Abstract
Surgical procedures are not infrequent among patients admitted to regional neonatal intensive care units. Adequate nutrition in the newborn period, especially during times of critical illness and “stress,” is necessary to decrease the morbidity and mortality associated with malnutrition. Additionally, many surgical patients are at risk for long-term growth failure and nutritional deficiencies. This chapter aims to address critical elements in understanding the metabolic stress response and the nutritional management of surgical neonates.
Keywords
Metabolism, Nutrition, Stress, Surgical neonate
Introduction
Surgical procedures are not infrequent among patients admitted to regional neonatal intensive care units. It is estimated that 24.1% of referrals are for surgical evaluation and management and that 32% of admitted neonates undergo minor or major operations. Adequate nutrition in the newborn period, especially during times of critical illness and “stress,” is necessary to decrease the morbidity and mortality associated with malnutrition. Additionally, many surgical patients are at risk for long-term growth failure and nutritional deficiencies. This chapter aims to address critical elements in understanding the metabolic stress response and the nutritional management of surgical neonates.
Growth in Surgical Neonates
Somatic growth is an important marker of appropriate nutritional support and the general health of infants and children. Growth has classically been monitored as weight gain over time; however, as more attention has been given to weight gain and nutrition, there has been a shift toward a focus on linear growth and body composition. Linear growth and fat free mass represent protein accretion, lean body mass, and organ development. Inflammation and prolonged illnesses have been proposed to disrupt normal linear growth velocity and therefore are associated with stunting. Surgical neonates represent a special population at risk for growth failure, given the heightened inflammatory response to surgical stress, risk for malabsorption, and other comorbidities, which place them at risk for infection, such as with central venous access. Infants with gastroschisis, congenital diaphragmatic hernia (CDH), and short gut syndrome (SGS) (including infants with gastroschisis, intestinal atresia, and necrotizing enterocolitis [NEC] as primary diagnoses) have all been associated with poor weight gain, linear growth, and head circumference during the first year of life. This is in contrast to the usual presentation of malnutrition, which disproportionately affects weight and typically spares height and head circumference. Linear growth stunting has been identified as an independent predictor of neurodevelopmental delay in premature infants, but few studies have examined the long-term effects of failure to thrive in the surgical cohort of patients.
Surgical Stress Response to Operative Trauma and Critical Illness
After injury or trauma the body undergoes a series of hormonal and metabolic alterations known as the metabolic stress response . This effect has been most extensively studied in adults and has been shown to increase pituitary hormone secretion, sympathetic nervous system stimulation, and activate a proinflammatory cascade. Pituitary hormones, such as adenocorticotropic hormone and arginine vasopressin, are released to initiate catabolism and maintain cardiovascular homeostasis. Neonates are also able to mount a metabolic response after surgical stress that results in increased catecholamines, cytokines, and counterregulatory hormones, such as glucagon and cortisol, which further stimulate catabolism. However, this response is often more severe and of shorter duration compared with that in older children and adults. The increased metabolic response directly opposes the anabolic actions of insulin, growth hormone, and insulin-like growth factor 1. During catabolism, protein from muscle is degraded to release free amino acids for the synthesis of proteins needed for the inflammatory response (cytokines, acute phase reactants) and tissue repair. The remaining carbon skeletons are then converted to glucose through gluconeogenesis in the liver. Factors affecting the surgical stress response include age, fasting status before surgery, degree of operative stress, anesthesia, and the surgical approach.
Energy Requirements During Stress
Energy homeostasis is the balance of energy influx and outflow. Providing the appropriate amount of energy to meet the needs of energy homeostasis is important in the nutritional management of the surgical neonate. Underestimation of energy needs can result in malnutrition, loss of muscle mass, and poor wound healing, whereas overestimation can result in increased risk of infection, liver damage, and hyperglycemia. This may be particularly challenging in the surgical neonate because energy expenditure is variable, making the use of standard predictive equations generally inaccurate.
Total energy expenditure comprises three main components: resting energy expenditure (REE), energy required for physical activity, and diet-induced thermogenesis. Surgical infants are generally considered to be at rest for 80% to 90% of the time; therefore energy needs are primarily dependent on REE.
