Children’s growth is a hallmark of their normal development and the association between nutrition and linear growth in children is well accepted. Growth requires an adequate supply of many different nutritional factors, some form the “building materials,” whereas others play regulatory roles. In this article we describe the growth of the growth plate and discuss the role of nutritional affected hormones on this process. In addition we describe the effect of local regulators and nutritional factors on the growth process and suggest the involvement of new regulatory factors in the translation of nutrition to growth.
Longitudinal growth in humans is generally divided into four major periods that differ in both the rate of linear gain as well as the major hormones that control it: prenatal period, infancy, childhood, and pubertal period.
The process begins the moment a zygote is created from sperm and oocyte and starts to divide. The zygote becomes embedded in the endometrium to receive essential nutrients through the uterus wall. The most rapid growth during the lifetime takes place in utero, when a complete fetus of about 50 cm in length is produced from a single cell in just 9 months. After birth, the baby continues to grow at a reduced speed during infancy and childhood, followed by a growth spurt during puberty. Thereafter, the growth rate slows, until final height is achieved. This article focuses on the role of nutrition in linear growth in children, without attending to the specific nutritional demands of each period. A detailed description of diseases that lead to insufficient food consumption is beyond the scope of this article.
Growth and development are specific hallmarks of childhood. In most children, they occur spontaneously, with no interruption. A complex system controls the initiation, rate, and cessation of growth. The association between nutrition and linear growth in children is well accepted. The growth of the human skeleton requires an adequate supply of many different nutritional factors. Some, such as proteins, lipids, and carbohydrates, form the “building materials”; others play regulatory roles. Classical nutrient deficiencies lead to stunting (energy, protein, and zinc), rickets (vitamin D), and other bone abnormalities (copper, zinc, vitamin C). However, the exact mechanism whereby nutrition modulates cellular activity during bone elongation is still not known.
Most of the studies that explored the role of specific nutrients in linear growth focused on malnourished children in developing countries. They found that in populations with marginal or poor nutritional status, increased intake of high-protein foods from animal and other sources stimulated weight gain and linear growth. Only a few studies were performed in developed countries, where food is not limited and the daily intake of dietary protein is sufficient to cover physiologic requirements. Moreover, most of the latter focused on children with idiopathic short stature, who are often characterized as poor eaters and lean, or on patients with eating disorders. Thus, their data need to be interpreted accordingly.
Linear growth is the product of an elaborate cascade of events that takes place in the cartilaginous growth center of the long bones, the epiphyseal growth plate (EGP). It involves the sequential replacement of chondrocytes of the EGP by osteoblasts, a process regulated by complex interactions among hormones, local growth factors, and components of the extracellular matrix, namely collagens and proteoglycans. Endochondral ossification begins with the proliferation of early chondrocytes, followed by their alignment in columns, and finally, their maturation into hypertrophic chondrocytes. The hypertrophic cells then cease dividing and increase in volume by 5- to 10-fold by the ingress of water ( Fig. 1 ). They begin to secrete extracellular matrix (ECM) consisting of collagen type X, as well as matrix vesicles (small particles that serve as centers of mineralization). Thereafter, the chondrocytes undergo programmed cell death, with calcification of the ECM, enabling the invasion of blood vessels and osteoblasts. The cartilage scaffold is thus replaced with bone tissue. This reorganization of the ECM is essential for proper maturation of the EGP. The hormones and local factors that control these events may be directly or indirectly affected by the nutritional status.

In this article, we describe the evidence-based knowledge gained to date on the effect of food on specific growth-stimulating hormones ( Fig. 2 ). In addition, we describe the effects of nutrition on the growth plate, with a focus on growth-related genes and suggest the involvement of novel factors such as sirtuins and microRNAs. We also describe the current knowledge on the effect of energy, vitamins, and micronutrients on growth, as shown in epidemiologic studies in humans.

Hormones
Growth Hormone: Insulin-like Growth Factor-I
During childhood, linear growth is predominantly regulated by Growth Hormone (GH), a 191-amino acid polypeptide synthesized and secreted by somatotrophs of the anterior pituitary gland. Besides promoting linear growth, GH also exerts metabolic effects on numerous tissues and organs. Its release from the pituitary is regulated by a complex interplay of hypothalamic, pituitary, and circulating factors. In the hypothalamus, GH secretion is stimulated by GH-releasing hormone (GHRH) and inhibited by somatostatin.
GH affects growth directly, by binding to its receptors in the growth plate, and also indirectly, via insulin-like growth factor (IGF)-I, a 70-amino acid peptide. GH stimulates many body tissues to produce IGF-I, especially the liver, thereby increasing circulating IGF-I levels and the local production of IGF-I in the EGP.
IGF-I serves as both the main mediator of GH action and as a GH-independent growth factor. Its direct effect on growth is best exemplified in utero: fetuses with deficient IGF-I production or a defect in the IGF-I receptor show significant growth delay, whereas fetuses with GH deficiency do not. Mice studies of targeted mutagenesis of the genes encoding IGF-I and its receptor, IGF-IR, found that IGF-I −/−mice had a birth weight of 60% of their wild-type littermates, and IGF-IR −/−mice had a birth weight of 45% of normal. The IGF-IR −/−mice all died immediately postpartum from respiratory failure. Human inactivation mutations in the IGF-I gene are also associated with growth retardation. All the human mutations in the IGF-IR gene identified so far are associated with severe intrauterine growth retardation (IUGR) (length deficit of –0.3 to –5.8 SDS depending on the specific mutation).
