Nutrient Supplements
Mary Frances Picciano
Michelle K. McGuire
Paul M. Coates
Introduction
During infancy and childhood, patterns of healthy growth and development are generally predictable and orderly. Although these growth trajectories are primarily determined by genetics, environmental influences such as nutritional status can also be important. This is especially true during the most rapid periods of growth, such as those which occur in infancy and adolescence. A good example of this is protein. During early infancy, protein requirements are estimated to be 1.52 g per kg per day; this decreases gradually through childhood until it reaches 0.8 g per kg per day (1). Although obtaining adequate amounts of the essential nutrients from foods during periods of growth is considered most desirable, sometimes this becomes difficult, and supplementation is warranted. This is especially true for several of the micronutrients (vitamins and minerals), which are the main focus of this chapter.
Vitamins and minerals are essential for myriad functions related to growth, differentiation, and development. Vitamins can also regulate the expression of genes involved in cell proliferation and tissue specification. Minerals primarily act as enzymatic cofactors and stabilizers for enzymes and transcription factors, although some are also critical for structural purposes. Because of the far-reaching functions of vitamins and minerals, micronutrient deficiencies can have negative impacts during periods of growth and development as well as long-term effects on structural integrity. Early malnutrition may also predispose an individual to increased risk for chronic degenerative diseases (e.g., cardiovascular disease) later in life.
Because of the importance of nutritional adequacy during early life, dietary recommendations for the pediatric population are relatively well delineated, and supplementation with vitamins and minerals is sometimes used to prevent or treat nutrient inadequacies (2). The main objective of this chapter is to review a selected group of micronutrients that are most frequently provided to infants and children as dietary supplements. These include vitamin A (and the carotenoids), vitamin D, vitamin E, vitamin K, calcium, iron, zinc, iodine, and fluoride. We will also comment briefly on a group of lipids referred to as the “omega-3 fatty acids.” For each of these, we will discuss their biological forms and functions, dietary sources, dietary reference intake (DRI) values, signs and symptoms of deficiencies and toxicities, and the most current recommendations for supplementation. We will also review the definition of a dietary supplement, how these products are regulated by the Food and Drug Administration, and statistics related to supplement use—especially in children.
What is a Dietary Supplement?
Although dietary supplements have long been available to the US consumer, they were not officially defined until 1994 when the US Congress passed the Dietary Supplement Health and Education Act (DSHEA) (3). Since that time, a “dietary supplement” has been defined as a product that meets the following requirements:
-
Intended to supplement the diet or contain one or more of the following: vitamin, mineral, herb or other plant-derived substance (e.g., ginseng, garlic), amino acid, concentrate, metabolite, constituent, or extract.
-
Intended for ingestion in pill, capsule, tablet, or liquid form.
-
Not represented for use as a conventional food or as the sole item of a meal or diet.
DSHEA was the first legislation to clearly define dietary supplements as foods (as opposed to drugs) having implications for both manufacturers and consumers in terms of what was needed (or not needed) for evidence of efficacy, safety, and quality of dietary supplement products. For example, similar to how food products are handled, manufacturers are required to have evidence to support claims of efficacy and safety. However, unlike what pharmaceutical companies are required to do for drugs, food manufacturers are generally not required to seek premarket approval of their claims by the Food and Drug Administration.
Dietary Supplement Use in American Children
Use of dietary supplements in the United States is strong and growing, and it is estimated that revenue from sales of dietary supplements was more than $21 billion in 2005 (4). Of the dietary supplements available, vitamins and minerals—as well as combinations thereof—are the most widely used types. Most (65%) of these supplements are purchased from retail establishments such as natural, health food, grocery, or drug stores; only 7% are obtained from a health practitioner.
Of special interest to this chapter is dietary supplement use among children. Ervin and colleagues compiled data from the National Health and Nutrition Examination Survey conducted between 1988 and 1994 (before the passage of DSHEA) and reported that children aged 1 to 5 years were major users of dietary supplements (5). The supplements most commonly taken by children aged 2 months to 11 years were multivitamins or combinations of multiple vitamins and minerals. Slightly less than 10% of children were taking two or more supplements.
Dietary supplement use by infants and children between 1999 and 2002 (after passage of DSHEA) has also been reported (6). In this study, the investigators used nationally representative data from the National Health and Nutrition Examination Survey, studying children (n = 10,136) from birth through 18 years. Their data indicate that 31.8% of children used dietary supplements, with the lowest use reported among infants younger than 1 year (11.9%), followed by teenagers 14 to 18 years (25.7%). Highest use was among 4- to 8-year-old children (48.5%). In an ethnic comparison, supplement consumption was found to be greatest in non-Hispanic white and Mexican American participants; lowest use was among non-Hispanic black participants. There appeared to be no difference in intake between boys and girls. These analyses suggest that the type of supplement most commonly used by children is a multivitamin/multimineral combination. Furthermore, supplement use was reported to be associated with higher income, a smoke-free environment, lower body mass index, and less daily recreational “screen time.” The highest use was in children who were underweight or at risk for becoming underweight.