The gold standard for the measurement of REE is direct calorimetry whereby patients are placed in a thermally isolated chamber that measures dissipated heat for a certain period. It is impractical to use direct calorimetry in hospitalized patients. However, through the use of breath measurements, indirect calorimetry offers a portable and equivalent alternative. Indirect calorimetry measures the amount of oxygen consumed (VO 2 ) and the amount of carbon dioxide produced (VCO 2 ) and then uses a correction factor based on urinary nitrogen excretion, which calculates the overall rate of energy production. Indirect calorimetry also provides the measurement of the respiratory quotient (RQ), which is determined by the ratio of VCO 2 to VO 2 and is a marker of substrate use. The RQ generally ranges from 0.85 to −1.0, with values >1.0 indicating the presence of lipogenesis, typically as a result of overfeeding. It is important to note that indirect calorimetry is not accurate in the setting of air leaks around the endotracheal tube, ventilator circuit, or chest tube, or in those with a fraction of inspired oxygen >0.6.
Indirect calorimetry can be technically challenging to perform; therefore its use should be reserved for special populations of infants. The use of predictive energy expenditure equations, such as the Schofield, Harris-Benedict, or World Health Organization equations, have been proposed but have largely been found to be inaccurate because of individual variation in energy expenditure. As a practical guide, estimated energy requirements for comparable “nonstressed” neonates are used as a starting point to determine energy provisions for the stressed neonate. Generally, newborns require 40 to 70 kcal/kg/day for maintenance metabolism, 50 to 70 kcal/kg/day for growth, and 20 kcal/kg/day for fecal and urinary losses. The total energy requirement of an enterally fed term newborn infant is therefore in the range of 100 to 120 kcal/kg/day. Preterm infants may require even more energy than term infants. Parenterally fed newborns do not have losses of energy or diet-induced thermogenesis and therefore their energy requirement is about 80 to 100 kcal/kg/day. It is recognized that this may be quite inaccurate, and careful monitoring of head circumference, weight, length, and weight for length is therefore necessary.
The REE in surgical neonates is only moderately elevated postoperatively, and this increase is short-lived. Conflicting reports about REE after surgical procedures in neonates have been published ; however, this likely the result of different timings of the studies in relation to surgery and small sample sizes. REE has been shown to increase 15% to 25% by 4 hours after major abdominal surgery in infants and return to normal by 12 to 24 hours postoperatively. Therefore studies occurring >24 hours postoperatively would not show an elevation in REE. However, there is paucity of data regarding REE in neonates who remain critically ill and are dependent on parenteral nutrition for a prolonged time. In adults, REE can be increased by 20% to 80% and remain elevated for >30 days after major trauma to compensate for the energy required for tissue repair. It is thought that the minimal change in energy expenditure seen in infants is a result of diversion of protein and energy normally spent on growth to tissue repair and healing, placing surgical infants at risk for growth retardation during metabolic stress. Careful monitoring of head circumference, weight, length, and weight for length is necessary over time. Surgical neonates may benefit from more exact measurement of their REE by indirect calorimetry to provide the appropriate energy prescription.
Macronutrient Metabolism and Requirements During Stress
Amino acids, carbohydrates, and lipids are the three macronutrients needed for growth and metabolism. The processing of macronutrients changes to support the body’s new metabolic needs after surgery or periods of “stress.”
Nearly 98% of amino acids are found in proteins with the remainder available as free amino acids. Through protein turnover, amino acids are released from protein breakdown and are available for protein synthesis to meet physiologic needs. This dynamic process makes amino acids the most important building blocks used for tissue growth and recovery. Neonates have high protein turnover rates (6-12 g/kg/day) and need to remain in a positive nitrogen balance to achieve appropriate growth and development. This is in contrast to adults, who are not in a period of active growth. Their protein turnover is less (4 g/kg/day), and they remain in a neutral nitrogen balance.
An 80% increase in protein turnover is noted in critically ill neonates and persists throughout the duration of illness. Similarly, infants with cardiopulmonary failure who are supported on extracorporeal life support (ECLS) exhibit nearly double the amount of protein turnover compared with stable patients. The accelerated protein turnover during stress facilitates the redistribution of amino acids from skeletal muscle to injured tissues. The enhanced catabolism of skeletal muscle is the fastest way to provide amino acids and glucose for ill neonates. However, protein stores in neonates are considerably less than in adults, and when protein turnover predominates over synthesis, it places critically ill neonates in a state of negative nitrogen balance. This is clinically evident as skeletal muscle wasting, impaired growth, delayed immune response, and poor wound healing.