The mediatory effect of IGF-I on GH activity comes into play postnatally. IGF-I acts through its receptor, IGF-IR, which is expressed on all tissues except the liver, including cells of the EGP. It stimulates cell proliferation and differentiation and protects cells from apoptosis. In the EGP, IGF-I stimulates growth and differentiation in a spatial-dependent fashion.
Multiple studies have shown that GH and IGF-I concentrations are responsive to changing nutritional status and intake of amino acids and free fatty acids. Fasting induces a GH-resistant state, although its exact effect on the GH/IGF-I axis remains unclear. Whereas fasting increased serum GH levels in humans, rabbits, sheep, cows and pigs, it reduced serum GH levels in mice and rats; nevertheless, in all animals examined, IGF-I levels were reduced. The effect was more pronounced with protein restriction. Down-regulation of either systemic or local IGF-I production was assumed to play a role.
Accordingly, fasting was found to lead to a decrease in the rate of longitudinal bone growth and reduced length of the EGP. Heinrichs and colleagues reported an increase in GH receptor (GHR) and IGF-I mRNA levels in rabbits after short fast, whereas our group noted that mice subjected to 40% food restriction for 10 days showed a dramatic reduction in the level of GHR in the EGP. This finding suggested GH resistance at the receptor level may be as a result of increased circulating levels of glucocorticoids. It is possible that the decrease in plasma IGF-I and local GHR is part of an adaptive mechanism by the body to shunt calories away from nonessential processes, including growth, during periods of malnutrition.
Ghrelin
Nutritional status also affects levels of Ghrelin, a 28-amino acid peptide identified less than a decade ago that serves as the endogenous ligand for the GH secretagogue receptor (GHSR). Ghrelin is mainly expressed in the stomach, but also in other tissues, such as the hypothalamus and placenta. Its major function is the central regulation of food intake: ghrelin levels increase preprandially and under cachectic conditions and significantly decrease within 1 hour after eating. It has therefore been named “the hunger hormone.” Ghrelin not only induces an increase in food consumption, it also apparently shifts food preference toward high-fat diets.
In vivo and in vitro studies showed that ghrelin, in its acylated form, releases GH by binding to its hypothalamic receptor, GHSRα 1. Interestingly, this receptor has significant ligand-independent activity. Its physiologic importance was suggested by findings that natural mutations segregated with short stature in several families. Functional analysis revealed a disruption of the constitutive activity of the receptor, with no effect on binding of the ligand. The short stature in these cases may have been caused by reduced activity at the hypothalamic-pituitary-GH axis. Recently mutations in GHSR were identified in children with constitutional delay of growth and puberty. These mutations showed a reduction in cell surface expression, with the p.Ser84Ile mutation also associated with a defect in ghrelin potency. However, a large genetic study in a French population failed to find evidence of a major contribution of common variants of the ghrelin and GHSR genes to height variations. Moreover, in several animal models, knock out of either ghrelin or its receptor genes was not associated with any attenuation of growth.
GHSR levels are stimulated by fasting, and high levels of IGF-I inhibit pituitary GHSR mRNA levels. By contrast, neither total nor octanoylated ghrelin increased during fasting in parallel to the massive increase in GH secretion.
Although specific ghrelin activity in the growth plate has not yet been identified, a direct effect was suggested by findings that ghrelin is synthesized by growth plate chondrocytes.
Leptin
We summarized the role of leptin in growth in a recent publication ; thus, only a brief review is presented here.
Leptin, a hormone predominantly produced by adipocytes, was first identified as the product of the Ob gene in 1994, in studies of leptin-deficient obese (Ob/Ob) mice. It was originally described as a circulating hormone involved in feeding behavior and energy homeostasis. However, the wide distribution of the Ob receptor (ObR; LEPR) in different tissues indicated that besides body weight regulation, leptin had numerous peripheral effects, including bone growth and remodeling.
In children, the involvement of leptin in growth was supported by a series of studies suggesting that periods of fast growth such as fetal life and adolescence require a certain level of leptin.
A direct link between leptin and linear growth was suggested by findings that leptin administration to Ob/Ob mice corrected their metabolic abnormalities and also led to a significant increase in femoral length. Leptin was also found to directly stimulate GH secretion. Others reported lower levels of GH in both leptin-deficient Ob/Ob mice and humans with a mutation of the leptin receptor. In addition, in an animal model of catch-up growth consisting of 40% food restriction for 10 days followed by food replenishment, we observed that weight gain associated with an increase in the level of serum leptin preceded tibial catch-up growth.
To address the role of leptin on linear growth, several groups followed its effects in vivo and ex vitro and noted a stimulatory effect on growth-plate cartilage proliferation and differentiation. In our studies, leptin was shown to exert its effects on chondrocytes in the EGP through its active, long-form, receptor and possibly through the activation of the PTHrP/Ihh axis.