In summary, the use of dietary supplements and more specifically those containing vitamins and minerals is common in the pediatric American population. In the next sections, we discuss several micronutrients that are sometimes recommended as dietary supplements during infancy and childhood. We address their physiological importance, recommended intakes, signs and symptoms of deficiency and toxicity, and—when possible—recommendations for supplementation.
Vitamin a and the Carotenoids
The term “vitamin A” (or retinoid) refers to a group of fat-soluble compounds that all contain a complex 20-carbon structure with a methyl-substituted cyclohexenyl ring and a tetrene side chain. These compounds differ among themselves in that they can contain a hydroxyl group (retinol), aldehyde group (retinal), carboxylic acid group (retinoic acid), or ester group (retinyl ester).
The term “vitamin A” is also used to describe a series of dietary compounds (called carotenoids) that can be converted in the body to retinol, some of which are shown in Figure 56.1. These dietary substances are also called “provitamin A carotenoids” to distinguish them from other carotenoids that cannot be converted to vitamin A. Carotenoids, which usually contain 40 carbon atoms with conjugated double bonds and one or two cyclic structures, function mainly as antioxidants. Of the 600 or more carotenoids that exist in nature, β-carotene, α-carotene, and cryptoxanthin are the primary provitamin A forms. There is growing interest in both nonprovitamin A and provitamin A carotenoids, because higher dietary intakes of some forms have been associated with decreased risk for macular degeneration and cataracts, some cancers, and some cardiovascular events (7).
Metabolism of Vitamin A
Regardless of whether it is consumed as preformed vitamin A or a provitamin A carotenoid, retinoids are metabolized in many organs (liver, intestine, kidney, and skin), being converted to different forms with specific functions. For example, retinal can be reversibly reduced to retinol and irreversibly oxidized to retinoic acid (8). Retinoids, bound to retinoid-binding proteins and receptors, are transported to specific sites for metabolic transformations both within the plasma and the cell.
Physiologic Roles of Vitamin A
Perhaps the most thoroughly understood function of vitamin A (specifically retinal and retinoic acid) in the body is the role it plays in vision. Specifically, retinal is required for the transduction of light into neural signals necessary for vision, whereas retinoic acid is needed to maintain normal differentiation of the cornea and conjunctional membranes. However, vitamin A is also needed for a variety of other functions as well. For instance, during embryonic development, retinoic acid as well as specific retinoid receptors (retinoic acid receptor and retinoid X receptor) and retinol-binding proteins are postulated to be involved in the development of the vertebrae and spinal cord. Retinoic acid also functions in limb development and formation of the heart, eyes, and ears (8).
Retinoic acid also appears to be involved in the genesis of tissue specificity, directing undifferentiated stem cells to stop proliferating and assume a differentiated phenotype (8). In addition, retinoic acid regulates the expression of various genes that encode structural proteins involved in maintaining epithelial cell integrity (e.g., skin keratins), extracellular matrix proteins (e.g., laminin), and retinol-binding proteins and receptors. Retinoic acid is also needed to maintain adequate circulating levels of natural killer cells, increase macrophage phagocytic activity, and increase cytokines that mediate T and B lymphocyte production (9).
Dietary Sources of Vitamin A
Preformed vitamin A is found in some animal-derived foods such as liver, dairy products, and fish. Provitamin A carotenoids tend to be plentiful in colorful fruits and vegetables, such as cantaloupe, carrots, broccoli, spinach, and some oily plants (e.g., avocado and red palm oil). The primary dietary form of provitamin A is β-carotene, which is cleaved to form free retinol in the small intestine. Note that the vitamin A content of foods or biological tissues is measured using a unit referred to as a retinol activity equivalent (RAE); 1 RAE is equivalent to 1 μg of all-trans-retinol, 2 μg of supplemental all-trans-β-carotene, 12 μg of dietary all-trans-β-carotene, or 24 μg of other dietary provitamin A carotenoids.*
Dietary Reference Intake Values for Vitamin A
DRIs have been set for vitamin A during infancy. Like all of the adequate intake (AI) levels for young infants (0 to 6 months), that for vitamin A is based on the vitamin A intake of infants primarily fed human milk. The AI for this essential nutrient from 0 to 6 months is 400 μg of RAE per day; from 7 to 12 months it is 500 μg of RAE per day. Recommended dietary allowances (RDAs) for children aged 1 to 3 and 4 to 8 years are 300 and 400 μg of RAE per day, respectively, after which time they increase. Because vitamin A toxicity can occur, tolerable upper intake levels (ULs) have also been set. These values are 600 μg of RAE per day for the first 3 years of life; 900 μg of RAE per day from 4 to 8 years; 1,700 μg per day from 9 to 13 years; and 2,800 μg per day from 14 to 18 years. The AI and UL values for vitamin A (as well as the other micronutrients discussed in this chapter) during infancy are listed in Table 56.1; the AI/RDA and UL values during childhood and adolescence can be found in Table 56.2.