Amino acid supplementation improves nitrogen balance in surgical patients by supporting protein synthesis. The minimum amino acid requirement of term surgical patients to prevent protein catabolism is 1 to 1.5 g/kg/day of amino acids; however, infants who received 2.5 g/kg/day had improved nitrogen balance without evidence of protein intolerance. Even higher protein administration may be tolerated in this subgroup of patients, as it is known to be beneficial and safe to provide intravenous (IV) amino acid solutions of 3.5 to 4 g/kg/day to very low–birth weight preterm neonates, but amino acid infusions at this rate have not been studied in the neonatal surgical population. Some neonates who have extensive protein losses through ostomies or who are already severely malnourished may require additional protein supplementation.
Protein provides 4 cal/g of amino acid and therefore is limited in the amount of energy that can be prescribed by protein administration. Carbohydrates in parenteral nutrition also provide 4 cal/g of carbohydrate but can be infused at a higher rate than amino acids. Lipids provide a more concentrated amount of calories at 9 cal/g of lipid. Therefore the oxidation of both carbohydrates and lipids provides the primary sources of energy as adenosine triphosphate, whereas protein is utilized for protein synthesis and growth.
The most important carbohydrate is glucose. Glucose is the primary substrate used in nearly all organs but is of particular importance in the brain, renal medulla, and red blood cells, where it is used as an obligate energy source. Adults are easily able to mobilize glucose from glycogen during times of increased energy requirement. However, glycogen stores are limited in the term neonate and even more so in the preterm population. The limited availability of glycogen necessitates an exogenous supply of glucose, which is commonly administered as D-dextrose, a monohydrate form for IV use. However, during times of stress, endogenous glucose production continues despite administration of IV glucose. Endogenous glucose is produced through glycogenolysis, gluconeogenesis from protein catabolism, and peripheral insulin resistance, which can result in hyperglycemia. Indeed, hyperglycemia has been identified postoperatively in patients with NEC and is associated with increased morbidity and mortality. Currently there are no recommendation regarding the routine administration of exogenous insulin to treat stress-induced hyperglycemia. Insulin administration may be considered when hyperglycemia persists despite reduction in glucose infusion rate (GIR) and correction of other underlying causes of hyperglycemia, such as sepsis. No changes in morbidity and mortality have been seen in postoperative cardiac cases with tight glycemic control. Careful monitoring of blood glucose during provision of insulin therapy is necessary to prevent hypoglycemia. Because of extreme sensitivity to insulin, however, its use in neonates is rare.
Glucose provided in excess of energy needs or in excess of the rate of glucose oxidation (RGO) will be converted to fat through the process of lipogenesis, which may increase the baseline energy expenditure. This process can result in metabolic acidosis, increase in VCO 2 and can induce hepatic steatosis through the inability to export very-low-density lipoproteins at the same rate it is produced. In term surgical neonates and infants on long-term parenteral nutrition, the RGO has been found to be 18 g/kg/day, which corresponds to a GIR of 12 mg/kg/min. Therefore it is not recommended to exceed this amount in supplementation through parenteral nutrition in the surgical neonate.
Energy provided exclusively through carbohydrates has been associated with increased VCO 2 , VO 2 , and energy expenditure. The untoward effects of excess glucose administration can be minimized by diversifying the nonprotein energy source through providing a lipid emulsion. It is recommended to provide 25% to 40% of nonprotein calories as lipids, which generally can be translated to 2 to 4 g/kg/day of IV lipid emulsion. Lipids are calorically dense and isosmotic, and this makes them appropriate to be delivered peripherally if no central access can be obtained. Soy-based lipids provide the essential fatty acids (EFAs) linoleic (an omega-6 fatty acid [FA]) and alpha-linolenic (an omega-3 FA), which are necessary to prevent EFA deficiency, which can occur rapidly in preterm neonates because of limited stores of lipids.