In mice models, leptin administration led to reduced food consumption and reduced body weight. However, normal mice treated with repeated subcutaneous leptin injections had longer tibia than pair-fed controls. Leptin stimulation of the EGP was balanced, positively affecting both proliferation and differentiation, so that the ratio between proliferating and hypertrophic chondrocytes remained constant. These results were supported by the study of Martin and colleagues wherein leptin stimulated femoral length and the midshaft cortical area independently of peripheral IGF-I. Furthermore, leptin administration to rat with intrauterine growth retardation significantly improved structural properties and elongation rate of bones.
Fasting experiments performed in rodents reported a drop in GH and IGF-I serum levels in response to a reduction in food consumption. Leptin administration to the fasted rats restored their blunted GH secretion but failed to increase their serum IGF-I levels. These results again indicate that leptin can act as a metabolic signal connecting adipocyte tissues with the GH axis and that its stimulatory effect on growth under conditions of food restriction is not dependent on circulating IGF-I.
Although leptin-deficient mice have impaired linear growth, Farooqi and colleagues described a family with a mutation in leptin in which the index patient and her affected cousin were both tall. These observations may suggest that the effect of leptin in humans is different from that observed in rodents. At the same time, the scarce information on human subjects with leptin abnormalities should be taken into account.
Insulin
Insulin, a 51-amino acid beta-cell–specific hormone, is secreted from the pancreas in response to increased glucose levels and binds to its receptors on peripheral cells and tissues to enable the assimilation of glucose into cells. Insulin was the first hormone in the central nervous system that was implicated in the control of body weight. Findings of severe IUGR in babies with pancreatic agenesis or mutations in the insulin receptor gene suggest an essential role for insulin signaling in normal intrauterine growth. Extreme insulin resistance as a result of a mutation in the insulin receptor leads to leprechaunism, a congenital disorder characterized by insulin resistance, fasting hypoglycemia, and severe pre- and postnatal growth retardation. The outcome may be caused by an insufficient energy supply to the cells or lack of insulin activity on chondrocytes of the growth plate.
Other evidence for the role of insulin in fetal growth comes from children with mutations in the gene encoding for glucokinase (GCK), which catalyzes the rate-limiting step in glycolysis and serves as a pancreatic β-cell glucose sensor. Mutations in GCK result in altered glucose sensing and decreased insulin secretion. Children with a mutation in GCK are approximately 500 g smaller than unaffected siblings.
In children with type I diabetes, the lack of adequate insulin levels may lead to growth failure if the disease is chronically under poor control. However, the growth failure in general is modest, and it probably represents a combination of calorie wasting, chronic acidosis, and increased glucocorticoid production characteristic of other chronic diseases as well. In most cases, there is no correlation between glycemic control and skeletal growth, and many children with apparently marginal control appear to grow well.
Energy/calories/protein
Protein-energy malnutrition (PEM) is the most important nutritional disease in developing countries because of its high prevalence and its relationship with child mortality rates and impaired physical growth and higher cognitive development. Children with chronic PEM were found to perform poorly on tests of attention, working memory, learning and memory, and visuospatial ability; only scores on tests of motor speed and coordination were not affected.
Children with marasmus (a form of malnutrition caused by long-lasting insufficient caloric intake) and kwashiorkor (a form of malnutrition caused mainly by insufficient protein consumption) had significantly lower body weight and height than healthy subjects, as well as reduced levels of serum leptin, insulin, and IGF-I.
Animal studies clearly demonstrated the deleterious effects of PEM on linear growth, but in humans, it was somewhat difficult to dissociate the effect of the nutritional and other environmental factors or to ascertain the irreversibility of the nutritional damage. In the presence of infection, PEM induced greater loss of nutrients or led to metabolic alterations. In children with idiopathic short stature from developed countries, where plenty of variable food is available, calorie intake was positively correlated with growth velocity both before GH treatment and during the first year of GH treatment. Children with eating disorders from developed countries were on average shorter than controls; this effect was independent of age of onset. Several specific amino acids, such as the amino acids arginine and lysine, which are known to stimulate GH\IGF-I secretion, might have an important role in linear growth. Unfortunately, studies exploring the role of specific amino acids in growth are sparse. One recent observational study, which explored the association between arginine and lysine intake and linear growth in children, found that high arginine intake was positively associated with linear growth among girls. No such association was found for lysine.
Anorexia nervosa (AN), characterized by voluntary marked food restriction, could potentially serve as an excellent model to follow the effect of PEM on growth. AN has a female predominance, with an estimated prevalence of 1% in young women. Adolescents may present with short stature and delayed pubertal development or secondary amenorrhea. These abnormalities normalize with weight gain, although the effect of AN on final height is not clear. In a study performed in male adolescents, who account for 5% to 10% of all patients with AN, growth failure was a prominent feature, and weight restoration resulted in accelerated growth in all those with growth retardation. Weight gain was necessary for catch-up growth, but the weight had to be gained before the ability to grow was lost with age. By contrast, in two studies performed in girls, height potential was preserved. Some authors suggested that the discrepancy among studies was attributable to AN-associated hypogonadism or to the fact that by the time of disease onset in girls, that is, around age 13 to 14 or 17 to 18, most have already or nearly completed growth.