Consequences of Inadequate Vitamin A Intake and Effect of Supplementation
Because human milk, bovine milk, and infant formulas are good sources of vitamin A, vitamin A deficiency in the United States is rare in infancy and childhood. However, vitamin A deficiency can occur in infants and children with fat malabsorption (2). Furthermore, vitamin A concentration in milk from mothers delivering prematurely is very low and may not provide recommended amounts (10). Thus, vitamin A nutriture may be inadequate in this subpopulation, even in developed countries.
The most common signs and symptoms of vitamin A deficiency progress from night blindness to xerosis (dryness and wrinkling of the conjunctiva) and the development of Bitot’s spots, to corneal xerophthalmia, keratopathy, and finally loss of vision. In developing countries (especially Asia and Africa) vitamin A deficiency not only is the leading cause of preventable blindness but also elevates the risk of disease and death from severe infections such as diarrhea and measles (11,12). For example, children with mild xerophthalmia are at increased risk of respiratory tract infections and diarrhea, and mortality rates are up to four times greater among children with than without mild xerophthalmia. Experts estimate that between 100 and 140 million children are vitamin A deficient worldwide, 250,000 to 500,000 of whom become blind annually; half of these affected children are thought to die within 12 months of losing their sight (13). Other factors in developing countries that can influence a child’s requirement for vitamin A are presence and severity of infection with parasites, inadequate iron status, low intakes of dietary fat, and protein energy malnutrition.
Fortunately, high-dose vitamin A supplementation can reduce the incidence of morbidity, including night blindness in children (13). However, vitamin A supplementation may be able to reverse the process at its early stages (14). There is also growing evidence that the risk of severe morbidity and mortality diminishes with vitamin A repletion. For example, a meta-analysis of controlled trials in several developing countries suggests a 23% to 30% reduction in mortality of young children older than 6 months after vitamin A supplementation (15). Similarly, a recent randomized trial conducted in Bangladesh showed a 15% reduction in all-causal mortality when newborns received a single, oral supplement of vitamin A (50,000 IU) at birth (16).
Vitamin A supplementation may also help prevent mortality and morbidity in very low–birth-weight (VLBW) infants. In support of this, a recent Cochrane review utilized data from eight randomized controlled trials which compared the effects of supplemental vitamin A with standard vitamin A regimes in VLBW infants (17). These authors concluded that supplementing VLBW infants with vitamin A was associated with a reduction in death or oxygen requirement at 1 month of age and oxygen requirement among survivors at 36 weeks, with the latter outcome being confined to infants with birth weight less than 1,000 g.
Table 56.1 Representative Values for Nutrient Content of Mature Human Milk and Reference Intake Values for Selected Nutrients During Infancy and Childhood | ||||||||||||||||||||||||||||||||||||||||||||||||||||||||||||||||||||||||||||||||||||||||||||||||||||||||||||||||||||||||||||||||||||||||||||||||||||||
---|---|---|---|---|---|---|---|---|---|---|---|---|---|---|---|---|---|---|---|---|---|---|---|---|---|---|---|---|---|---|---|---|---|---|---|---|---|---|---|---|---|---|---|---|---|---|---|---|---|---|---|---|---|---|---|---|---|---|---|---|---|---|---|---|---|---|---|---|---|---|---|---|---|---|---|---|---|---|---|---|---|---|---|---|---|---|---|---|---|---|---|---|---|---|---|---|---|---|---|---|---|---|---|---|---|---|---|---|---|---|---|---|---|---|---|---|---|---|---|---|---|---|---|---|---|---|---|---|---|---|---|---|---|---|---|---|---|---|---|---|---|---|---|---|---|---|---|---|---|---|
|
Improvement in vitamin A status of preterm infants is also associated with decreased incidence of and morbidity from bronchopulmonary dysplasia, indicating that vitamin A supplementation may prevent lung injury and promote healing (18). Vitamin A supplementation may be especially beneficial for extremely low–birth-weight infants. Ambalavanan and colleagues determined whether vitamin A supplementation in extremely low–birth-weight infants (n = 807) during the first month after birth affects survival without neurodevelopmental impairment (19). Although they found no evidence that neonatal vitamin A supplementation reduced hospitalizations or pulmonary problems after discharge, those provided with supplementation had reduced bronchopulmonary dysplasia.