During times of critical illness and stress, lipids are used preferentially in oxidation. Increased lipid metabolism can result in lipid peroxidation and free radical formation, which may be an important mediator in oxygen-induced tissue damage. However, it has been shown that exogenous lipid administration is tolerated during times of stress and does not need to be stopped during times of stress or sepsis. Many have concern regarding the development of hypertriglyceridemia with IV infusion of lipid emulsions and the potential adverse effects of elevated serum triglyceride levels, such as impaired oxygenation from lipid microemboli, altered pulmonary vascular tone, displacement of bilirubin from albumin causing hyperbilirubinemia, and immunosuppression. Monitoring of triglyceride levels could be considered during times of critical illness and adjustments made if lipid accumulation is identified. Many practitioners would consider lipid intolerance at >150 to 250 mg/dL in the preterm neonate. However, there are no data regarding the maximum triglyceride concentrations that would be considered unsafe in a surgical neonate.
One significant side effect of long-term administration of parenteral nutrition is cholestasis, defined as a conjugated bilirubin ≥2 mg/dL. Cholestasis can start as steatosis, progress to bile duct proliferation and fibrosis, and ultimately result in cirrhosis and liver failure. The incidence of cholestasis is >50% in infants maintained on parenteral nutrition for >2 months ; this risk is higher in the surgical subpopulation with SGS, including those with gastroschisis, intestinal atresia, and NEC.
IV lipids have been implicated as a causative agent in the development of parenteral nutrition–associated cholestasis (PNAC). Until recently, the only available lipid emulsion in the United States was derived from soybean oil (Intralipid). Plant-based lipid emulsions contain phytosterols and a greater amount of proinflammatory omega-6 FAs compared with the anti-inflammatory omega-3 FAs. Both the phytosterols and omega-6 FAs have been implicated in the pathogenesis of PNAC. Current strategies in reversing cholestasis include lipid reduction (1 g/kg lipid 1-3 times per week) and advancement of enteral nutrition, if tolerated. However, if lipid reduction is undertaken, it is important to not compensate for decreased caloric intake by increasing the GIR over the RGO (18 g/kg/day) as previously mentioned. This will only increase the RQ to >1.0 and result in lipogenesis. In these cases it may be preferential to allow lower caloric intake. Other strategies that have evolved in recent years include alternative lipid emulsions, including fish oil (Omegaven) and combination oils (SMOF, Clinolipid). Fish oil contains a greater amount of omega-3 FAs compared with omega-6 FAs and do not contain phytosterols. Additionally, fish oil contains a greater amount of alpha-tocopherol, a vitamin E isoform that acts as a potent anti-inflammatory molecule that inhibits lipid peroxidation and oxidative stress. Fish oil has been shown to reverse the effects of cholestasis, but there is insufficient evidence in the prevention of cholestasis. Currently the fish oil emulsion, Omegaven, is only available in the United States through the Food and Drug Administration (FDA) under a compassionate use protocol; therefore it is not widely available. In 2016 the FDA approved the use of SMOFlipid in adults. SMOFlipid is a combination oil composed of 30% soy oil, 30% medium-chain triglycerides, 25% olive oil, and 15% fish oil. It contains intermediary amounts of EFAs, omega-6 FAs, omega-3 FAs, alpha-tocopherol, and phytosterols compared with pure soy and fish oils, but has shown to have some benefit in reversing cholestasis compared with soy oil in small studies. The surgical neonatal subpopulation may benefit from the use of alternative lipid emulsions; however, larger randomized trials are needed to further study safety and efficacy in the neonatal and pediatric populations.
Micronutrient Metabolism and Requirements
Micronutrients are composed of vitamins and trace elements that are essential for many of the metabolic processes in the body. In surgical neonates, the metabolism of these micronutrients may be altered during times of stress, or these infants may experience excessive loss through diarrhea or ostomy output. Essential trace elements include zinc, iron, copper, selenium, manganese, iodide, molybdenum, and chromium. Vitamins can be divided into water soluble (ascorbic acid, thiamine, riboflavin, pyridoxine, niacin, folate, vitamin B 12 ) and fat soluble (vitamins A, E, D, K). These vitamins and minerals are routinely administered through parenteral nutrition while advancing enteral nutrition. Other trace minerals, such as iodine and iron, are not typically provided in parenteral nutrition. In the surgical neonate iron is obtained through periodic blood transfusions or additional supplementation, whereas iodine is absorbed through application of skin disinfectants, such as betadine.