In several animal models, even short-term (48- to 72-hour) fasting caused a decrease in the rate of longitudinal bone growth. The height of the growth plate, the number of proliferative and hypertrophic chondrocyte per column, and the height of terminal hypertrophic chondrocytes were all reduced, with no disorganization ( Fig. 3 ). This immediate growth cessation may have important implications also for children, although in most cases recovery was rapid.

Energy/calories/protein
Protein-energy malnutrition (PEM) is the most important nutritional disease in developing countries because of its high prevalence and its relationship with child mortality rates and impaired physical growth and higher cognitive development. Children with chronic PEM were found to perform poorly on tests of attention, working memory, learning and memory, and visuospatial ability; only scores on tests of motor speed and coordination were not affected.
Children with marasmus (a form of malnutrition caused by long-lasting insufficient caloric intake) and kwashiorkor (a form of malnutrition caused mainly by insufficient protein consumption) had significantly lower body weight and height than healthy subjects, as well as reduced levels of serum leptin, insulin, and IGF-I.
Animal studies clearly demonstrated the deleterious effects of PEM on linear growth, but in humans, it was somewhat difficult to dissociate the effect of the nutritional and other environmental factors or to ascertain the irreversibility of the nutritional damage. In the presence of infection, PEM induced greater loss of nutrients or led to metabolic alterations. In children with idiopathic short stature from developed countries, where plenty of variable food is available, calorie intake was positively correlated with growth velocity both before GH treatment and during the first year of GH treatment. Children with eating disorders from developed countries were on average shorter than controls; this effect was independent of age of onset. Several specific amino acids, such as the amino acids arginine and lysine, which are known to stimulate GH\IGF-I secretion, might have an important role in linear growth. Unfortunately, studies exploring the role of specific amino acids in growth are sparse. One recent observational study, which explored the association between arginine and lysine intake and linear growth in children, found that high arginine intake was positively associated with linear growth among girls. No such association was found for lysine.
Anorexia nervosa (AN), characterized by voluntary marked food restriction, could potentially serve as an excellent model to follow the effect of PEM on growth. AN has a female predominance, with an estimated prevalence of 1% in young women. Adolescents may present with short stature and delayed pubertal development or secondary amenorrhea. These abnormalities normalize with weight gain, although the effect of AN on final height is not clear. In a study performed in male adolescents, who account for 5% to 10% of all patients with AN, growth failure was a prominent feature, and weight restoration resulted in accelerated growth in all those with growth retardation. Weight gain was necessary for catch-up growth, but the weight had to be gained before the ability to grow was lost with age. By contrast, in two studies performed in girls, height potential was preserved. Some authors suggested that the discrepancy among studies was attributable to AN-associated hypogonadism or to the fact that by the time of disease onset in girls, that is, around age 13 to 14 or 17 to 18, most have already or nearly completed growth.
In several animal models, even short-term (48- to 72-hour) fasting caused a decrease in the rate of longitudinal bone growth. The height of the growth plate, the number of proliferative and hypertrophic chondrocyte per column, and the height of terminal hypertrophic chondrocytes were all reduced, with no disorganization ( Fig. 3 ). This immediate growth cessation may have important implications also for children, although in most cases recovery was rapid.
Molecular mechanisms regulating the interaction between nutrition and growth
Regulatory factors involved in the translation of energy within cells may be important for linear growth. These include hypoxia-inducible factor (HIF) 1, mammalian target of rapamycin (mTOR), epigenetic mechanism, and microRNAs (miRNAs). The involvement of the last two in regulation of growth is only suggested and was not shown in growth plates yet. It will be interesting to follow upcoming developments in these fascinating new fields.
Hypoxia-inducible Factor 1
To study the effect of food restriction on gene expression, analyses were performed on a diversity of animals and tissues. Although no common gene affected by food restriction was identified across the different species, several shared factors were found, including metabolism and cell growth, regulation of transcription, energy metabolism, and stress and immune functions. To the best of our knowledge, the only study that analyzed genes in the EGP itself is our own. We showed that in rats, 40% food restriction for 10 days induced dramatic changes in the expression of several genes. One of them was HIF1α, a key subunit of HIF, which serves as a master transcription factor regulating the expression of several genes that code for proteins involved in angiogenesis, cell metabolism, proliferation, motility, adhesion, and survival.
HIF1α is responsible for the adaptation of chondrocytes to the low oxygen pressure of the avascular and relatively hypoxic tissue in which they are located. Its significance to chondrocyte survival, especially in the hypoxic regions of the embryonic EGF, and its involvement in chondrocyte proliferation, differentiation, and growth arrest, are well recognized. These studies demonstrated the importance of tight regulation of HIF1 levels during development of the prenatal growth plate. HIF1 was also found to be a major factor in anaerobic glycolysis, which supplies most of the energy requirements of the chondrocytes. In addition it is required for extracellular matrix production which is essential for proper growth and development, in cultured proliferating chondrocytes, by up-regulating the expression of the cartilage transcription factor Sox9 and by regulating the enzymes responsible for the hydroxylation of collagen prolines (P4HaI and P4HaII), and the enzyme lysyl oxidase, which is responsible for the formation of cross links between collagen molecules.
These findings indicate that nutrition has a profound effect on the level of gene expression within the growth plate during longitudinal growth and that HIF1α plays an important role in growth of the mature plate growth in response to nutritional manipulation.