Many recent studies have reported positive effects of vitamin A supplementation on overall infant and child health, especially in areas of endemic vitamin A deficiency, although others show no effect and sometimes even detrimental outcomes (20,21,22). An example is a randomized clinical trial of 147 severely anemic children living in Zanzibar who were being treated with the antimalarial drug sulfadoxine pyramethamine (23). The authors hypothesized that both vitamin A (100,000 or 200,000 IU depending on age) and sulfadoxine pyrimethamine treatments rapidly stimulate erythropoietin production. However, while it did reduce inflammation (as measured by C-reactive protein) and mobilized iron from stores, vitamin A supplementation decreased erythropoietin concentrations. There is also evidence of a differential response to vitamin A supplementation between boys and girls (24,25), and this interaction between vitamin A supplementation and sex on vitamin A-related outcomes warrants further study.
Because low vitamin A status has been shown to be a risk factor for maternal-to-child human immunodeficiency virus (HIV) transmission, there has also been considerable interest in whether vitamin A supplementation may be beneficial. Several intervention trials have been conducted, some of them (but not all) suggesting a positive outcome of supplementation (26). However, unease about potential negative outcomes associated with large-dose maternal or neonatal vitamin A supplementation on infant mortality in HIV-endemic areas is considerable (27). Emerging data suggest that there may exist a gene–environment interaction between having the mannose-binding lectin (MBL-2) gene and vitamin A intake in terms of HIV transmission during infancy (28).
Table 56.2 Dietary Reference Intakes (Per Day) of Nutrients Likely to be Limiting in Diets of Childrena | |||||||||||||||||||||||||||||||||||||||||||||||||||||||||||||||||||||||||||||||||||||||||||||||||||||||||||||||||||||||||||||||||||||||||||||||||||||||||||||||||||||||||||||||||||||||||||||||||||||||||||||||||||||||
---|---|---|---|---|---|---|---|---|---|---|---|---|---|---|---|---|---|---|---|---|---|---|---|---|---|---|---|---|---|---|---|---|---|---|---|---|---|---|---|---|---|---|---|---|---|---|---|---|---|---|---|---|---|---|---|---|---|---|---|---|---|---|---|---|---|---|---|---|---|---|---|---|---|---|---|---|---|---|---|---|---|---|---|---|---|---|---|---|---|---|---|---|---|---|---|---|---|---|---|---|---|---|---|---|---|---|---|---|---|---|---|---|---|---|---|---|---|---|---|---|---|---|---|---|---|---|---|---|---|---|---|---|---|---|---|---|---|---|---|---|---|---|---|---|---|---|---|---|---|---|---|---|---|---|---|---|---|---|---|---|---|---|---|---|---|---|---|---|---|---|---|---|---|---|---|---|---|---|---|---|---|---|---|---|---|---|---|---|---|---|---|---|---|---|---|---|---|---|---|---|---|---|---|---|---|---|---|---|---|---|---|---|---|---|---|
|
Consequences of Excessive Intake of Vitamin A
Commonly recognized signs and symptoms of vitamin A toxicity (hypervitaminosis A) in infants and young children include skeletal abnormalities, bone tenderness and pain, increased intracranial pressure, desquamation, brittle nails, mouth fissures, alopecia, fever, headache, lethargy, irritability, weight loss, vomiting, and hepatomegaly (29). As little as 1,800 μg RAE per day can produce serious toxic effects in children. Although vitamin A toxicity is typically associated with excessive consumption of supplemental vitamin A, toxic effects have also been reported in young children fed large amounts of chicken liver daily (90 μg per g RAE) for 1 month or longer (30).
Experimental data indicate that β-carotene, even when ingested in extremely large doses, is not carcinogenic, mutagenic, embryotoxic, or teratogenic. Instead, it results in a benign disorder referred to as hypercarotenemia, characterized by elevated concentrations of carotenoids in serum and liver and carotenoid deposits in skin and underlying subcutaneous adipose causing the skin to become yellowish orange in color (31).