During times of critical illness and hypermetabolism, certain micronutrients, such as vitamins C and E and the trace element selenium, become important as antioxidants neutralizing the effects of reactive oxygen species produced through increased oxidative metabolism. Surgical neonates suffering from diarrhea or excessive ostomy losses may require increased supplementation of micronutrients, specifically zinc and selenium. Selenium deficiency has been associated with an increased risk of infections in neonates. Zinc deficiency has also been associated with predisposition to infection in addition to failure to thrive, poor wound healing, and impaired immune function, making zinc one of the most important micronutrients to the surgical neonate.
Copper and manganese are both excreted through the biliary system and may accumulate in the liver of surgical neonates with hepatic failure from parenteral nutrition–associated cholestasis. This may contribute to toxic levels of copper and manganese, and therefore supplementation with these minerals should be reduced or discontinued when the direct bilirubin reaches >2 mg/dL.
Periodic monitoring of trace vitamins and minerals is recommended in surgical neonates at risk for micronutrient deficiencies or toxicities. Either supplementation or reduction in micronutrients may be required in the surgical neonate.
Fluid and Electrolyte Requirements
Drastic fluid shifts and electrolyte imbalances can be seen in the immediate postoperative period in neonates undergoing surgical interventions. These changes are most evident in neonates who are critically ill and undergoing operations, such as those with NEC. They often experience third spacing of fluid and electrolytes as a result of capillary leak and may develop tachycardia and hypotension requiring fluid bolus administration. These infants require careful monitoring of serum electrolytes (Na + , K + , Ca 2+ , Cl − , HCO 3− , Mg 2+ , P − ) and fluid balance with adjustment in IV fluid components, as needed.
In the surgical neonate, metabolic acidosis can be caused by a variety of mechanisms. Metabolic acidosis can be the result of poor tissue perfusion after surgical procedure, or it can be caused by gastrointestinal (GI) losses of bicarbonate as seen in infants with ostomies. It is important to recognize the cause of the metabolic acidosis because they are treated differently. Surgical intervention in a critically ill neonate may result in hypotension and poor tissue perfusion. This is oftentimes managed by replacing chloride with acetate in IV fluids because hyperchloremia can exacerbate metabolic acidosis. However, in this setting, providing a base will not correct the underlying cause of acidosis and may cause hypercarbia. In this case, providing volume resuscitation and vasoactive medications to improve tissue perfusion may be required. Additionally, acute metabolic acidosis as seen in critically ill neonates causes hyperkalemia through the shifting of intracellular potassium to the extracellular space to buffer hydrogen ions into the cell. Correcting the underlying acidosis will correct the hyperkalemia in most situations, but careful monitoring of potassium is required. Potassium may need to be removed from IV fluids if urine output ceases.
GI losses of electrolytes through ostomy output generally represent more chronic loss rather than critical illness after surgical intervention. These patients require electrolyte replacements and are primarily at risk for sodium and bicarbonate losses. GI fluid and electrolyte losses can be exacerbated by the osmotic load of enteral nutrition known as “dumping” syndrome which may prevent the advancement of enteral nutrition. An average of 3.3 mEq/kg/day of sodium may be lost through normal ostomy output. In cases of extreme sodium losses, aldosterone stimulates absorption of sodium in the proximal renal tubules. This leaves little sodium to participate in the sodium–hydrogen ion exchange in the distal renal tubule, thereby limiting the kidney’s ability to correct the metabolic acidosis caused by GI bicarbonate loss. An equation to assist in calculating sodium supplementation is “Na intake” = 1.2 mEq + (0.13 × ostomy output in mL/kg/day). Another common consequence of sodium deficits and metabolic acidosis is failure to thrive that improves after adequate sodium supplementation with or without bicarbonate supplementation. Patients with ostomies should be monitored closely with measurement of ostomy output, urine sodium, and serum sodium, bicarbonate, and chloride on a regular basis until stable. A urine sodium >10 mEq/L indicates adequate serum sodium reaching the distal renal tubules. Sodium chloride supplementation should be considered if urine sodium <10 mEq/L.