Mammalian Target of Rapamycin
Cells have a complex sensing system designed to ensure that they do not undergo periods of growth unless adequate levels of nutrients are available to produce the energy necessary to support that growth. mTOR is an evolutionarily conserved serine/threonine protein kinase, which, like HIF1, serves as a key regulator of cell metabolism. It is activated in the presence of adequate levels of nutrients (glucose, amino acids, lipoproteins, minerals), turning on the cell’s translational machinery for the synthesis of proteins that are essential for its growth and other functions. Activated mTOR stimulates angiogenesis, which increases the number of blood vessels through which nutrients can reach the cell. In addition, it increases the production of nutrient transporter proteins, which enhance the cell’s ability to import essential nutrients, and stimulates HIF1α expression and glycolysis. When nutrient levels are inadequate, mTOR is inactivated, protein synthesis is inhibited, cell growth is arrested, and autophagic protein degradation takes place.
mTOR is found in the form of two multiprotein complexes, mTOR Complex 1 (TORC1) and mTOR Complex 2 (TORC2). TORC1 is sensitive to the cellular nutritional state, and it targets the phosphorylation of proteins that regulate protein translation, gene expression, and autophagy. TORC2, by contrast, does not respond to changes in nutritional conditions but has been implicated in cytoskeleton regulation.
Among the most studied substrates of TORC1 are the eukaryotic initiation factor 4E (eIF4E), binding protein (4E-BP), and ribosomal protein S6 kinase (S6K). 4E-BP is a translational inhibitor that is deactivated by TORC1 phosphorylation; S6K is a positive translational effector activated on phosphorylation. By activating ribosomal S6K and inhibiting 4E-BP, mTOR initiates translation.
TORC1 is regulated by insulin and nutrients, including glucose and amino acids, particularly leucine, as well as a variety of cellular stresses. In some cell types, amino acids can activate mTOR alone; in others, they collaborate with growth factors, such as insulin. In the absence of amino acids, growth factors are helpless.
Modulation of mTOR signaling was shown to stimulate chondrocyte differentiation. TOR, together with HIF1α, is also involved in the autophagy of the maturing chondrocytes of the EGP. Autophagy is induced under energy-restricted environmental conditions and inhibited by nutrient sufficiency. It plays a role in the control of several physiologic processes. Specifically, in response to nutrient deprivation, the cells degrade the cytosolic content by the formation of a double-walled vesicular structure that eventually fuses with lysosomes, so that energy can be generated from the cells’ own protein and lipid stores. Nutrient-stimulated activation of the TOR protein kinase leads to the phosphorylation and inactivation of components of the autophagy pathway.
Vps34 (vacuolar protein sorting 34), a member of the PI3K family of lipid kinases, also participates in nutrient signaling to mTOR. It is inhibited by amino acid deprivation and up-regulated with mTOR signaling.
In a recent study, maturing chondrocytes were found to exhibit an autophagic phase. Its regulation was dependent on the activities of mTOR and AMP kinase in response to the AMP/ATP ratio in the cells. When AMP kinase activity was blocked, autophagy could not be activated. Thus, nutrient insufficiency may increase the autophagic response in the growth plate chondrocytes, reducing the size of the cells and growth plate and leading to growth attenuation. When the restriction is short, this process may be reversible, but when it is prolonged, cell number may be reduced and growth stunted.
The involvement of the following two systems was not shown in growth plate yet; however, we hypothesize that these systems may serve as potential links translating nutrition to growth .
Sirtuins
Sirtuins, class III histone deacetylases (HDAC), are highly conserved enzymes that use nicotinamide adenine dinucleotide (NAD+) to deacetylate a number of histone and nonhistone substrates. The founding member of this family, silent information regulator 2 (Sir2), promotes longevity in yeast by repressing gene expression and stabilizing chromatin. Mammals have seven Sir2 homologs (SIRT1 to SIRT7), which are involved in regulating cell survival and stress response. Specifically, SIRT1 and SIRT6 are implicated in the response to calorie restriction (CR). Studies found that SIRT1 was induced in vitro by nutrient deprivation and in vivo after long-term CR. Cells cultured in the presence of serum from CR rats showed an attenuation of stress-induced apoptosis and an increase in SIRT1 expression. The enzymatic activity of SIRT1 appears to be positively regulated by NAD+, which increases during CR and fasting. Mice overexpressing SIRT1 exhibited similar physiologic properties to mice on a CR regimen. SIRT1 may regulate cell proliferation, senescence, and apoptosis by regulating several transcription factors that govern metabolism and endocrine signaling, including PPAR-γ, PGC-1α, FOXOs, and p53. Levels of SIRT6, whose absence in mice causes genome instability and the premature appearance of aging-related pathologies, were also found to increase in rats subjected to prolonged CR, in mice after 24 hours of fasting, and in cell culture after nutrient depletion. The role of sirtuins in the EGP has not been reported.
