(4)
Paediatric Intensive Care Unit, Kremlin Bicetre Hospital, Assistance Publique-Hôpitaux, 78 rue du Général Leclerc, 94270 Le Kremlin Bicêtre, France
Continuous positive airway pressure experience is mostly derived from retrospective or small clinical studies. Reported results either include a few patients or focus on a single disease. As a result, evidences are lacking in order to define indications, techniques and complications of CPAP in children. Indeed, physiological response of infants and children to acute respiratory failure (ARF) cannot be extrapolated from adults, as shown later on.
41.1.1 Indications
The first published experience, in the early 1970s by Gregory et al., of CPAP in the management of respiratory distress of the newborn launches a new era of ventilatory support in the youngest patients (Gregory et al. 1971). Ventilatory support used by paediatric intensive care units during the last decades reveals that CPAP was rarely used outside the neonatal period and the chronic condition. Specific technical concerns, such as the lack of interface, have hindered for a long time CPAP application in the population of the 3–10 k of body weight. Moreover, the need to maintain a constant pressure during the entire cycle and uncontrolled air leaks has limited the potential benefit of CPAP technique (Gherini et al. 1979). The recent development of devices able to convert the kinetic energy of a jet of gas into a stable airway pressure has allowed the use of CPAP in the youngest (Moa et al. 1988; Klausner et al. 1996). The main aetiology of acute respiratory distress failure in patient of less than 1 year old is lower respiratory tract infections, mainly due to acute viral bronchiolitis. Beasley et al. described for the first time the use of CPAP in the management of 24 patients with severe bronchiolitis with a significant improvement in respiratory rate and gas exchange (Beasley and Jones 1981). During the 1990s, similar benefits were demonstrated by Soong et al. in further ten infants with severe bronchiolitis treated by nasal CPAP (nCPAP) (Soong et al. 1993). Using the Benveniste device (either mononasally or binasally) as a first intention ventilatory support, Kristensen et al. showed that in severely ill infants with viral bronchiolitis a reduced requirement of mechanical ventilation (1.3 %) when compared to previous studies from North America using invasive ventilation as a first support (Kristensen et al. 1998).
In the early 2000s, new interfaces (nasal prongs), which could be used in children over 3 kg of body weight, became commercially available. The nasal prongs facilitated noninvasive pressure support implementation to infants with severe bronchiolitis.
The helmet NIV interface, first developed for the adults, was recently available for children, with the new “baby body” (CaStar; Starmed, Mirandola, Italy), and used for nCPAP implementation in various aetiology of acute respiratory failure (Piastra et al. 2004, 2009).
In the last 5 years, several clinical studies have reported their experience in management of bronchiolitis with nCPAP (Campion et al. 2006; Larrar et al. 2006; Martinon-Torres et al. 2006; Cambonie et al. 2008; Thia et al. 2008). These studies are resumed in the Table 41.1. All studies showed an improvement in breathing pattern, and gas exchange, as well as a low rate of intubation. In addition, nCPAP has been shown to decrease respiratory muscle load in children with upper airway obstruction (Fauroux et al. 2001; Essouri et al. 2005), but no data has been published for young infants with more distal obstructive airway disease. Recently, Cambonie et al. showed that nCPAP can significantly decrease respiratory effort in 12 infants with severe bronchiolitis (Cambonie et al. 2008). We made similar observations in ten infants with acute bronchiolitis and further demonstrated that nCPAP can adequately decrease oesophageal and diaphragmatic pressure time product (PTPes and PTPdi), reflecting diaphragm oxygen consumption and work of breathing (WOB) (Essouri et al. 2011). Although there is no published consensus, all clinical and physiological data allow us to assert that nCPAP is a good respiratory support in infants of less than 1 year old with viral bronchiolitis.
Table 41.1
Clinical studies published on continuous positive airway pressure (CPAP)
Main primary diagnosis | Age (months) | Weigh (kg) | CPAP level (cm H2O) | CPAP duration (days) | CPAP failure (n) | |
---|---|---|---|---|---|---|
Larrar et al. (2006) (n = 53) | Bronchiolitis | 1.4 | 3.7 | 5 à 8 | 2.7 | 13 |
Campion et al. (2006) (n = 69) | Bronchiolitis | 1.6 | 3.9 | 4 à 8 | 2 | 12 |
Codazzi et al. (2006) (n = 15) | Post-extubation | 24 | 5–10 (helmet) | 2 | ||
Thia et al. (2008) (n = 31) | Bronchiolitis | 5 à 6 | ||||
Cambonie et al. (2008) (n = 12) | Bronchiolitis | 1.4 | 3.6 | 6 | 0 | |
Martinon-Torres et al. (2008) (n = 12) | Bronchiolitis | 4.7 | 7.2 ± 1.2 | 0 | ||
Javouhey et al. (2008) (n = 27) | Bronchiolitis | 1.3 | 5 à 10 | 2.2 | ||
Mayordomo-Colunga et al. (2009) (n = 78) | Bronchiolitis, asthma | 8 | 8 | 6.7 ± 1.2 | 6 |
41.1.2 Initiating CPAP
Implementation of CPAP respiratory support requires to answer to the following questions: (1) When CPAP should be initiated? (2) Which devices should be used? (3) In which setting should CPAP be initiated?