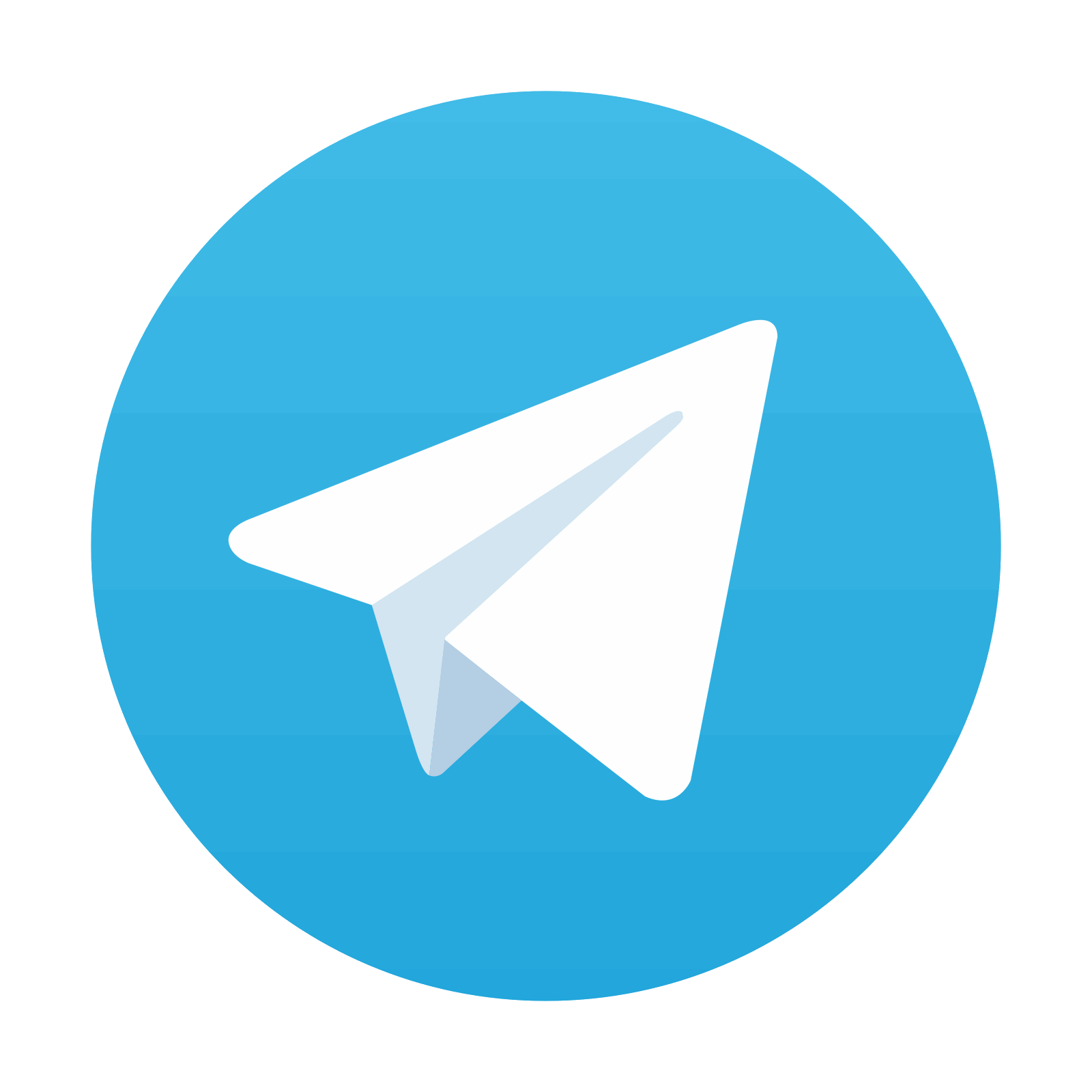
Stay updated, free articles. Join our Telegram channel

Full access? Get Clinical Tree
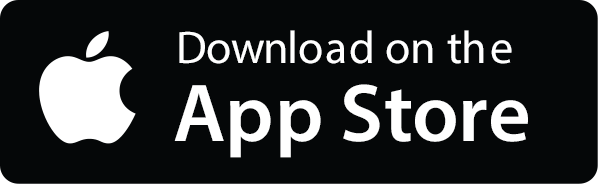
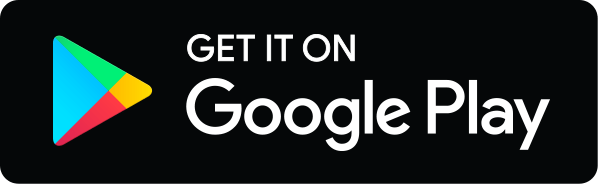