MicroRNAs
On completion of the Genome Project, researchers recognized that a large part of the genome is not translated into proteins. This noncoding, so-called “junk” DNA, has recently been found to be highly relevant to the regulation of gene expression and the maintenance of genomic stability. Some of it is transcribed into small non–protein-coding RNAs, or microRNAs (miRNAs), measuring approximately 19 to 23 nucleotides in length, which negatively regulate the expression of a large portion of the protein-encoding and non–protein-encoding genes at the posttranscriptional level ( Fig. 4 ). Each miRNA can regulate one to several mRNA transcripts, and conversely, a single mRNA may be regulated by one to several miRNA sequences. Genomic computational analysis indicates that as many as 50,000 miRNAs may exist in the genome. Cumulative laboratory data have confirmed the presence of hundreds of these miRNAs in the genomes of animals, plants, and viruses.
The miRNAs are transcribed by RNA polymerase II–producing primary (pri)-miRNAs, which vary greatly in size, from a few hundred bases to tens of thousands. Mature miRNAs are derived from two major processing events driven by sequential cleavages by the RNAse-III enzymes, Drosha and Dicer. The result is a small double-stranded (ds) miRNA duplex that is quickly unwound by a helicase and the single mature strand is asymmetrically incorporated into the RNA-induced silencing complex (RISC) to guide it to its target. This is accomplished by base-pairing of the mRNAs with their complementary miRNA binding sites, most of which are thought to lie in the 3′ untranslated region (UTR) of the target. RISC then acts by translational repression (cleavage-incompetent RISC) or mRNA degradation (cleavage-competent Slicer containing RISC). The degree of inhibition is often correlated with the number of miRNA binding sites. The major advantage of the miRNA regulatory system is its tiny size: it is much more rapidly expressed than a typical protein-coding primary transcript, and it is not further delayed by splicing and translation. In mammals, miRNAs have been shown to regulate numerous systems, among them adipocyte differentiation, insulin secretion and B-cell development, neural stem cell fate, immune function and cellular metabolism ; miRNA dysregulation is associated with several diseases, including cancer.
Recently it was shown that miRNA-375, a beta-cell–specific microRNA, regulates glucose-induced insulin secretion, suggesting a link between nutrition and microRNA. Others identified miR-140 to be chondrocyte-specific in zebrafish. In mice, miRNA-140 was detected specifically in cartilaginous tissues of the developing limbs, ribs, vertebrae, sternum, and skull. Binding sites for miRNA-140 were predicted in numerous genes, including some known to play a role in chondrogenesis, such as vascular endothelial growth factor A, matrix metalloproteinase (MMP) 13, basic fibroblast growth factor 2, platelet-derived growth factor and many more. In addition, miRNA-140 is known to target HDAC4, an essential element in chondrocyte hypertrophy in mice limbs. Roles for Dicer, and several additional miRNA including miR-1, miRNA-196, miR-199a, and miR-675 in skeletal morphogenesis have also been reported.
These results show that miRNA may be responsive to nutritional cues and that microRNAs are involved in chondrogenesis; thus, it seems reasonable to suggest that microRNA may serve as mediators translating nutritional signals to processes in the EGP.
Effect of specific nutritional factors
The role played by amino acids and glucose in growth has been extensively studied. What about other components of our daily meals? Do specific food ingredients have specific beneficial effects, or are they all alike?
Vitamins
Vitamin D
Vitamin D is known to be involved in calcium homeostasis. Calcium is required for normal growth and development and maintenance of the skeleton. Vitamin D regulates endochondral ossification in a cell-maturation–dependent manner via nuclear vitamin D receptor (VDR) as well as ERp60, a membrane-associated 1α25 (OH) 2 D 3 -binding protein. Both resting zone and growth-zone cells possess enzymes involved in the metabolism of 25(OH)D 3 , and they produce and secrete the 24R,25(OH) 2 D 3 metabolite and the 1α25(OH) 2 D 3 metabolite into their extracellular environment. They also harbor nuclear receptors for 1α25(OH) 2 D 3 and respond to it by a decrease in number and proliferation. Cells in the resting zone respond primarily to 24R,25(OH) 2 D 3 , and cells in the prehypertrophic and upper hypertrophic zones respond primarily to the 1α25(OH) 2 D 3 . The vitamin D hormone 1α25(OH) 2 D 3 binds with high affinity to the VDR, which recruits its retinoid X receptor heterodimeric partner to recognize the vitamin D–responsive elements in target genes. VDR also selectively binds certain omega3/omega6 polyunsaturated fatty acids (PUFA) with low affinity, leading to transcriptionally active VDR-RXR complexes. The cell maturation–specific actions of the metabolites affects ECM synthesis and turnover, including matrix composition with release and activation of latent factors such as TGF-β, all of which fine tune the rate and extent of chondrocyte proliferation and hypertrophy.
Numerous association studies have dealt with single-nucleotide polymorphisms (SNPs) in the coding and intronic regions of the human VDR gene; however, only one study showed a clinical association between two SNPs located upstream of the transcriptional start site of the main human VDR gene promoter and height. These authors found that adolescent girls with a CC/GG genotype had lower circulating levels of 25-(OH)D, with no detectable consequence on calcium metabolism, in addition to lower serum levels of IGF-I and shorter stature from age 11 years to achievement of adult height. Interestingly, however, in the absence of vitamin D or in the presence of a malfunctioning receptor, rickets rather than short stature is described. Furthermore, a recent study shows that pharmaceutical doses (given for example to children with CKD) of calcitriol, the 1,25 vitamin D inhibited intra-cortical bone formation and elongation.