The timing of NPPV initiation in patients with neuromuscular disease has been well established in numerous studies (Robert et al. 1993; No authors listed 1999; Finder et al. 2004), but there is no similar data regarding the criteria for CPAP initiation during acute respiratory failure. However, it is certainly easier to define the non-indication for CPAP than to characterise the clinical parameters leading to the decision to initiate CPAP. Nevertheless, criteria used for nCPAP initiation in published experience of infants bronchiolitis are shown in Table 41.2. Interestingly, authors have quantified the extent of respiratory distress during acute bronchiolitis with the modified Wood’s clinical asthma score (m-WCAS) (Table 41.3) (Hollman et al. 1998; Martinon-Torres et al. 2002; Cambonie et al. 2006). Strength of m-WCAS resides in its use in all children, irrespective of the age, and the easiness of the scoring by any health-care providers. In addition, Cambonie showed that m-WCAS efficiently correlates with respiratory effort intensity measured by the oesophageal pressure (P es) swings, PTPes/breath and PTPes/mn. Interestingly, a significant correlation between the decrease in P es swings and use of accessory muscles score is also described (Cambonie et al. 2008). All published data are in agreement with recent experience showing that clinical signs of respiratory distress (increase respiratory rate and high transcutaneous PCO2) are correlated with an increase in respiratory muscles load and WOB (high oesophageal and diaphragmatic PTP) (Essouri et al. 2011). Based on published experiences, some criteria for the initiation of CPAP during paediatric acute respiratory failure can be suggested: alteration of gas exchange with PCO2 ≥50 mmHg, clinical respiratory distress with m-WCAS >5, increase in respiratory rate (≥2 SD) and bronchiolitis with recurrent apnea. However, CPAP should be contraindicated in the following conditions: cardiac or respiratory arrest, undrained pneumothorax, nonrespiratory organ failure (hemodynamic instability, severe encephalopathy) and inability to protect the upper airway.
Table 41.2
Clinical studies on severe acute bronchiolitis with nasal CPAP support
Larrar et al. (2006) (n = 53) | Campion et al. (2006) (n = 69) | Thia et al. (2008) (n = 31) | Cambonie et al. (2008) (n = 12) | Martinon-Torres et al. (2008) (n = 12) | |
---|---|---|---|---|---|
Baseline PCO2 (mmHg) | 62 | 69 | 55.8 | 64 | 61.6 |
Baseline RR (breaths/mn) | 61 | – | 53 | 61 | – |
Baseline pH | 7.3 | 7.27 | 7.3 | 7.29 | – |
Baseline HH (beats/mn) | 159 | – | 151 | 159 | – |
m-WCAS | – | – | – | 5.3 | 7.7 |
Table 41.3
Modified Wood’s clinical asthma score
Score | ||||
---|---|---|---|---|
0 | 0.5 | 1 | 2 | |
Cyanosis | SpO2 ≥95 % in room air | 95 % > SpO2 ≥90 % in room air | SpO2 ≥ 90 % with FiO2 >0.21 | SpO2 <90 % with FiO2 >0.21 |
Inspiratory breath sounds | Normal | Slightly unequal | Unequal | Decreased or absent |
Accessory muscles use | VAS <5 cm | 10 cm > VAS ≥5 cm | 15 cm > VAS ≥10 cm | VAS ≥15 cm |
Expiratory wheezing | VAS <5 cm | 11 cm > VAS ≥ 5 cm | 15 cm > VAS ≥10 cm | VAS≥15 cm |
Cerebral function | Normal | Agitated if disturbed | Depressed or agitated | Coma |
CPAP support requires four components: a ventilator or flow generator, a circuit, a humidifier and an interface. Theoretically, a simple flow generator with an external PEEP can be used to implement CPAP (Beasley and Jones 1981; Soong et al. 1993). Currently, many devices are commercially available, ranging from the simple jet CPAP to polyvalent ventilators used in intensive care unit. The most important parameter is to maintain a stable positive expiratory pressure during the whole respiratory cycle. There is no problem of patient-ventilator synchrony and inspiratory or expiratory trigger as we will see in the NPPV. Cambonie et al. describe that a significant decrease in oesophageal PTP is observed with Infant Flow® (Electro Medical Equipment Ltd, Brighton, United Kingdom) when the level of pressure is achieved (Cambonie et al. 2008). We also describe the same result in decreasing the oesophageal and diaphragmatic PTP with the Babylog 8000TM (Dräger, Lubeck, Germany) (Essouri et al. 2011). Humidification and warming of the gas delivered are critical even for nasal CPAP, especially when fresh gas is used. Most frequently, duration of CPAP support lasts from a few hours to days until respiratory failure resolves. In such circumstances, exposure to cold gas can lead to local inflammation and delayed healing of the respiratory mucosa (Hayes et al. 1995; Randerath et al. 2002). For young infants, the heated humidifier is the best adapted (Jaber et al. 2002; Lellouche et al. 2002). The circuit between the ventilator, or flow generator, and the interface can be single or double stranded and has to be adapted to the used interface. Prongs and small nasal masks are the most frequently used nCPAP interfaces. The two main systems used in Europe are Infant Flow® (Electro Medical Equipment Ltd, Brighton, United Kingdom) and Fisher & Paykel Healthcare (Auckland, New Zealand). The second one provides prongs available for infants weighing more than 3 k of body weight. A more recent interface is the helmet with the new “baby body”. The modified helmet that seems to be a suitable device for delivering CPAP is well tolerated without major side effects. However, only a feasibility study on 15 children has been published (Codazzi et al. 2006), and no data are available for infants. Several questions remain pendent regarding the helmet system: (1) What is the physiological consequence CO2 rebreathing? (2) What is the minimal body weight considering that in the smallest, interface overlap may alter thoracic compliance?
