Although optimal growth of the neonate can be insured with a caloric intake from 100 to 150 kcal/kg/D, during acute metabolic stress (injury) states, characterized by increased C-reactive protein and decreased prealbumin serum concentrations, daily energy repletion should be based on either measured energy expenditure values or basal metabolic rate.
The metabolic rate increases 10% to 13% per degree centigrade elevation and 7.2% per degree Fahrenheit elevation.
The nutritional caloric regimen for the metabolic impact of acute injury in infants does not require the replacement of calories allotted for growth.
Glycemic control during the resuscitative and stabilization phase of acute illness may provide a favorable impact on patient morbidity and mortality.
Indirect calorimetry is a useful energy assessment tool. During critical illness, changes in daily energy repletion are dependent on the magnitude and duration of the acute metabolic stress response, but do not include energy required for growth, insensible losses, or activity.
Both enteral and parenteral nutrition are effective in reducing the hypermetabolic response to injury as well as reducing protein catabolism.
Optimal nutritional therapy is an essential requirement in the postoperative care of pediatric surgical patients, especially infants and children in the intensive care setting. Growth velocity during early infancy is higher than at any other time during childhood and is exceeded only by intrauterine growth rates. Daily energy needs range from 100 kcal/kg/day in term infants to 150 kcal/kg/day in very premature babies. Although these energy needs decrease in older children, growth and activity requirements still exceed those of normal adults. In the surgical population, particularly in critically ill patients, metabolic status is significantly altered. Perioperative acute tissue injury markedly reduces these energy needs, due to the injury-related induction of catabolic metabolism (which inhibits growth), sedation (which inhibits activity), and environmental temperature control (which reduces insensible heat loss). In concert, these factors result in a substantial decrease in energy needs, especially in the mechanically ventilated child. To account for these alterations in energy metabolism, caloric amounts equal to measured energy expenditure (MEE) values or basal energy requirements should be provided to prevent overfeeding (ie, the provision of calories and/or nutritional substrates in excess of the energy required to maintain the metabolic homeostasis). Overfeeding can create or prolong the requirement for mechanical ventilation (increased ventilatory workload), impair liver function (induced hepatic steatosis and cholestasis), and increase injury-induced hyperglycemia (increased risk of infection). Nutritional assessment of critically ill pediatric patients can be quantitatively accomplished by measuring the visceral protein pool (ie, serum C-reactive protein [CRP]), the acute-phase protein pool (ie, serum prealbumin), and energy expenditure. Serum prealbumin levels decrease and CRP levels increase with a magnitude and duration proportional to injury severity, and the values return to normal as the acute injury response resolves. Serum CRP concentrations have been shown to correlate well with MEE; however, predictive equations for energy expenditure are inaccurate. A substantial percent of preterm infants are dependent on total parenteral nutrition (TPN) to prevent hypoglycemia and provide a sufficient energy intake due to inadequate bowel absorptive function. However, diminished tolerance for parenteral glucose delivered at high rates frequently provokes hyperglycemia in this patient population. Hyperglycemia is further increased in the presence of infection, which impairs hepatic glucose uptake and enhances insulin resistance. Hyperglycemia can cause immunocompromise and is associated with an increased risk of infection-related morbidity and increased mortality in both adult and pediatric intensive care populations. To avoid overfeeding during acute injury states, critically ill infant and pediatric patients should be administered basal energy requirements until serial serum CRP concentrations decrease in association with increasing serum prealbumin concentrations, at which time caloric intake can be safely advanced. While enteral nutritional delivery is generally preferred, parenteral nutrition is often necessary due to intestinal dysfunction.
The assessment of energy expenditure has been widely used to characterize alterations in metabolism and to determine daily caloric requirements accompanying a variety of clinical states in both health and disease. Energy can be partitioned into (1) maintenance metabolic needs (BMR, activity, and heat loss to the environment) and (2) energy required for growth. Energy requirements are age-related and are 3 to 4 times higher for infants than for adults.