Vitamin A
Vitamin A and its derivative, retinoic acid, were suggested to promote the differentiation of pituitary cells to secret GH. At the same time, retinoic acid was shown to inhibit the growth of metatarsals in vitro, by inhibiting chondrocyte proliferation, hypertrophy, and matrix synthesis. Results of epidemiologic studies are also confusing: some observational studies reported significant correlations between vitamin A status and stunting, a recent study shows that six months of vitamin A, iron and zinc supplementation induces growth acceleration in short children born SGA with subnormal nutrients intake similar to growth hormone therapy. In contarst but others concluded that vitamin A supplementation had little or no impact on linear growth. A recent study in mice also failed to demonstrate any effect of vitamin A deficiency on longitudinal growth. Further studies are required to fully unravel the role of vitamin A in growth.
Micronutrients
Several micronutrients have been investigated for their potential influence on linear growth, namely, zinc, iron, copper, iodine, calcium, phosphorous, and magnesium. Observational or animal studies suggested that children with stunted growth are deficient in these micronutrients, but there is as yet no confirmatory evidence-based data for any of them, apart from zinc and iron.
Zinc
Studies of zinc have so far provided the most conclusive evidence linking the intake of a specific micronutrient to child growth. However, the mechanism by which zinc deficiency impairs growth has not been elucidated. Some authors reported that zinc supplementation led to a highly significant increase in height and weight, with a greater growth response in children with initially low Z-scores for weight or height, especially those known to be zinc deficient. By contrast, when zinc was administered to children with idiopathic short stature and normal serum zinc levels there was no effect on height or weight SDS. In addition, treatment of infants with nonorganic failure to thrive was associated with an increase in serum IGF-I levels, but not with an improvement in growth parameters.
Researchers speculated that the effect of zinc on growth was mediated by its effect on IGF-I levels. Zinc deficiency reduces IGF-I production and may also decrease cellular IGF-I responsiveness, and transient partial GH deficiency due to zinc deficiency was reported in children. Zinc supplementation was found to increase basal levels of IGF-I, IGFBP-3, alkaline phosphatase, and osteocalcin, without changing GH levels or increasing subjects’ sensitivity to exogenous GH. Others reported that Zinc supplementation led to a significant increase in growth rate and GH levels. Additionally, through its influence on the GH/IGF-I system, zinc deficiency has been observed to affect bone metabolism. In our study, zinc increased levels of IGF-I but not of IGFBP3.
Zinc deficiency was shown to cause morphologic and pathologic changes in the growth plate, leading to disorganization of the chondrocytes of the EGP and shorter tibias and femurs in rats. This effect may be attributable to malfunctioning of zinc-containing nucleoproteins, transcription factors, or MMPs. The latter are a group of zinc-dependent endopeptidases that play an important role in catabolism and turnover of the matrix and are crucial during bone growth and development. Deficiency of either of the most abundant MMPs (MMP-9, 13, 14) in mice led to phenotypes of severe skeletal dysplasia. Both MMP-9 and VEGF are regulated by vitamin D. Thus a balanced regulation of MMPs is essential for proper EGP growth.
In conclusion, zinc appears to be an important linear-growth–limiting micronutrient. Further studies are needed to clarify the underlying mechanism of its action in this setting.
Iron
Several observational studies documented a relationship between iron-deficiency anemia and impaired physical growth. The proposed mechanisms include its effect on immunity, appetite, thermogenesis, and thyroid hormone metabolism. However, a clear causal inference was precluded in these studies owing to the presence of several confounders, as follows: (1) Coexistent parasitic infections, which cause iron deficiency, may also impair growth. (2) Children who grow rapidly may have more depleted iron stores, whereas those with slow growth may appear iron-replete. (3) Concomitant deficiencies of other macronutrients and micronutrients, such as zinc, may also affect linear growth. Two recent reviews of the literature concluded that iron interventions had no significant effect on child growth, with similar results obtained across categories of age, duration of intervention, mode and dosage of intervention, and baseline anthropometric status. Some authors suggested that the effect of iron on growth was supported by the findings that zinc has a positive stimulatory effect on growth only when iron levels are adequate. However, others failed to show a beneficial effect of these supplements on growth, possibly because of concurrent deficiencies of other micronutrients. The fact that most of the studies were conducted in developing countries, where food supply is limited, should be considered when interpreting the clinical meaning of the results.
Combinations of Micronutrients
The treatment of children with a mixture of micronutrients for 1 year had small but significant impact, with a greater benefit in those of low and medium socioeconomic status. The supplements contained at least vitamin A, iron, zinc, B vitamins, and folic acid, in addition to iodine, vitamin C, vitamin E, calcium, potassium, copper, and other trace vitamin and minerals.
Milk
The unique role of milk in newborns led many researchers to study its effect on linear growth also in older children. Milk is an important source of nutrients supporting growth, such as high-quality proteins containing all essential amino acids, high lactose content which seems to support growth due to a pro-biotic effect and improved absorption of minerals, and minerals, such as potassium, magnesium, phosphorus and zinc. Whole milk is also a good source of energy and has a good balance between energy and protein, which is important for optimal utilization of proteins. An extensive review by Hoppe and colleagues suggested that milk has a significant growth-stimulating effect. Their conclusion was based on observational and interventional studies in developing countries, as well as observational studies of well-nourished populations. However, in the well-nourished populations, the association between milk and linear growth was less clear, and some interventional studies had negative findings. The effect of milk on growth may not be the same in all age groups. In a study using data from the NHANES 1999-2002, milk consumption was a predictor of height in age groups 12-18 years but not in age group 5-11 years. Cow’s milk may have the strongest effects in children with existing undernutrition.