Recommendations for Vitamin A Supplementation During Infancy and Childhood
The International Vitamin A Consultative Group, the World Health Organization (WHO), and the United Nations Children’s Fund recommend oral doses of vitamin A for infants and young children in vitamin A-deficient populations. Specifically, the International Vitamin A Consultative Group recommends that three 50,000 IU doses of vitamin A should be given at the same time as infant vaccines during the first 6 months of life in all populations where vitamin A deficiency is an important public health problem (32). High-dose vitamin A treatment is also recommended for infants and young children with xerophthalmia, severe malnutrition, or measles. The WHO recommends broad-based prophylaxis with vitamin A in vitamin A-deficient children who suffer from xerophthalmia, measles, prolonged diarrhea, wasting malnutrition, and other acute infections. Specifically, if a child shows any ocular signs of deficiency, it is recommended that he/she receive vitamin A on days 1, 2, and 14, and then repeatedly throughout the first year of life (33). The American Academy of Pediatrics (AAP) does not make any special recommendations concerning dietary supplementation with vitamin A during infancy and childhood other than those associated with using appropriate human milk fortifiers and proprietary formulas when needed. However, there is some evidence that supplemental vitamin A may be indicated for low–birth-weight (LBW), preterm infants (10,34).
Vitamin D
Vitamin D (technically referred to as calciferol) and its metabolites constitute a group of fat-soluble sterols that are essential for proper growth and development of the skeletal system. Vitamin D exists in two major physiologically relevant forms: vitamin D2 (ergocalciferol) and vitamin D3 (cholecalciferol) (Fig. 56.2). Ergosterol, the precursor to vitamin D2, is synthesized by plants; 7-dehydrocholesterol, the precursor of vitamin D3, is synthesized by humans and other mammals in their skin. Conversion of both precursors to active vitamin D initially requires cleavage via ultraviolet B light (UVB; 290 to 320 nm). It is because of this that vitamin D is sometimes referred to as the “sunshine vitamin” and why vitamin D deficiency is more common in areas of the world where exposure to sunlight is limited (e.g., Scandinavian countries). The availability and metabolism of vitamin D is complex, involving endogenous synthesis, sunlight exposure, dietary intake, and biotransformation to active and inactive metabolites (Fig. 56.2). Briefly, circulating vitamin D3 is converted in the liver to 25-hydroxyvitamin D3 and then again hydroxylated principally in the kidney to the biologically active form, 1,25-dihydroxyvitamin D3 (calcitriol).
Physiologic Roles of Vitamin D
Vitamin D has many roles in the body, although the most well studied relates to its importance in skeletal growth and maturation. For instance, the active metabolite (calcitriol) functions as a hormone along with parathyroid hormone (PTH) in small intestine, bone, and kidney tissues to maintain serum calcium and phosphate concentrations within physiologically optimal ranges. In addition to enhancing dietary absorption of calcium and phosphorus in the small intestine, 1,25-dihydroxy-vitamin D3 functions with PTH to mobilize calcium from bone and increase renal resorption of calcium when blood calcium is low. Other recently recognized functions of vitamin D include immunomodulation, control of other hormone systems, inhibition of cell growth, and induction of cell differentiation (35,36,37).
Sources of Vitamin D and Factors Affecting Vitamin D Status
As described, the body can synthesize vitamin D given adequate precursor material and sunlight. In this way, vitamin D is often considered a conditionally essential nutrient or prohormone, instead of an essential nutrient. Nonetheless, vitamin D can be obtained from dietary sources and is therefore a nutrient. Like vitamin A, the amount of vitamin D in a food or biological material is measured by the amount of biological activity it provides. In the case of vitamin D, the IU, the biological activity of 1 μg of vitamin D is said to be 40 IU (38). One IU of vitamin D activity is equivalent to 5 ng of 25-hydroxyvitamin D3 and 1 ng of 1,25-dihydroxyvitamin D3.
Egg yolks, butter, whole milk, fatty fish, fish oils, and mushrooms are some of the few foods that naturally contain vitamin D, and even these foods are not especially rich sources. Therefore, obtaining an adequate intake of vitamin D solely from nonfortified foods is seldom possible (39). However, most liquid and dried milk products as well as breakfast cereals are fortified with this vitamin, and in fact most dietary vitamin D comes from these foods in the United States. Human milk is relatively low in vitamin D (0.33 μg per L IU) unless the mother is given supraphysiologic doses of the vitamin (40).
Dietary vitamin D intake can influence an individual’s vitamin D status. However, perhaps more than any other nutrient, other environmental and lifestyle factors are also important. One of these factors is latitude (38). Changes in season and dark skin pigmentation also affect vitamin D status. Compared with their lighter-skin counterparts, individuals with darker skin require longer periods of sun exposure to produce similar amounts of vitamin D.
Reference Intake Values for Vitamin D and Recommendations for Solar Exposure
The AI for vitamin D during infancy, childhood, and adolescence is 5 μg per day (Tables 56.1 and 56.2). Experts now agree that the dietary intake of vitamin D recommended to prevent vitamin D deficiency in normal infants cannot be met with human milk as the sole source of vitamin D. The same is likely true for dietary sources for children and adolescents. Thus, additional vitamin D must be obtained from endogenous production (via sunlight exposure) and/or supplements. Recommendations for solar exposure are discussed here, whereas recommendations for supplementation are provided in a subsequent section.
