22 Andrés López Bernal1 and Errol R. Norwitz2 1 Translational Health Sciences, University of Bristol, Dorothy Hodgkin Building and St Michael’s Hospital, Bristol, UK 2 Department of Obstetrics and Gynecology, Tufts Medical Center, Boston, Massachussetts, USA The onset of labour is the result of a cascade of intrauterine biochemical events that culminates in cervical softening and dilatation and increased uterine contractility (Fig. 22.1). Alterations in the timing of birth are the leading cause of neonatal mortality and morbidity. The mechanisms involved in the onset of labour in women remain elusive and as a consequence the prediction and management of preterm labour are poor, resulting in potentially severe complications for the newborn, distress to the parents and high medical costs. Spontaneous preterm labour may or may not result from the same endocrine and intracellular pathways as the physiological onset of labour at term. Despite considerable improvements in special care baby units, the perinatal mortality rates for preterm birth remain steady, and there is a wide range of both short‐term and long‐term complications and handicap in the surviving infants [1,2]. On the other hand, our limited understanding of the physiological mechanisms can make induction of labour difficult. More than 25% of pregnant women will undergo an induction of labour for prolonged gestation (41 or more weeks), hypertensive disorders of pregnancy, pre‐labour rupture of membranes, or other indications [3]. A combination of vaginal prostaglandins and intravenous oxytocin is a common method for induction of labour; moreover, oxytocin is used for augmentation of contractions after labour of spontaneous onset. Stimulation of contractility carries the risk of failure and increased chance of delivery by caesarean section: in a study of reasons for caesarean section in labour in nulliparous women, the majority (70%) were due to ‘failed induction/dystocia’, 29% were indicated for fetal distress and 1% were due to acute clinical emergencies (e.g. cord prolapse, placental abruption) [4]. Studies involving more than two million women in several countries show that the rates of caesarean section in induction of labour at term are 30–40%, contributing to a 50% increase in caesarean section deliveries over the past decade [5]. This compelling epidemiological evidence shows that induction of labour at term is a major contributor to maternal and neonatal morbidity and emphasize the need to improve our understanding of human parturition so that we can develop more efficient ways of inducing and augmenting labour. Fig. 22.1 Proposed ‘parturition cascade’ for the onset of labour at term. The spontaneous onset of labour at term is regulated by a series of paracrine/autocrine hormones acting in an integrated parturition cascade. The factors responsible for maintaining uterine quiescence throughout gestation (a) and for the onset of labour at term (b) are shown. This includes the withdrawal of the inhibitory effects of progesterone on uterine contractility and the recruitment of cascades that promote estriol production and lead to upregulation of the contraction‐associated proteins within the uterus. ACTH, adrenocorticotrophic hormone (corticotrophin); CAPs, contraction‐associated proteins; CRH, corticotrophin‐releasing hormone; DHEAS, dehydroepiandrosterone sulfate; 11β‐HSD, 11β‐hydroxysteroid dehydrogenase; SROM, spontaneous rupture of membranes. Fetal surfactant (a mixture of lipids and apoproteins) is a signal for parturition because it provides a link between fetal lung maturation, which is essential for extrauterine life, and the onset of labour through stimulation of prostaglandin production. Amniotic fluid surfactant, which reflects fetal lung maturation, is an important intrauterine source of arachidonic acid, and increases the rate of prostaglandin synthesis in the fetal membranes. This effect is due to the release of fatty acids, including arachidonate, from the lipids of fetal surfactant by the sequential action of phospholipase C and diglyceride lipase activities in amniotic fluid and by the transfer of arachidonate from surfactant phosphatidylcholine to phosphatidylethanolamine and phosphatidylinositol in amnion cells [6]. These lipid pathways provide a link between the process of maturation of the fetus in preparation for birth and the labour‐initiating prostaglandin activation. In addition to the effect of its lipid components, fetal surfactant is likely to be involved in the process of parturition through the effects of the surfactant‐associated proteins SP‐A and SP‐D. These are multimeric molecules containing C‐type lectin domains and collagen‐like regions. SP‐A and SP‐D concentrations in amniotic fluid rise sharply from about 26 weeks’ gestation to term and the proteins are found in the fetal membranes and decidua [7]. SP‐A and SP‐D perform innate immune functions in the lungs via macrophage activation, control of inflammation, and clearance of microbes and apoptotic and necrotic cells. These proteins have an important role in non‐immune defence mechanisms in the amniotic cavity. SP‐A interacts with toll‐like receptors TLR‐2 and TLR‐4 on macrophages and increases the expression of interleukin (IL)‐1β and nuclear factor (NF)‐κB [8]. In mice, intra‐amniotic injection of SP‐A provokes premature delivery by a process which involves NF‐κB activation [9]. SP‐A has direct stimulatory effects on human myometrial cells through activation of NF‐κB and mitogen‐activated protein (MAP) kinase pathways [10]. On the other hand, SP‐A appears to inhibit proinflammatory cytokine expression in the amniotic epithelium [11]. The role of SP‐D in pulmonary host defence, particularly in the lungs of premature newborns, is well established. SP‐D binds DNA from bacteria and on the surface of dying cells, enhancing the clearance of nucleic acids and limiting inflammatory reactions at sites of infection or apoptosis. SP‐D is localized in the endometrium of the secretory phase and in placental villi and may have a role in the prevention of intrauterine infections at the time of implantation and during pregnancy [12]. Steroids have complex and versatile metabolic pathways, with complementary distribution of enzyme activities in the different compartments of the human fetoplacental unit [13]. The fetal adrenal glands and liver, the maternal adrenal and liver and the placenta operate as integrated steroidogenic organs in pregnancy [14]. The fetal adrenals produce