According to one hypothesis, milk promotes linear growth by stimulating circulating IGF-I levels. However, although some studies reported supportive findings, others did not.
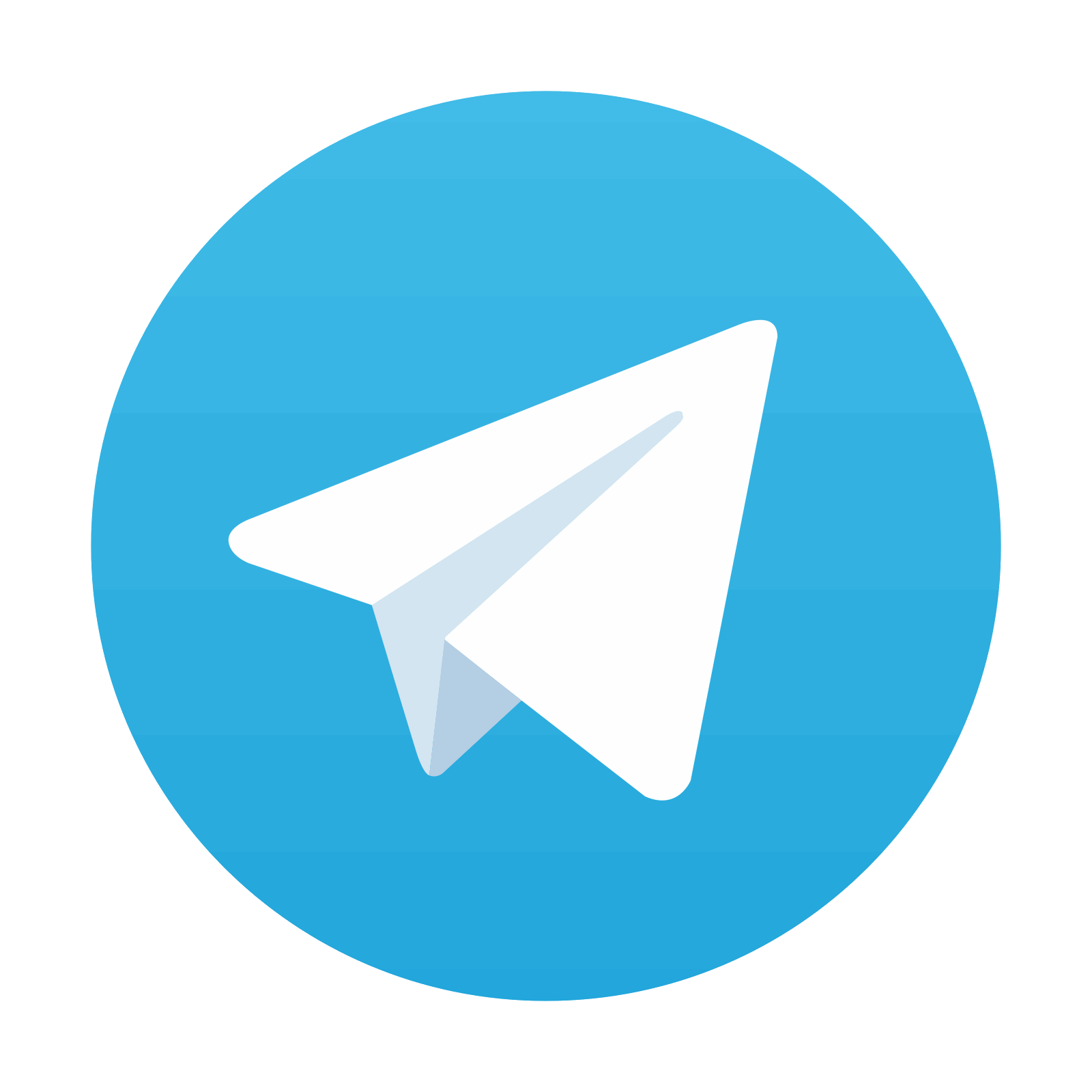
Stay updated, free articles. Join our Telegram channel

Full access? Get Clinical Tree
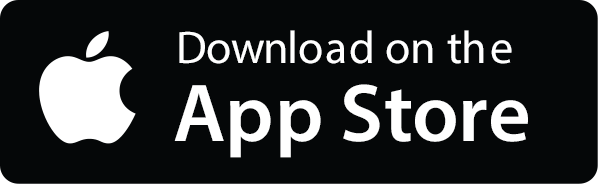
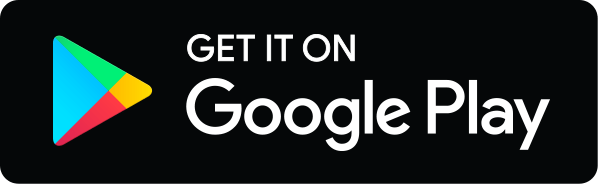