In healthy, term babies, the BMR is about 40 to 45 kcal/kg/day in the neonate, increases to about 60 kcal/kg/day by approximately the fourth to fifth month of life, and then gradually declines to about 20 to 25 kcal/kg/day during adolescence, largely due to a relative decrease in the growth of parenchymal organs with high oxygen consumption rates (brain, liver, kidney, and heart) and an increase in muscle mass (with relatively low oxygen consumption) and fat. Total daily energy requirements for healthy, uninjured children decrease progressively with age, from 90 to 120 kcal/kg/day (with 2.5-4 g/kg/day protein) during the first year of life gradually down to 30 to 60 kcal/kg/day (with 1.5 g/kg/day protein) during the adolescent and teenage years. However, estimates of energy requirements in the postsurgical setting are often unreliable, especially in critically ill pediatric patients, due to a high degree of interpatient variability. An accurate measure of energy expenditure can provide information necessary for appropriate nutritional repletion, including the type and amount of macronutrient substrates needed. In disease states, especially during critical illness, these considerations may substantially aid in improving mortality and morbidity outcomes following acute injury states, particularly by allowing for crucial metabolic resuscitation while avoiding the administration of calories in great excess of actual energy requirements. While a variety of assessment techniques have been described, indirect calorimetry is the method most frequently employed. Indirect calorimetry can be carried out at the patient’s bedside using a metabolic cart to measure energy expenditure on the basis of oxygen consumption and carbon dioxide production. If indirect calorimetry is unavailable, BMR values should be used as a guide for daily caloric administration until the acute injury response resolves (see Nutritional Assessment and Resuscitation in the Postinjury Setting below).
Growth can be expressed in terms of protein accretion, which is the amount of protein generated as new tissue. Nitrogen accounts for approximately 2% of total body weight at birth in contrast to just about 3% in the adult. Most of this difference is made up in the first year of life because of rapid somatic growth. During this first year, the infant’ body length and body weight increase by 2-fold and 3-fold, respectively. Parenchymal growth is particularly accelerated during this period. For instance, the brain mass grows to 60% of its normal adult size during the first year of life. However, energy needs to take metabolic precedence, so that protein will be preferentially used as an energy source (even if protein delivery is low) if nonprotein substrate delivery is inadequate to meet energy needs.
In the healthy infant, protein as a function of body weight is highest in the neonate (0.93 g/kg/day) and decreases progressively from that point. Protein accretion is 0.5 g/kg/day during the second and third months of life, 0.26 g/kg/day during the fifth and sixth months of life, 0.18 g/kg/day from 9 to 12 months of age, and 0.08 g/kg/day between 2 and 3 years of age. Protein accretion is dependent on the amount of protein actually absorbed (metabolizable protein), the efficiency of conversion of various dietary proteins into tissue protein (estimated at 90% for breast milk but only 70% for soy protein found in infant formulae), and the protein lost during breakdown.
Protein lost because of incomplete enteral absorption and breakdown can be estimated by measuring stool, urine, and skin nitrogen content and the loss is calculated to be approximately 0.95 g/kg/day of protein during the first year of life. Taking these factors into account and allowing for the interpatient variability, the estimated enteral protein requirement is approximately 2.6 g/kg/day during the neonatal period, 2 g/kg/day at 2 to 3 months of age, and 1.3 g/kg/day at 1 year of life. Values may be somewhat less for parenteral delivery because of decreased absorptive losses.
In the premature or small-for-gestational-age (SGA) child, protein needs are proportionately higher (ranging to 3.5 g/kg/day) owing to substantially increased urinary nitrogen losses and increased catch-up growth requirements (~20% higher than the 3 g/kg/day needed to support intrauterine growth rates).
During the acute injury period in children (see Acute Metabolic Stress Response below), it is particularly important to meet daily protein requirements. Current recommendations include 3 to 4 g/kg/day in neonates, 2.5 to 3 g/kg/day in infants, 2 g/kg/day in children and adolescents, and 1.5 g/kg/day in teenagers. Early adequate protein administration increases synthetic rates in tissue with rapid turnover (eg, bone marrow, intestinal endothelium, etc) and promotes endogenous protein sparing during the injury-induced catabolic phase.