large amounts of dehydroepiandrosterone (DHA) and DHA‐sulfate which, together with 16α‐hydroxy metabolites from the fetal liver, are essential precursors for the synthesis of oestrogens in the placenta [15]. Moreover, fetal pregnenolone sulfate contributes significantly to placental progesterone production, complementing progesterone synthesis from maternal cholesterol. The complexity of the steroid metabolome in fetal and maternal blood has been reviewed elsewhere [16]. There are many steroid metabolites in the circulation reflecting fetal organ development and maternal responses for parturition. For example, the levels of conjugated androstenediol and of 16α‐hydroxyandrostenedione rise sharply in fetal blood in the last few weeks of pregnancy [15]. On the other hand, several reduced progesterone metabolites including 5β‐dihydroprogesterone, which bind to progesterone receptors and may contribute to uterine quiescence, decrease in late pregnancy, providing a functional progesterone withdrawal [17]. The search for steroid signals for parturition is difficult, given the metabolic complexity and the multiplicity of cellular effects on uterine and cervical tissues; however, few areas of physiology offer such clear functional integration among fetal and maternal organs in late pregnancy. Placental corticotrophin‐releasing hormone (CRH) plays a key role in stimulating steroid synthesis in pregnancy, specifically the production of DHA and other steroid sulfates in the fetal zone of the fetal adrenal. Increasing CRH levels in the maternal circulation has been proposed as a predictor of parturition and preterm labour [18], but the performance is poor [19]. The predictive value of measuring multiple steroid compounds simultaneously during late pregnancy and around parturition is likely to be better than measuring CRH alone [16]. Decidual cells, especially tissue macrophages, may have a role in the mechanism of labour by promoting the synthesis and release of prostaglandins and proinflammatory cytokines [20]. Prostaglandins are the final common pathway for parturition in many species and synchronize uterine activation and cervical ripening through a highly integrated transport system and specific receptor signalling pathways in decidua and myometrium [21,22]. Moreover, the decidua provides a place of immune tolerance and defence to protect the fetus and the placenta from infections. The output of prostaglandin (PG)F2α by human decidual cells is higher in samples obtained after labour than before the onset of labour, whereas the output of other prostaglandins (PGE2, PGD2) and their metabolites remains relatively constant [23]. The release of the PGF isomer 9α,11β‐PGF2 from placenta, amnion and choriodecidua into the amniotic fluid increases at term [24]. PGF2α and 9α,11β‐PGF2 have direct effects on the myometrial cells of the uterus, increasing intracellular calcium and promoting contractions through the stimulatory FP receptor [25]. The reason for the selective increase in PGF release with labour is not clear, but it appears to involve the action of cytokines produced by macrophages that migrate and settle in the decidua where they have important regulatory roles in pregnancy. Macrophages exhibit remarkable plasticity and their behaviour is influenced by the tissue microenvironment. Proinflammatory cytokines (IL‐1; tumour necrosis factor‐α, TNF‐α) have strong paracrine and autocrine effects on decidual macrophages promoting the upregulation of prostaglandin synthase enzymes [24,26]. In some women, the premature release of prostaglandins provoked by decidual infection or haemorrhage can lead to very early delivery (before 30 weeks’ gestation) with devastating consequences for the baby [27,28]. The cellular disruption of inflammation, especially in the setting of infection, can lead to uterine activation and labour, but it should not be accepted as the general mechanism for the spontaneous onset of labour. In a study of more than 200 pregnant women undergoing caesarean section at term, the intrauterine site most commonly associated with histopathological inflammation was the decidua [29]. When caesarean sections were elective and carried out before the onset of labour, the incidence of deciduitis was low (6%) whereas in women operated on after the onset of labour deciduitis was much more frequent (29%). This could be interpreted as a cause–effect relationship between inflammation and spontaneous labour; however, most of the women with inflammation (86%) were in a group with cervical dilatation of 5 cm or greater. Decidual inflammation may be a consequence or labour, rather than its cause [29]. Inflammation of the fetal membranes is much more common in preterm deliveries than at term. A recent study detected acute chorioamnionic inflammation in 30% of preterm pregnancies but only 5.1 % of term pregnancies [30], confirming previous observations that there is an inverse relationship between gestational age and the frequency of bacteria in the fetal membranes or histological chorioamnionitis [31]. Many acute‐phase response genes increase in myometrium due to factors unrelated to labour (e.g. surgical trauma, hypoxia, underlying pathology); these changes are likely to be due to secondary inflammatory responses late in labour, rather than changes required for the onset of labour [32]. Inflammation may be a trigger for spontaneous labour in infection‐associated preterm deliveries, but it should not be accepted as the underlying mechanism for the onset of labour at term. The continuing search for the physiological pathways of parturition in women is important. New perspectives into decidual function and the timing of birth have been presented, highlighting the complex interaction of biological factors (prostaglandins, cytokines, growth factors and reactive oxygen species), genetic predisposition and immune defence mechanisms in the regulation of human parturition [33]. There are many genes potentially involved in the regulation of uterine contractility whose expression is modulated by oestrogens, progesterone and other pregnancy hormones. Oxytocin (OXT) is a member of an ancient nonapeptide hormone system and it is widely used in synthetic form for the induction and augmentation of labour because it stimulates uterine contractions. The pulse frequency of OXT secretion increases during human labour [34] and there is general agreement that it is important during the puerperium to facilitate uterine haemostasis after delivery