm (YS) (a derivative of VE), which is incorporated into the hindgut endoderm upon folding of the embryo at ~E24. cyPGCs further migrate into the bilateral gonads through the dorsal mesentery by ~E40
25.3.1 Induction of Human PGC-Like Cells in Humans
Reconstitution of the human germline in vitro using hESCs or hiPSCs has a tremendous impact on both basic research and clinical perspectives. Since human embryos before 4 wpc, which is when specification of hPGCs occurs, are inaccessible due to ethical reasons, human PGC-like cells (hPGCLCs) induced in vitro from pluripotent stem cells provide the indispensable tool in understanding the molecular mechanisms of hPGC specification. Additionally, the reconstitution of the human germline in vitro will also enable the molecular dissection of the critical events during the subsequent germline development including epigenetic reprogramming, genomic imprinting, X-chromosome reactivation, or meiosis through gain- or loss-of-function analysis which, in turn, provides critical insight into causes of infertility or birth defects as a consequence of abnormal germ cell development [2]. Moreover, creation of fertile eggs or sperms in a dish provides new opportunities for infertile couples possessing defective gametes. Despite such significance, human in vitro gametogenesis has several hurdles to be overcome, making it more challenging than its mouse counterpart. First, our knowledge of human germline in vivo, in particular, that of before 4 wpc is very limited, which makes it difficult to assure that hPGCLCs recapitulate hPGC development in vivo. Second, species specific differences exist in the