The amount of solar exposure needed to furnish adequate vitamin D is difficult to define because the amount of UVB light that penetrates skin depends on many factors such as latitude, time of day, level of air pollution, extent of skin pigmentation, sunscreen usage, and habitual dress. Nonetheless, Specker et al. suggested sun exposure of 30 minutes per week was adequate for white infants wearing only a diaper and living in Cincinnati, Ohio (USA), or 2 hours per week if they were fully clothed without a hat (41). However, dietary or supplemental sources of vitamin D become essential during the winter months at northern latitudes because intensity of and exposure to UVB radiation is insufficient to meet the needs for endogenous synthesis.
Because vitamin D toxicity can occur, ULs for this nutrient have also been set. Based on measurement of linear growth (42) and hypercalcemia (43), the UL for infants is 25 μg (1,000 IU) of vitamin D per day for the first 12 months of life; this doubles to 50 μg of vitamin D per day for children 1 to 18 years of age (38) (Tables 56.1 and 56.2).
Consequences of Inadequate Vitamin D Status
Vitamin D deficiency during early life can result in a serious disease called rickets, which results from inadequate synthesis of 1,25-dihydroxyvitamin D3. Rickets is characterized by impaired skeletal mineralization at the epiphyseal growth plates, causing deformities and poor linear growth of the long bones. Clinical signs of advanced rickets include craniotabes, frontal skull bossing, rachitic rosary (enlarged costochondral junctions), widened ribs, bowed legs, and muscle weakness. Subclinical vitamin D deficiency occurs months before signs of rickets become obvious and is assessed by measuring plasma 25-hydroxyvitamin D3 concentration. Vitamin D deficiency later in life can result in osteomalacia, and emerging evidence suggests that low vitamin D status may be a risk factor to the development of various chronic diseases including cardiovascular disease, hypertension, diabetes mellitus, some inflammatory and autoimmune diseases, and cancer (44,45,46).
Until recently, rickets resulting from vitamin D deficiency was believed to be almost nonexistent in the United States. However, many cases of rickets due to low vitamin D intake and inadequate endogenous synthesis in the skin have recently been reported in the United States as well as in other Western countries such as Canada, Greece, and the United Kingdom (47,48,49,50,51). Vitamin D-deficiency rickets is also common in breastfed infants of Arab and south Asian countries (52). One recent estimate suggests that the annual incidence of vitamin D–deficiency rickets in developed countries ranges between 2.9 and 7.5 cases per 100,000 children (53). Other data suggest that lesser degrees of vitamin D insufficiency are also widespread (54). Vitamin D–deficiency rickets has also been reported recently in adolescence, thus making the issue of vitamin D deficiency crucial during the entire period of growth (55,56,57).
Infants and children with dark skin pigmentation, living at northern latitudes, or who are exclusively breastfed are at greatest risk for developing vitamin D deficiency. Vitamin D–deficiency rickets is also more common in children whose mothers observe the Islamic custom of covering their entire bodies with clothing; practice prolonged breastfeeding without vitamin supplementation or addition of vitamin D–fortified dairy products in their infants’ diet; or do not themselves consume vitamin D-fortified dairy products.
Consequences of Excessive Vitamin D Intake
Large oral doses of vitamin D lead to excessive calcium absorption by the intestine, increased bone resorption, and hypercalcemia. This condition, referred to as hypervitaminosis D, is characterized by elevated levels of 25-hydroxyvitamin D3 ranging from 160 to 500 ng per mL (38). Hypercalcemia associated with hypervitaminosis D disrupts normal kidney function, resulting in polydipsia and polyuria. Anorexia, nausea, and vomiting have also been reported in individuals taking 50,000 to 200,000 IU of vitamin D per day. It is noteworthy that doses of 1,800 to 6,300 IU of vitamin D per day were reported decades ago to inhibit linear growth (38). However, that finding was not confirmed in a later study conducted over a longer period of time and with a larger number of infants (42).
Recommendations for Vitamin D Supplementation
Because of growing concerns of widespread vitamin D deficiency in infants and children—especially those with dark skin—several organizations now recommend routine vitamin D supplementation in these groups. Data from studies conducted in China, the United States, and Norway show that consumption of at least 200 IU of vitamin D per day prevents clinical signs of vitamin D deficiency and maintains serum 25-hydroxyvitamin D3 at or above 27.5 nmol per L (11 ng per mL). Indeed, this value is consistent with the current AI for vitamin D during infancy (38). However, it is clear that 25-hydroxyvitamin D3 concentrations of more than 50 nmol per L can be maintained in exclusively breastfed infants with supplements of 400 IU of vitamin D per day, which is the amount contained in one teaspoon of cod liver oil and for which there is historic precedence of safety and prevention and treatment of rickets (58,59,60).