and to initiate lactation. However, the role of OXT in the initiation of parturition is less clear. The high sensitivity of the uterus to OXT in pregnancy is due to a significant increase in the concentration of myometrial oxytocin receptor (OXTR) compared with the non‐pregnant uterus [35,36]. The OXT sensitivity of the uterus remains very high at term but there is no clear increase at parturition; labour begins with no preceding warning and no change in the sensitivity of the uterus to circulating levels of OXT [37]. OXT has several roles in human parturition: one promoting uterine contractility to facilitate delivery and to prevent postpartum haemorrhage, and other roles preparing the uterus for the initiation of labour. In addition to stimulating contractility through the phospholipase C/calcium pathway in myometrial cells, OXT activates transcription factors of the NFAT (nuclear factors of activated T cells) family in a calcineurin‐dependent manner [38]. The calcineurin–NFAT signalling cascade lies downstream of many cell surface receptors, including G protein‐coupled receptors (e.g. receptors for OXT and prostaglandins in myometrium) and receptor tyrosine kinases (e.g. receptors for growth factors and cytokines), providing points of convergence and amplification for hormones, growth factors and other endogenous mediators involved in the preparation of the uterus and the cervix for parturition. NFAT is involved in OXT‐mediated stimulation of PTGS2 (prostaglandin‐endoperoxide synthase 2, also known as COX2) expression in human myometrial cells, regulating the rate‐limiting step in prostaglandin synthesis. Moreover, OXT induces PTGS2 expression and stimulates prostaglandin release in human myometrial and amnion cells through other pathways, including the NF‐κB complex, providing a link with the uterine response to cytokines and other inflammatory stimuli [39]. The influence of OXT on calcium homeostasis in human myometrial cells highlights its importance in normal labour [40,41]. OXT is a potent stimulatory peptide and it has been proposed that an increase in the concentration of OXTR in the uterus may be a trigger for parturition [35]. The implication is that low levels of OXTR expression in myometrium may favour uterine quiescence during ongoing pregnancy. However, the concentration of myometrial OXTR is very high in late pregnancy and there is no evidence that it increases further at the onset of labour [42–44]. It may be argued that uncoupling or blocking of OXTR may be a mechanism to inhibit contractility until parturition. However, the sensitivity of the human uterus to OXT remains relatively unchanged during the last few weeks of pregnancy and does not increase before the spontaneous onset of labour [37]. Both OXT‐ and OXTR‐deficient mice have normal pregnancies and deliveries [45,46]. The accumulated evidence in mice suggests that inhibition of OXTR function is not responsible for uterine relaxation in pregnancy, although OXT retains important roles in luteal function [47], nursing response [46] and social behaviour [45,48]. Nevertheless, the design of OXTR antagonists is seen as a good approach for the management of preterm labour in women, because these drugs have relatively high uterine selectivity and few side effects [41,49,50]. The concentration of epidermal growth factor (EGF) increases in amniotic fluid during pregnancy [51]. EGF concentrations in maternal blood range from 70 to 800 pg/mL [52], whereas in cord blood the levels are higher (500–3000 pg/mL) [53]. EGF has traditionally been studied as a critical factor in fetal maturation and more recently it has been implicated in the pathogenesis of pre‐eclampsia [52]. However, the role of EGF as a physiological mediator in parturition is less well established. EGF stimulates PTGS2 expression in human amnion [54] and myometrial cells [55] and the mechanism has been investigated in some detail. EGF operates through a specific receptor (EGFR, the first member of the ErbB tyrosine kinase family), which is the target of several clinically approved anticancer agents. In human myometrial cells, EGF stimulates PTGS2 expression through interaction of EGFR with members of the mitogen‐activated protein kinase family, namely MEK1/2 and ERK1/2. This effect requires activation of protein kinase C [55]. Interestingly, OXT stimulates ERK1/2 in myometrial cells through a mechanisms that may involve transactivation of EGFR by OXTR and the effect of calcium [56]. The effect of EGF, and possibly other growth factors and cytokines, on prostaglandin release and acute‐phase responses in the uterus is mediated by steroid receptor co‐activator interacting proteins and by transcription factors [57]. OXT and EGF can stimulate PTGS2 expression and prostaglandin release through receptor transactivation and convergence of intracellular signals. These cooperative effects facilitate the preparation for labour in a physiological manner. Moreover, these pathways can be further stimulated by proinflammatory cytokines which amplify PTGS2 transcriptional responses via NF‐κB and protein kinase C [39,55]. IL‐1β and TNF‐α stimulate not only PTGS2, but also the terminal prostaglandin synthases AKR1B1 (prostaglandin F synthase) and PTGES (prostaglandin E synthase) in human myometrial cells [58]. It is likely that the mechanism of labour is set to respond primarily to endogenous hormones and growth factors, with the system retaining the ability to react to inflammatory stimuli in an accelerated or premature manner (Fig. 22.2). Many studies rely on experiments using isolated uterine tissues in vitro and may not account for the full biological complexity of interacting maternal and fetal organs during parturition in vivo. Fig. 22.2 Simplified mechanisms of uterine activation. (a) During pregnancy the uterus remains relatively quiescent with little prostaglandin synthesis because the inducible prostaglandin‐endoperoxide synthase 2, also known as COX2, is expressed at low levels. (b) COX2 expression and prostaglandin output can be increased through stimulation of oxytocin receptors (OXTR) and epidermal growth factor receptors (EGFR) which act separately or coordinately via major signalling cascades involving mitogen‐activated protein kinases (e.g. ERK), protein kinase C (PKC) and transcription factors such as NFAT (nuclear factor of activated T cells). (c) In the presence of inflammatory stimuli (leucocyte infiltration/macrophage activation following infection or haemorrhage) interleukin 