Most infant formulas provide a relatively balanced delivery of nonprotein calories, in the range of 45% of total calorie intake each for carbohydrate and fat. In the postnatal period, infant metabolism is characterized by a greater dependence on lipid substrate in addition to carbohydrate substrate for energy needs. There is substantial evidence that premature infants, because of impaired fat absorption by immature gut, may benefit from increased concentrations of medium-chain triglycerides (MCTs) in enteral formulas. Carbohydrates remain important as a source of energy for children and are optimally provided in the form of starches, such as those found in cereals. Generally, standard balanced dietary distribution recommendations include 15% protein, 35% fat, and 50% carbohydrate caloric intake daily.
Factors known to influence metabolic demand include age, gender, the type, amount, and route of macronutrient substrate administration, ambient temperature, body temperature, activity level, and acute metabolic stress. The metabolic rate increases in direct proportion to endogenous (body) temperature. The metabolic rate is dependent on body temperature and has been shown to increase 10% to 13% for each degree centigrade (or 7.2% for each degree Fahrenheit) of body temperature elevation. Tissue injury elicits an acute metabolic stress response, which can have complex and profound effects on energy requirements in children, particularly during infancy. The consideration of critically ill subjects introduces additional variables that include the effects of disease type (ie, trauma vs sepsis) and severity (magnitude and duration) on the extent of the inflammatory response. In addition, the ability of the host to respond to the metabolic injury challenge is effected by conditions that deplete the endogenous metabolic substrate reserve (eg, malnutrition, cancer cachexia, etc) and, thus, further alters energy expenditure. In order to understand how this occurs, the mechanisms of the response, how energy metabolism is affected, how this condition predisposes to excess caloric delivery in critically ill infants, and how to avoid overfeeding are discussed below.
In response to a variety of local or systemic injury stimuli (such as trauma, sepsis, and acute inflammatory conditions), a series of metabolic changes occur that characterize the acute injury response state. Among the early features of the injury response is the release of cytokines, followed rapidly by important alterations in the hormonal environment. Increased counter-regulatory hormone concentrations are associated with insulin and growth hormone resistance. As a result of this response, a sequence of metabolic events is initiated that includes the catabolism of endogenous stores of protein, carbohydrate, and fat to provide essential substrate intermediates and energy necessary to fuel the ongoing response process. Amino acids from catabolized proteins flow to the liver, where they provide substrate for the synthesis of acute-phase proteins and glucose (gluconeogenesis). Therefore, the acute metabolic stress response to injury represents a hypermetabolic, hypercatabolic state that results in the loss of endogenous tissue. Growth, which is an anabolic process, is inhibited during periods of acute metabolic stress.
As the acute metabolic stress response resolves, adaptive anabolic metabolism ensues to restore catabolic losses. In children, this phase is characterized by the resumption of somatic growth.
Insulin is a potent anabolic hormone responsible for glycogen synthesis and the storage of carbohydrate, lipogenesis and the storage of fat, and new protein synthesis. Insulin and insulin-like growth factor 1 (IGF-1) are essential hormones for somatic growth in infants and children. Acute metabolic stress is characterized by substantial increases in serum concentrations of catecholamines, glucagon, and cortisol, which are referred to as counterregulatory hormones because they oppose the anabolic effects of insulin. Serum concentrations of these metabolic stress-related hormones increase as a result of cytokine release.