property of ESCs/iPSCs between mice and humans. hESCs/hiPSCs exhibit “primed” pluripotency, resembling peri-gastrulating epiblasts in vivo, while mESCs/miPSCs show “naïve” pluripotency recapitulating the features characteristic of inner cell mass (ICM) [37]. Although mESCs/miPSCs are known to be germline competent, it has yet to be known whether hESCs/hiPSCs have such competency. Third, an appropriate assay system to test the functionality of germ cells is lacking in humans. Although with ethical and legal constraints, fertilization using hPGCLC-derived gametes and observing the successive embryo development may provide clues that such germ cells have a potential to create the embryonic and extraembryonic tissues, a hallmark of germline [2].
Despite such technical and ethical constraints, many laboratories have attempted the induction of human germline from pluripotent stem cells over the last two decades. These studies used various methods including random differentiation [40, 41], directed differentiation using BMP4 [42–44] or retinoic acids [45], or overexpression of DAZ gene family [43]. These studies however suffer from either low induction efficiency due to the failure to recapitulate hPGC development in vivo or inappropriately gauge the induction using markers that is not specific to or only detectable at the late stage of human germ cells (e.g., DDX4, DAZL, or meiotic markers).
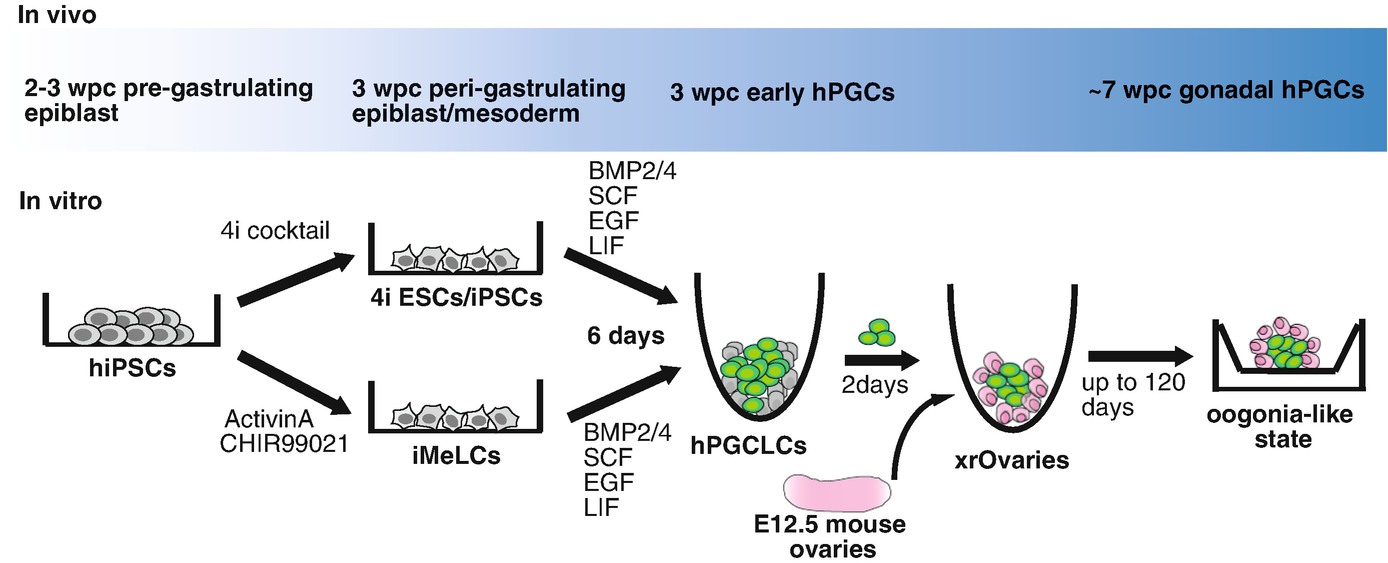
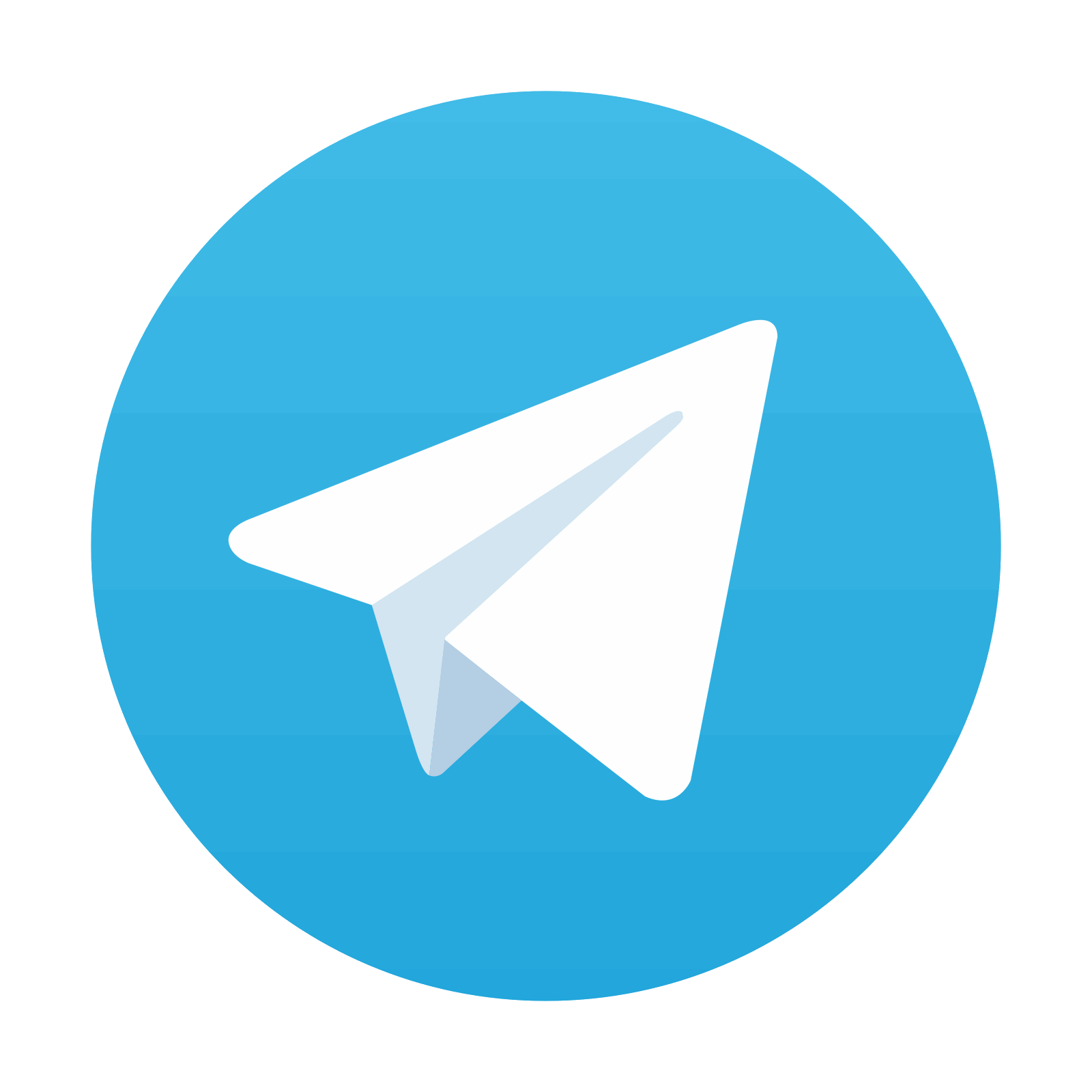
Stay updated, free articles. Join our Telegram channel

Full access? Get Clinical Tree
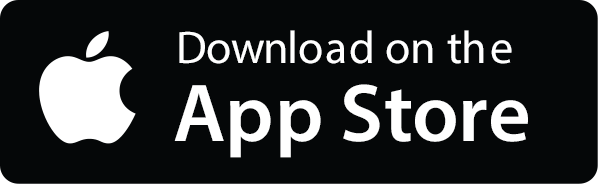
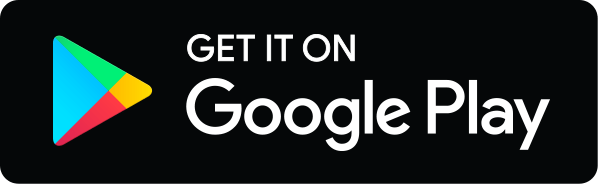