1β receptors (IL1R) can increase prostaglandin release to very high levels through strong stimulation of COX2 and other terminal prostaglandin synthases (e.g. PTGES). The pathways involved include PKC and NFKB (nuclear factor of kappa B) and may involve disruption of OXTR and EGFR signalling. The uterus provides a safe environment for the blastocyst to implant. The nutritious endometrial layer becomes receptive and encourages the development of a viable embryo and the trophoblast. Over a period of 40 weeks of gestation, the fetus, placenta and amniotic fluid increase their masses considerably, posing remarkable haemodynamic, metabolic and mechanical demands on the mother. At the same time, the uterus must expand and remain relatively relaxed, with a closed cervix, to allow the growth and differentiation of the fetus to a stage when it is ready to cope with extrauterine life. However, at the onset of labour the uterus is responsible for driving the process of birth through structural, biochemical and electrophysiological changes that result in cervical ripening and dilatation and the establishment of synchronized myometrial contractions. The process ends with the delivery of the newborn and the placenta, followed by an intense period of uterine remodelling and involution. The myometrial layer contains specialized smooth muscle cells arranged in bundles embedded in a matrix of collagenous connective tissue. The collagen fibres facilitate the transmission of force generated by myometrial bundles. The process of cervical ripening involves changes in the connective tissue with an increase in collagen solubility and alterations in proteoglycans of the ground substance. This process has been regarded as an inflammatory reaction [59], with elevated levels of cytokines [60] and other proinflammatory molecules signalling through toll‐like receptors (TLR2, TLR4) implicated in the mechanism of cervical remodelling in spontaneous labour [61]. Myometrial smooth muscle cells contain actin (thin filaments) and myosin (thick filaments) in a less organized fashion than in striated muscle, but nevertheless forming efficient contractile units due to their intricate cytoskeletal organization [62]. Mammalian smooth muscle myosin belong to the class II myosins, which are characterized by a hexameric protein structure composed of two heavy chains (about 200 kDa) and two pairs of light chains: the 20‐kDa regulatory myosin light chain (MYL20) and the 17‐kDa essential light chain. Functionally, myosin consists of three domains: the motor domain which interacts with actin and binds ATP, the neck domain which binds the light chains and is the site of calmodulin interaction, and the tail domain or anchoring domain which helps position the motor domain so that it interacts with actin [63]. Myosin generates contractile force by pulling actin filaments of opposite polarity towards one another (Fig. 22.3). Fig. 22.3 Mechanics of muscle contraction. (a) Appearance of the contractile unit in the resting state. The thick filament refers to myosin; the thin filament is actin. Myosin‐binding sites on the actin filaments are covered by a thin filament known as tropomyosin that obscures the myosin‐biding sites, therefore preventing the myosin heads from attaching to actin and forming cross‐bridges. Adenosine triphosphate (ATP) is hydrolysed into adenosine diphosphate (ADP) and inorganic phosphate (Pi). The troponin complex is attached to the tropomyosin filament. (b) As intracellular calcium concentrations increase, calcium binds to the troponin complex resulting in a conformational change that allows binding sites between actin and myosin to be exposed with the formation of actin–myosin cross‐bridges. (c) Formation of actin–myosin cross‐bridges results in release of Pi and ADP, causing the myosin heads to bend and slide past the myosin fibres. This ‘power stroke’ results in shortening of the contractile unit and generation of force within the muscle. (d) At the end of the power stroke, the myosin head releases the actin‐binding site, is cocked back to its furthest position, and binds to a new molecule of ATP in preparation for another contraction. The binding of myosin heads occurs asynchronously (i.e. some myosin heads are binding while other heads are releasing the actin filaments), which allows the muscle to generate a continuous smooth force. Cross‐bridges must therefore form repeatedly during a single muscle contraction. Myosin is both a structural protein and an enzyme (Mg‐ATPase) capable of hydrolysing ATP to generate mechanical energy. The ATPase region of myosin is located in the head and is activated by actin; however, the level of activity is very low when MYL20 is unphosphorylated, but increases manifold upon phosphorylation of the regulatory chains. The enzyme responsible for MYL20 phosphorylation is the calcium‐calmodulin dependent myosin light chain kinase (MYLK). There are three types of MYLK, the skeletal, cardiac and smooth muscle enzymes. The three types have a common conserved serine threonine kinase domain, but smooth muscle MYLK has unique immunoglobulin and fibronectin domains [64]. Despite its name, smooth muscle MYLK is expressed in almost all tissues and is involved not only in contractions but in many other cellular activities. Kinases are often portrayed as promiscuous enzymes with multiple substrates; however, despite its ubiquitous expression, the only known substrate of MYLK is myosin. Human myometrium expresses 137‐kDa and 218‐kDa isoforms of MYLK in both non‐pregnant and in term pregnant uterine tissue [65,66]. Interestingly, a small 19‐kDa non‐catalytic C‐terminal fragment of MYLK called telokin is highly expressed in pregnant compared to non‐pregnant myometrium, suggesting it may have a regulatory role in gestation [66]. Smooth muscle MYLK has an actin‐binding domain at the N‐terminus which binds to purified polymeric actin (F‐actin); however, the affinity of MYLK for myofilaments, which contain myosin II and many other proteins in addition to F‐actin, is much higher. MYLK also has myosin‐ and calmodulin‐binding domains. Purified MYLK can only bind to calmodulin in the presence of calcium. The protein structure of MYLK suggests that it is a long and flexible molecule capable of attaching itself to actin through its N‐terminus, and to the neck region of myosin through the C‐terminal telokin domain. In this position the catalytic core of the enzyme is able to phosphorylate MYL20 in the thick filaments while being