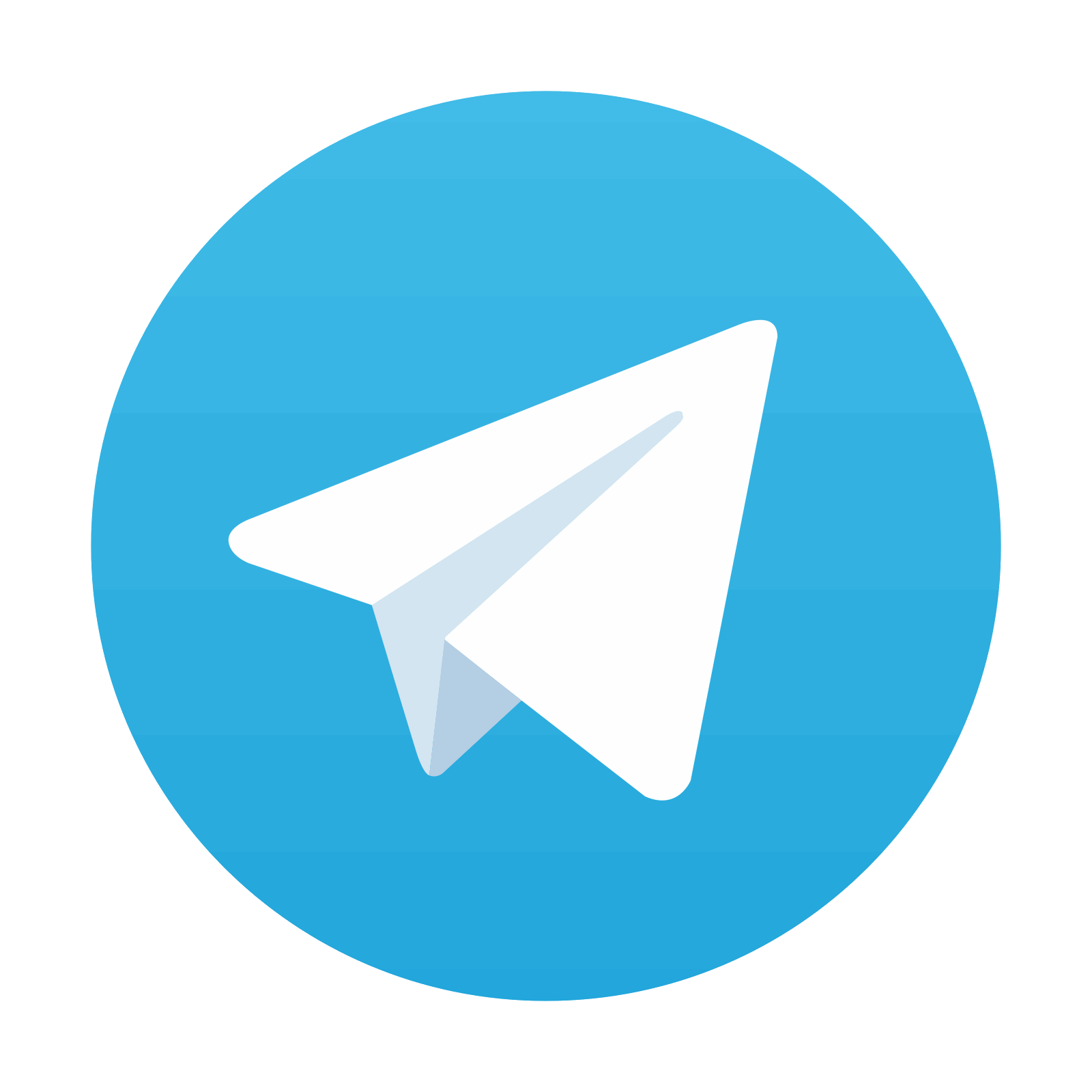
Stay updated, free articles. Join our Telegram channel

Full access? Get Clinical Tree
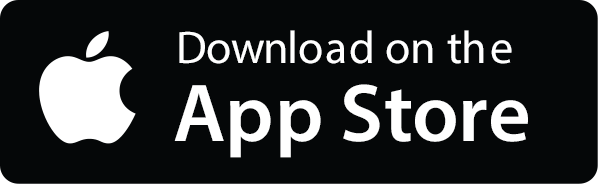
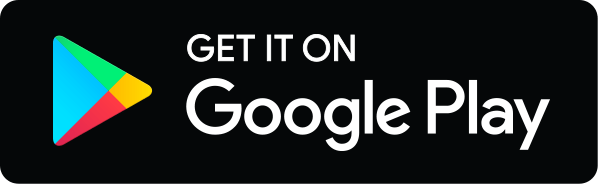