In light of the AAP recommendation that infants be kept out of direct sunlight and wear protective clothing and sunscreen when exposed to sunlight (61), it is important that special efforts be directed toward supplementing populations at increased risk of developing rickets and vitamin D deficiency. Therefore, the AAP recommends that all breastfed infants be supplemented with 400 IU of vitamin D per day beginning within the first few days of life (62). Formula-fed infants receiving less than 1 L of vitamin D–fortified formula per day also need an alternative way to get 400 IU of vitamin D per day, such as through vitamin supplements. This level of supplementation should be continued through childhood. Adolescents who do not obtain 400 IU of vitamin D per day through vitamin–D fortified milk (100 IU per 8 oz serving) and vitamin D–fortified foods should receive a vitamin D supplement of 400 IU per day.
Vitamin E
The term “vitamin E” refers to all tocol and tocotrienol chemical derivatives that exhibit biological activity similar to that of α-tocopherol, the most abundant and active form of vitamin E. Vitamin E occurs in eight naturally occurring forms: four tocopherols and four tocotrienols (Fig. 56.3). As shown in Figure 56.3, tocopherols have similar chromanol structures, which vary only in the number and position of methyl groups on the chromanol ring: trimethyl (α), dimethyl (β or #979;), and mono (δ). Tocotrienols differ from tocopherols in that they have an unsaturated side chain.
The most biologically active form of vitamin E occurs naturally in foods and thus is often referred to as “natural” α-tocopherol or more technically as RRR-α-tocopherol.† Synthetic vitamin E is a mixture of eight stereoisomers of α-tocopherol and is designated all-rac-α-tocopherol (often termed d,l-α-tocopherol). Compared with RRR-α-tocopherol other stereoisomers of all-rac-α-tocopherol have lower (21% to 90%) biologic activity. Absorption of α-tocopherol from natural and synthetic sources is similar. Synthetic vitamin E is preferentially degraded over the natural forms; thus, it is postulated that RRR-α-tocopherol has a specific regulatory role in a system that sorts, distributes, and degrades the different forms of vitamin E (63).
![]() Figure 56.3. Parent forms of tocopherols and tocotrienols. (Modified from Traber MG, Sies H. Vitamin E in humans: demand and delivery. Annual Rev Nutr 1996;16: 321–347, with permission.) |
Physiologic Roles of Vitamin E
Vitamin E has many functions in the body, the first recognized being that of reproduction. In fact, the name tocopherol was derived from the Greek tokos (childbirth) and phero (to bear). In general, this vitamin functions as a chain-breaking antioxidant to prevent free radical damage (64). Specifically, vitamin E typically carries out its antioxidant function within membranes. This antioxidant protection is especially important in cells that are exposed to oxygen, such as those in the lungs and red blood cells. Recent evidence also suggests a protective effect of vitamin E in the eye, such that cataract formation may be decreased with vitamin E supplementation (65).
Dietary Sources of Vitamin E
Natural α-tocopherol is obtained largely from dietary sources such as whole grains, nuts, vegetable oils, and meats. The RRR-α-tocopherol content is especially high in wheat germ, safflower oil, and sunflower oil. Vitamin E in the diet is typically associated with polyunsaturated fatty acids (PUFAs), in particular linoleic acid. Vitamin E supplements, such as those widely consumed in the United States, are sold in the retinyl ester form and can contain natural RRR-α-tocopherol or the synthetic all-rac-α-tocopherol.
A common unit is used to express the total vitamin E activity of a food, diet, or biological sample. Traditionally, the IU for vitamin E was based on the biologic activity as determined by a rat fetal resorption assay. However, current convention is to report the vitamin E activity of a substance using units of milligrams of RRR-α-tocopherol, or RRR-α-tocopherol equivalents (αTE). For purposes of calculating vitamin E intakes in αTE, #979;-tocopherol is assumed to substitute for α-tocopherol with an efficiency of 10%, β-tocopherol with an efficiency of 50%, and α-tocotrienol with an efficiency of 30%. Note that 1 mg of α-tocopherol (αTE) is equal to 1.49 IU of vitamin E.
Experts generally agree that human milk supplies an amount of vitamin E adequate to meet the requirements of term infants. Values in human milk for α-tocopherol equivalents, composed mainly of α-tocopherol, range from 3.0 to 8.0 mg per L, and the vitamin E content of milk from mothers of preterm infants is similar to that from mothers of term infants. (Table 56.1) (40). Maternal tocopherol intake via supplements does not appear to have a major influence on the vitamin E content of human milk (66).