tightly anchored to the thin filaments. MYLK can itself be phosphorylated, modulating its activity during myometrial contractions. Myometrial smooth muscle is myogenic: it can generate contractions spontaneously without the need for external stimulation. Contraction of myometrial cells is initiated by action potentials that depolarize the cell membrane, allowing the rapid entry of calcium through voltage‐operated channels (Fig. 22.4). The rise in intracellular calcium concentration ([Ca2+]i) is detected by the Ca2+ sensor calmodulin, which activates MYLK and provokes the phosphorylation of MYL20. The activity of myometrial MYLK is very sensitive to minor changes in [Ca2+]i around the 100–200 nM range and it is thought that the enzyme reaches maximal activity at lower Ca2+ concentrations than in other types of smooth muscle. Phosphorylation of MYL20 stimulates the formation of cross‐bridges between actin and myosin filaments and the generation of force [65,67]. The phasic nature of myometrial contractions (recurrent episodes of force separated by intervals of relaxation) at parturition is necessary to allow vascular flow in the placenta and exchange of oxygen and waste products with the fetus during several hours of labour. In order to promote relaxation between contractions, myometrial cells have efficient Ca2+ extrusion mechanisms that include Ca2+ pumps and Na+/Ca2+ exchangers in the cell membrane, as well as Ca2+ entry into intracellular stores, for example sarcoplasmic reticulum (SR) [68,69] (Fig. 22.4). Fig. 22.4 The physiological basis of myometrial contractility. Action potentials are generated by pacemaker depolarization of the cell membrane (top) which is regulated by a complex interaction of several channels and ion pumps. These include store‐operated (S) and T‐type calcium channels as well as calcium‐sensitive chloride (Cl–) channels, all of which contribute to membrane depolarization. This is balanced by the Na+/Ca2+ exchanger, and the strong hyperpolarizing effect of the Na+/K+ pump and the calcium‐sensitive K+ channels. Action potentials (measured in mV) provoke rapid entry of Ca2+ into the cell by opening voltage‐operated L‐type channels (VOC); the increase in intracellular Ca2+ levels (typically from 100 to 500 nM) provokes tension (measured in mN) by enhancing actin–myosin interaction. Stimulatory agonists such as oxytocin operate through G protein‐coupled receptors (GPCR) which activate G protein effector complexes (G/E). A common G/E interaction involves Gq/phospholipase C which generates two second messengers from membrane phosphatidylinositol 4,5‐bisphosphate (PIP2), namely inositol 1,4,5‐trisphosphate (InsP3) and diacylglycerol (DAG). InsP3 stimulates contractility by releasing Ca2+ from intracellular stores in the sarcoplasmic reticulum (SR) through ITPR channels. DAG stimulates protein kinase C. The phasic nature of myometrial contraction requires rapid Ca2+ extrusion mechanisms to lower intracellular Ca2+ and decrease tension. Ca2+ is transported out of the cell by plasma membrane Ca2+‐ATPases (PMCA) and taken up by the SR through smooth endoplasmic reticulum Ca2+‐ATPases (SERCA). Phospholamban is a protein that inhibits SERCA, but this inhibition can be blocked by phosphorylation of phospholamban by cyclic AMP‐dependent kinases. Sources: adapted from Noble et al. [83], Berridge [84] and Sanborn [89]. The increase in steroid hormones and placental‐derived growth factors in pregnancy has important effects on the structure of the uterus. Myometrial tissue becomes more vascularized and there is hyperplasia and hypertrophy of myometrial cells. The cellular content of actin and myosin and of the actin‐binding proteins caldesmon and calponin increases several fold compared with non‐pregnant cells [65]. The increased expression of caldesmon protein in pregnancy has been described as a ‘brake’ on the myosin motors of the contractile filaments by a mechanism of Ca2+ desensitization [70]. Comparisons between pregnant and non‐pregnant myometrial strips in vitro show that the relationship between the level of MYL phosphorylation and the amount of force is more favourable in pregnant tissue [65]. The phasic nature of uterine contractions is an intrinsic property of uterine smooth muscle and is related to the capacity of myometrial cells to modify [Ca2+]i through reversible activation/inhibition of membrane receptors, ion channels and Ca2+ pumps. The main source of Ca2+ is the extracellular fluid, but intracellular stores such as the SR have important regulatory functions [71]. Myometrial cells are depolarized by action potentials which provoke the influx of Ca2+ through voltage‐operated channels [72,73]. Interestingly, spontaneous action potentials in pregnant human myometrial cells are inhibited in sodium‐deficient or calcium‐free solutions [74–76]. This is due to the essential role of extracellular Ca2+ and of membrane Na+/Ca2+ exchangers in the generation of action potentials and myogenic contractility. Magnesium sulfate is a natural calcium antagonist and a potent L‐type calcium channel inhibitor. Nifedipine is a commonly used pharmacological blocker of external Ca2+ entry which also acts on L‐type channels. In the presence of nifedipine both spontaneous and OXT‐induced contractions in myometrial strips from term pregnant women are virtually abolished, confirming the essential role of external Ca2+ in the development of phasic uterine contractions in late pregnancy. L‐type calcium channels have been targeted pharmacologically in the management of preterm labour, using magnesium sulfate or nifedipine; these drugs are effective at relaxing the uterus but they lack myometrial selectivity and can cause side effects. The role of T‐type channels in the transmission of action potentials and in the regulation of contraction frequency in myometrial cells has been investigated, but the ubiquitous expression of these channels makes them a poor pharmacological target for the control of uterine activity [77,78]. The study of calcium stores in smooth muscle has benefited from the development of fluorescent calcium indicator dyes that allow the measurement of transient rises in free Ca2+ in discrete cellular compartments. A number of general observations have been made across many tissues [79] which are relevant to human myometrium. Several isoforms of RYR and ITPR have been described in human myometrium [42,80]. The RYR acts as a Ca2+ channel and studies using human myometrial cells have confirmed the presence of functional RYRs in this tissue [81]. Ryanodine and cyclic ADP‐ribose (cADPR) promote intracellular Ca2+ release in myometrial preparations in vitro and potentiate oxytocin‐induced [Ca2+]i increases by facilitating Ca2+ entry from the extracellular medium through ‘calcium‐induced calcium release’ [82]. Interestingly, inflammatory mediators such as TNF‐α induce CD38 expression in myometrial cells; this suggests that inflammation may potentiate the parturition cascade by promoting the cADPR system [82]. ITPRs also act as intracellular Ca2+ channels and are activated by inositol 1,4,5‐trisphosphate (InsP3) generated when endogenous myometrial stimulants such as OXT, endothelin or α‐adrenergic agonists bind GPCRs coupled to proteins of the Gq family which activate phospholipase C. InsP3 binds to myometrial ITPRs and promotes Ca2+ release [42,71]. Experiments using myometrial strips from late pregnant women [71] and imaging techniques that allow the visualization of discrete Ca2+ fluxes in lipid microdomains [69] suggest that ITPR channel activation is an early event in uterine activation by OXT; however, in the absence of extracellular Ca2+ the development of force is short‐lived because intracellular Ca2+ stores are soon exhausted; in other words, the development of phasic uterine contractions is absolutely dependent on the availability of extracellular Ca2+ to myometrial cells. Under normal physiological circumstances, for example in the absence of inflammation, ITPR channels in the SR appear to be more important for myometrial function than RYR channels [71]. Experiments using myometrial strips from late pregnant women showed that ryanodine had little effect on spontaneous or OXT‐induced contractions [71], suggesting no functional role for RYR in this preparation. Moreover, blocking SR Ca2+ uptake with cyclopiazonic acid, an inhibitor of Ca‐ATPase in myometrium, increased contractile force and [Ca2+]i.This suggests that the SR may normally contribute to lowering [Ca2+]i during the relaxation phase of the contractions [71]. The complex role of intracellular Ca2+ stores requires further investigation because, in addition to acting as a trigger for agonist‐induced Ca2+ mobilization, the SR may act as a ‘sink’ for Ca2+ during stimulation and, by enhancing plasma membrane Ca2+ efflux, may contribute to homeostatic Ca2+ relaxation mechanisms [83]. A model for the activation of human myometrium based on electrophysiological and receptor mechanisms has been proposed [84], partly based on available experimental evidence [69,85,86]. In this model, activation of uterine contractility during labour is driven by action potentials initiated by slow depolarization of clusters of pacemaker myometrial cells. The action potentials trigger Ca2+ entry into the cells through L‐type voltage‐operated channels and the rise in [Ca2+]i provokes contractions, thus linking the electrical signal with force (excitation–contraction coupling). This model envisages that the balance of depolarization and hyperpolarization in the cell membrane is controlled by multiple pumps and channels, including a Na+/Ca2+ exchanger; a Na+/K+ pump, and Ca2+‐activated K+ channels that contribute to relaxation (hyperpolarization). On the other hand, Ca2+‐sensitive chloride channels, T‐type channels, and a putative channel that senses whether the level of Ca2+ in the SR store is low (store‐operated channel, SOC) can tip the balance of the ‘pacemaker’ towards depolarization [84]. GPCR agonists like OXT can initiate depolarization by generating InsP3 and emptying the SR store through ITPR channels, thus activating SOC, whereas RYR channels would contribute to contraction directly by increasing [Ca2+]i. Further regulatory refinement of myometrial activation can be provided by Ca2+‐ATPases that promote Ca2+ extrusion through the plasma membrane (PMCA) or Ca2+ uptake into the SR (SERCA). Some aspects of this model have been reinforced by the abundant experimental evidence in favour of a role for L‐type Ca2+ channels as the major route for Ca2+ entry into myometrial cells and the unquestionable involvement of the GPCR/phospholipase C/InsP3 pathway activating ITPR channels in human myometrium [76,87]. However the role of RYR channels in human myometrium is questionable. The presence of Ca2+‐activated K+ channels in this tissue has been demonstrated but their role in phasic myometrial activity during labour is not clear. An interesting interaction between Ca2+‐activated K+ channels and α2‐macroglobulin has been described in myometrial cells whereby in the presence of OXT α2‐macroglobulin induces oscillations in Ca2+‐activated K+ channel activity and promotes Ca2+ entry via SOC [88]. A number of endogenous Ca2+‐ATPase modulators has been described, including phospholamban, a membrane‐associated protein that inhibits SERCA‐mediated Ca2+ uptake into the SR. Interestingly, phospholamban can be phosphorylated by cAMP‐dependent kinases leading to its inactivation and the liberation of SERCA activity [83]. In addition to the spontaneous uterine activity driven by action potentials, there is strong evidence that receptors and their cell signalling pathways have a physiological influence on the regulation of uterine contractility. The uterus has a rich variety of receptors, many of which are upregulated in pregnancy, and responds to classical hormones and transmitters (e.g. OXT, 5‐hydroxytryptamine) as well as local modulators (e.g. prostaglandins and thromboxanes). The link between the myometrial cell membrane receptor and the initiation of a signalling cascade is, in most cases, a regulatory GTP‐binding protein. G proteins can activate effector enzymes such as phospholipase Cβ (PLCB), adenylyl cyclase (ADCY) or phosphoinositide 3‐kinase. The PLCB pathway is activated by receptors usually coupled to Gq which stimulate myometrial contractility. Endogenous agonists include peptide hormones (OXT, endothelin), prostanoids (PGF2α, thromboxane A2), catecholamines, muscarinic agents and inflammatory mediators (bradykinin, serotonin). The response is initiated by the hydrolysis of a receptor‐sensitive pool of phosphatidylinositol 4,5‐bisphosphate (PIP2) in the cell membrane. The breakdown of PIP2 generates two molecules with potent signalling effects: InsP3, which releases calcium form the SR, and 1,2‐diacylglycerol (DAG), which activates protein kinase C (PRKC) and stimulates the phosphorylation of many target proteins. Potential substrates of PRKC include ion channels, calcium