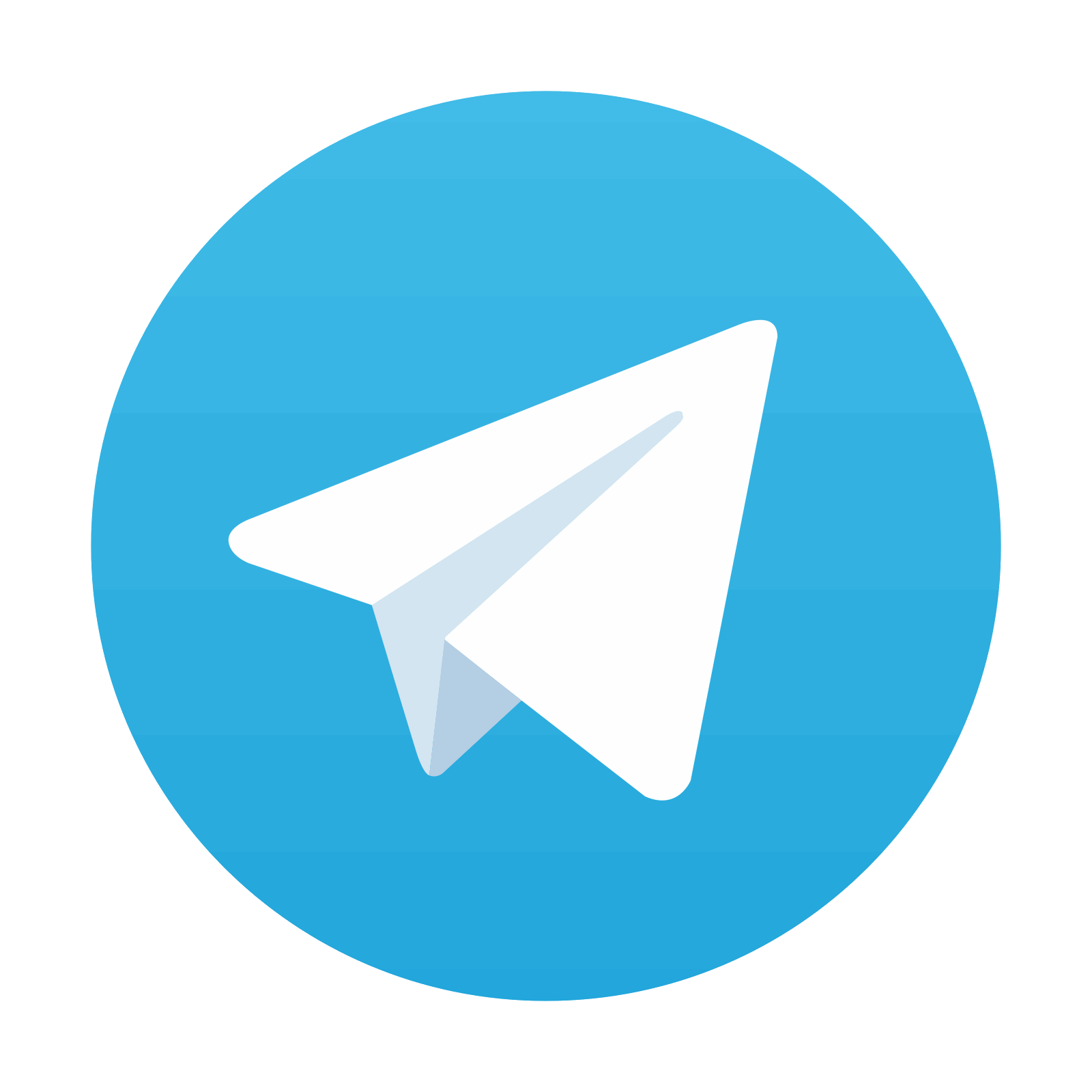
Stay updated, free articles. Join our Telegram channel

Full access? Get Clinical Tree
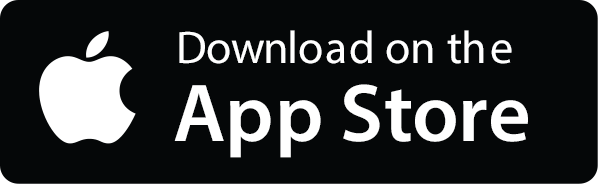
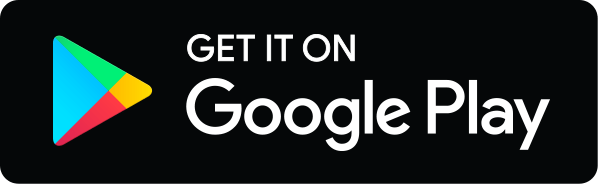