pumps, and proteins involved in GPCR function and coupling to phospholipase C, ITPR regulation and elements of the contractile machinery [89]. In addition, DAG has PRKC‐independent effects, for example in human myometrial cells DAG analogues facilitate store‐operated or receptor‐operated Ca2+ entry through the cell membrane via L‐type channels and Na+/Ca2+ exchangers [90]. Some ligands can activate more than one type of myometrial receptor, creating complex responses depending on the relative abundance and affinity of each receptor subtype and the presence of other agonists competing or interacting with related receptors. For each receptor, the effect on myometrial contractility will depend on the integration of these signals and on the physiological state of the organ under different endocrine influences. Changes in myometrial sensitivity to agonists are likely to be important mechanisms for maintaining the balance between uterine quiescence and contractility. The uterus is very sensitive to OXT, which is commonly used for the induction and augmentation of labour; however, many uncertainties remain about its mechanism of action. For instance, analysis of the Ca2+–tension relationship in pregnant myometrial strips reveals a strong component of ‘Ca2+ sensitization’ during OXT‐induced contractions [91,92], probably involving inhibition of myosin phosphatase. The pathways involve small GTP‐binding proteins of the Rho family and activation of Rho‐dependent kinases [93]. The physiological mechanisms responsible for the transition of the pregnant uterus from a long period of relative relaxation to a short period of active contractions in labour remain unknown. Greater understanding of the factors involved in the loss of uterine quiescence may assist in the anticipation and prevention of preterm labour. Therapeutic approaches to inhibit uterine contractility in women in preterm labour remain rudimentary [94], based on the use of drugs that are not effective or have potentially serious side effects. We need to increase our understanding of the endocrine and physiological control of parturition in women. The importance of cyclic nucleotides (cGMP and cAMP) in smooth muscle function cannot be over‐emphasized. These molecules provide rapid intracellular responses to a variety of signals and have profound effects on regulatory proteins through the activation of specific protein kinases. The role of cyclic GMP as a mediator of nitric oxide (NO) [95] effects in myometrium is a matter of controversy. Initial studies suggested a link between NO production, cGMP generation and uterine relaxation [96]. However, cGMP has a much more potent inhibitory role in tracheal and vascular smooth muscle than in myometrium [97,98]. Intravenous nitroglycerin has been used to relax the uterus during postpartum emergencies [99,100] but the use of NO donors for routine tocolysis is not recommended [101]. Cyclic AMP is produced from ATP in response to activation of GPCRs positively coupled to ADCY, and is known to influence a wide range of physiological events including relaxation of myometrial smooth muscle [102]. The actions of cAMP in myometrial cells, as in other cell types, result from the dynamic interplay of multiple ADCY, phosphodiesterases and protein kinase A (PRKA) isoforms that allow spatial and temporal changes in cAMP levels to be translated into compartmentalized responses within the cell. Most of the effects of cAMP are due to its ability to bind to PRKA. The localization of PRKA activity within cells depends on reversible interactions with ‘A kinase anchor proteins’ (AKAP). Although the evidence that agonist‐mediated increases in cAMP result in uterine relaxation is strong, the mechanism of action of cAMP in myometrium is not well known. PRKA–AKAP complexes have been described in pregnant human myometrium [103–105], but there is little information about the physiological targets that become phosphorylated during cAMP‐induced relaxation. New lines of research suggest that cAMP interacts with progesterone receptors to regulate COX2 expression in human myometrial cells [106]. The uterus has GPCRs for many endogenous agonists and responds with contraction or relaxation depending on the type of G protein and second messenger pathway activated. In general, receptors coupled to Gq are stimulatory through activation of PLCB and receptors coupled to Gs are inhibitory (promote relaxation) via stimulation of ADCY (Table 22.1). Some ligands can activate more than one type of myometrial receptor, creating complex responses depending on the relative abundance and affinity of each receptor subtype and the presence of other agonists competing or interacting with related receptors. G proteins are heterotrimers (αβγ subunits) and the effects of Gα subunits on PLCB, ADCY and other effectors are modulated by the interaction of Gβγ subunits, especially those liberated from Gi/o or G12, with the same or with other effector proteins including ADCY, ARF6, GRK, PLCB, PLCD, phosphatidylinositol kinases and ion channels. For each receptor, the effect on myometrial contractility will depend on the integration of these signals and on the physiological state of the organ under different endocrine influences. Changes in myometrial sensitivity to agonists may be an important mechanism for maintaining the balance between uterine quiescence and contractility. For example, pregnancy induces an increase in the myometrial response to OXT [35] and histamine [95] and a decrease in the response to tachykinins in women [95]. More research needs to be done on the responses of pregnant and non‐pregnant human myometrium to selective GPCR agonists and antagonists to build a complete picture. Table 22.1 Examples of G protein‐coupled receptors in myometrium and their signalling pathways.* * This table is not exhaustive and other receptors require further study in human pregnant myometrium [127–129]. ADCY, adenylyl cyclase; ARF6, ADP‐ribosylation factor 6; ARHGEF, RHO guanine nucleotide exchange factor; DAG, 1,2‐diacylglycerol; GRK, G protein‐coupled receptor kinase; InsP3, inositol 1,4,5‐trisphosphate; PLCB, phospholipase Cβ; PLCD, phospholipase Cδ; PRKA, protein kinase A; PRKCB, protein kinase Cβ. The β2‐adrenoceptor (ADRB2) has been a pharmacological target to relax the uterus for many years, using beta‐mimetic drugs. Exposure of human pregnant myometrial strips to the β2 agonist isoproterenol in vitro results in a significant decrease in contractile force and in the frequency of spontaneous contractions. Similar effects have been obtained with ritodrine, a beta‐mimetic used as a tocolytic agent in preterm labour [107,108]. Table 22.2 lists a number of endogenous ligands that can relax the uterus. Some of these, for example catecholamines and prostaglandins, have complex stimulatory and inhibitory effects due to the presence of multiple receptors in myometrium. Inhibitory receptors such as ADRB2 or the prostaglandin PTGER2 receptors have in common the coupling through Gαs to ADCY. Receptors coupled to Gi/o (e.g. α2‐adrenoceptors, ADRA2) inhibit ADCY and decrease cAMP production, favouring uterine contractions; however, in many systems prolonged stimulation of Gi/o‐coupled receptors provokes sensitization of ADCY to subsequent stimulation by other Gs‐coupled receptors (heterologous sensitization) [109]. Table 22.2 Endogenous and exogenous factors affecting myometrial contractility during labour. Changes in the sensitivity to endogenous agonists (catecholamines, prostaglandins) operating through ADRB2, PTGER2 and other receptors coupled to cAMP production may be responsible for the transition from uterine quiescence to the onset of labour. This is an attractive hypothesis, although it requires more evidence to support it. Beta‐mimetics (ritodrine, terbutaline, salbutamol) were among the earliest agents used clinically to inhibit uterine contractions, but their efficacy is questionable [110]. ADRB2 receptors are widely expressed in many organs, and consequently the use of beta‐mimetics in pregnancy is associated with potentially serious cardiovascular, neuromuscular and metabolic side effects. There is contrasting new information to be considered before the possible clinical use of PTGER2 agonists to relax the uterus [111]. Sustained stimulation of GPCRs typically causes desensitization (a reduction in response in the face of a constant stimulus), which protects cells from over‐stimulation and involves numerous adaptive mechanisms in characteristic time frames. Within seconds to minutes of stimulation, most GPCRs desensitize as a consequence of receptor phosphorylation by G‐protein receptor kinases (GRKs) or second‐messenger regulated protein kinases which are very abundant in human myometrium [112]. This phosphorylation can inhibit G‐protein activation (i.e. cause receptor desensitization), but the most important effect of GRK‐mediated phosphorylation is to facilitate the binding of arrestin proteins [113]. This not only prevents G‐protein activation but also targets the desensitized receptor for internalization. The consequent reduction in cell surface receptor number can also underlie desensitization in the intermediate time frame of minutes to hours. Over longer periods (hours to days), numerous adaptive processes serve to either reinforce or reverse the earlier desensitization by changing rates of synthesis and degradation of receptors and downstream effectors. Arrestins have many other roles, including acting as scaffolds to facilitate receptor‐stimulated activation of mitogen‐activated protein kinases (MAPKs) in discrete cellular compartments. This mechanism has been confirmed for bradykinin B2 receptors in human myometrial cells [114]. One of the difficulties with the use of agonists for Gαs‐coupled receptors to promote uterine relaxation is the tendency of GPCRs to desensitize and downregulate. This process has been particularly well studied for the ADRB2 receptor but it has long been known to occur with other utero‐relaxing receptors such as PTGER2 and PTGIR [115]. The loss of tocolytic effect of ritodrine in women in preterm labour has been known for many years [116].
Normal Mechanisms in Labour
Parturition
Fetal signals
Steroids
Inflammatory responses
Decidual activation
Inflammation and the onset of labour
Endogenous mediators
Role of oxytocin
Oxytocin receptors
Epidermal growth factor
The uterus as a smooth muscle organ
The basis of uterine contractility
Generation of uterine contractions
Calcium homeostasis
Intracellular calcium stores
Electrophysiological mechanisms
Receptor‐regulated myometrial contractility
Uterine relaxation
Role of cyclic nucleotides
G protein‐coupled receptors and their second messenger pathways
Endogenous ligands
Receptors
G protein class
Signalling pathway
Effect on myometrial contractility
Amines
Catecholamines
ADRA1
Gq/11
↑PLCB/InsP3–Ca2+/DAG–PRKCB
↑PLCD/ InsP3–Ca2+
Stimulation
ADRA2
Gi/o
↓ADCY/cAMP/PRKA
Stimulation
ADRB2
Gs
↑ADCY/cAMP/PRKA
Inhibition
ADRB3
Gs
Gi/o
↑ADCY/cAMP/PRKA
↓ADCY/cAMP/PRKA
Inhibition
Histamine
HRH1
Gq/11
↑PLCB/InsP3–Ca2+/DAG–PRKCB
Stimulation
HRH2
Gs
↑ADCY/cAMP/PRKA
Inhibition
Serotonin
HTR1,2
Gi/o
Gq/11
↓ADCY/cAMP/PRKA
↑PLCB/InsP3–Ca2+/DAG–PRKCB
Stimulation
HTR4,7
Gs
↑ADCY/cAMP/PRKA
Inhibition
Eicosanoids
Prostaglandin D2
PTGDR
Gs
↑ADCY/cAMP/PRKA
Inhibition
Prostaglandin E2
PTGER1
Gq/11
↑PLCB/InsP3–Ca2+/DAG–PRKCB
Stimulation
PTGER2
Gs
↑ADCY/cAMP/PRKA
Inhibition
PTGER3
Gi/o
Gs
Gq/11
↓ADCY/cAMP/PRKA
↑ADCY/cAMP/PRKA
↑PLCB/InsP3–Ca2+/DAG–PRKCB
Stimulation/inhibition
PTGER4
Gs
↑ADCY/cAMP/PRKA
Inhibition
Prostaglandin F2α
PTGFR
Gq/11
↑PLCB/InsP3–Ca2+/DAG–PRKCB
Stimulation
Prostacyclin
PTGIR
Gs
↑ADCY/cAMP/PRKA
Inhibition
Thromboxane A2
TBXA1R
Gq/11
G12/13
↑PLCB/InsP3–Ca2+/DAG–PRKCB ↑ARHGEF/RHOA/ARF6/PLD
Stimulation
Peptides
Angiotensin
AGTR2
G12/13
↑ARHGEF/RHOA/ARF6/PLD
Stimulation
Bradykinin
BDKRB2
↑PLCB/InsP3–Ca2+/DAG–PRKCB
Stimulation
Calcitonin‐related
CL‐RAMP
Gs
↑ADCY/cAMP/PRKA
Inhibition
Endothelin
EDNRA
Gq/11
Gi
↑PLCB/InsP3–Ca2+/DAG–PRKCB
↓ADCY/cAMP/PRKA
Stimulation
Oxytocin/vasopressin
OXTR/AVPR1A
Gq/11
Gi
↑PLCB/InsP3–Ca2+/DAG–PRKCB
↓ADCY/cAMP/PRKA
Stimulation
Receptor‐mediated uterine relaxation
Uterine stimulants
Endogenous
Oxytocin
Prostaglandins
Endothelin
Epidermal growth factor
Exogenous
Oxytocin
Prostaglandins
Uterine relaxants
Endogenous
Relaxin
Nitric oxide
L‐Arginine
Magnesium
Corticotrophin‐releasing hormone
Exogenous
β‐Adrenergic agonists (ritodrine hydrochloride, terbutaline sulfate, salbutamol, fenoterol)
Oxytocin receptor antagonist (atosiban)
Magnesium sulfate
Calcium channel blockers (nifedipine, diltiazem, verapamil)
Prostaglandin inhibitors (indometacin)
Phosphodiesterase inhibitor (aminophylline)
Nitric oxide donor (nitroglycerin, sodium nitroprusside)
Desensitization of GPCRs
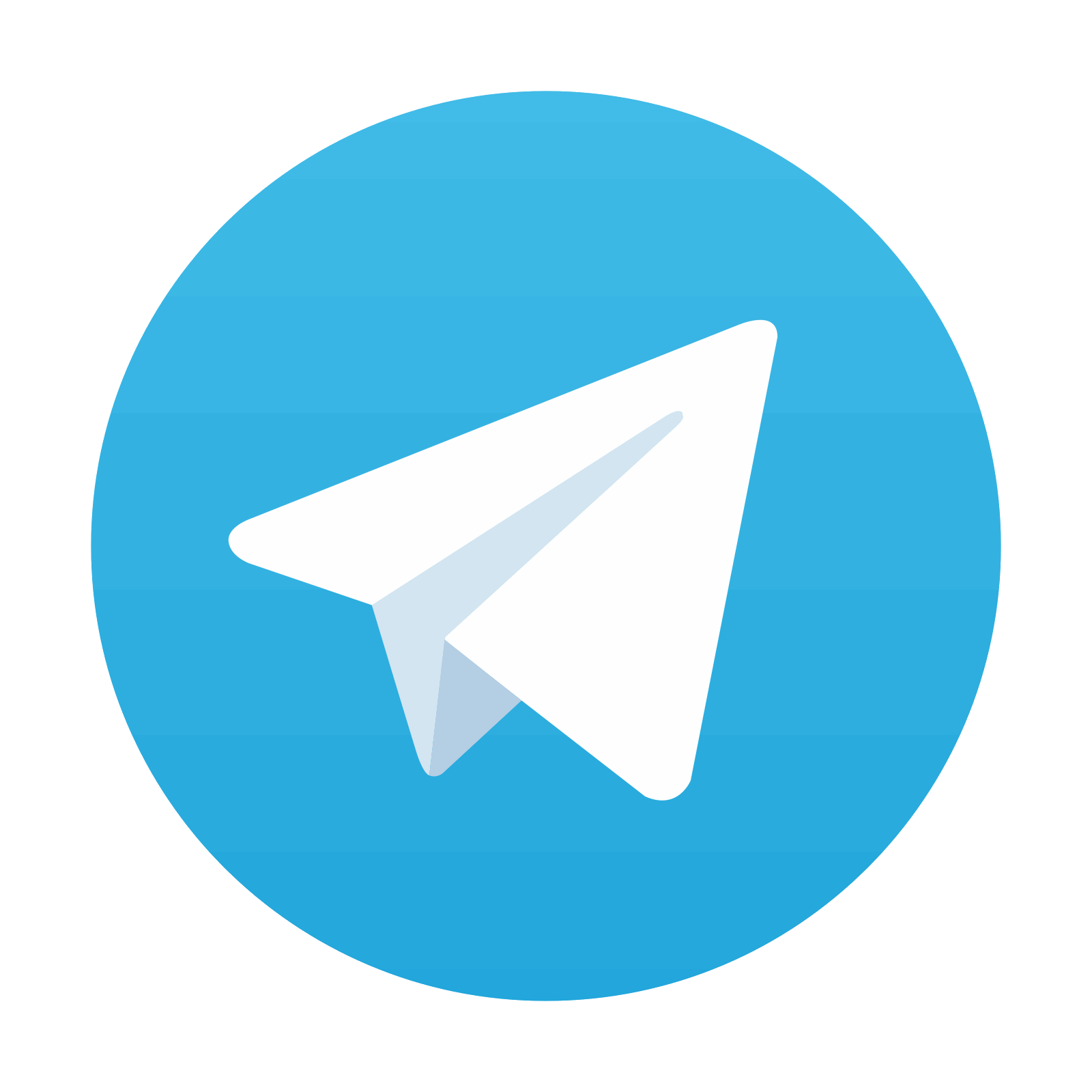
Stay updated, free articles. Join our Telegram channel

Full access? Get Clinical Tree
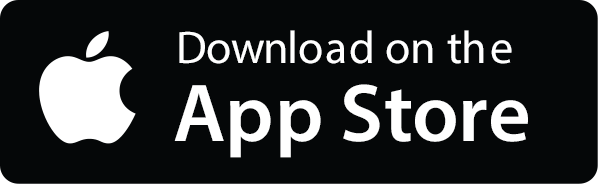
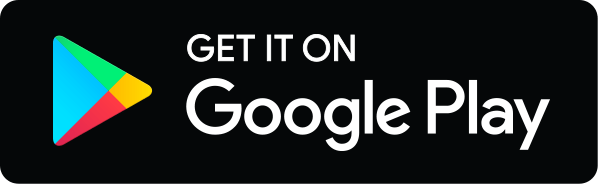