As the primary endpoint of the CPAP is to avoid severe respiratory failure leading to invasive ventilation, in patients with an acute respiratory failure, CPAP should be initiated as soon as possible. Thia et al. compared evolution between two groups receiving either early CPAP or delayed CPAP after 12 h of standard therapy. They observed a significant decrease in PCO2 following early CPAP when compared to delayed CPAP, suggesting that early treatment has prevented the development of additional airway collapse, slowing up and reversing the natural progression of the disease (Thia et al. 2008). Different situations may arise depending on the delay of admission to a PICU. In the last few years, French emergency transport teams were trained to use nCPAP before transfer to an experienced PICU. Although no international recommendation has been published, it can be anticipated that early CPAP, before and outside the intensive care unit, can prevent further respiratory deterioration. The 2001 International Consensus Conferences on noninvasive positive pressure ventilation in adults ARF have stated that “the optimal location for patients receiving NPPV depends upon the capacity for adequate monitoring, staff skill and experience in explaining the procedure, their knowledge of the equipment used, and awareness of potential complications” (Evans 2001).
41.1.3 Airway Pressure Adjustments During CPAP Therapy
The physiologic data emanating from studies are scarce, and currently level of CPAP is defined empirically determined on the basis of clinical tolerance and clinical response. Shortly, the CPAP level should be set up to the pressure level achieving a decrease in both respiratory muscle load and work of breathing without increasing air leaks. In published experiences of nCPAP for acute viral bronchiolitis, summarised in Table 41.1, level of CPAP was between 5 and 7 cm H2O. This pressure level was shown to be effective and safe in infants with mild respiratory distress (Courtney et al. 2003; Martinon-Torres et al. 2006; Cambonie et al. 2008; Martinon-Torres et al. 2008). Based on the recent work of Cambonie et al., we actually know that the level of 6 cm H2O is adapted for infants with acute obstructive airway distress (Cambonie et al. 2008). With this level of CPAP, there is a homogeneous decrease in the values of inspiratory effort of 53 % for Pes swing, 58 % for PTPes/breath and 59 % for PTPes/mn. These data are in agreement with our own in 10 infants with bronchiolitis managed with a mean level of CPAP of 7 cm H2O. We observed a significant and homogeneous reduction of oesophageal and diaphragmatic swings from, respectively, 48 and 50 % and from 55 % for oesophageal PTP and 53 % for diaphragmatic PTP (Fig. 41.1). For each infant, we also measured physiological data with high level of CPAP (until 10 cm H2O) without increase in respiratory effort and in seven infants decrease in the comfort score measured. With low level of CPAP, we observed a decrease in the improvement of oesophageal and diaphragmatic PTP (Essouri et al. 2011). Therefore, based on published clinical experience and physiological data, the recommended level of CPAP for the management of severe acute bronchiolitis is around 7 cm of water.
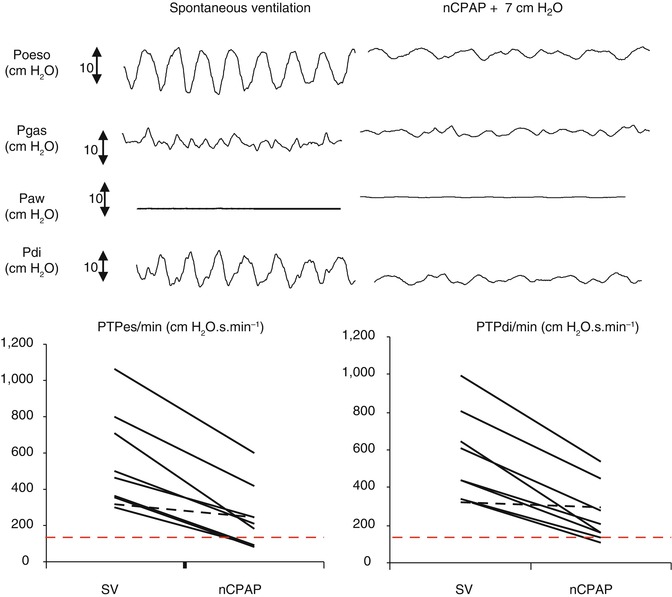
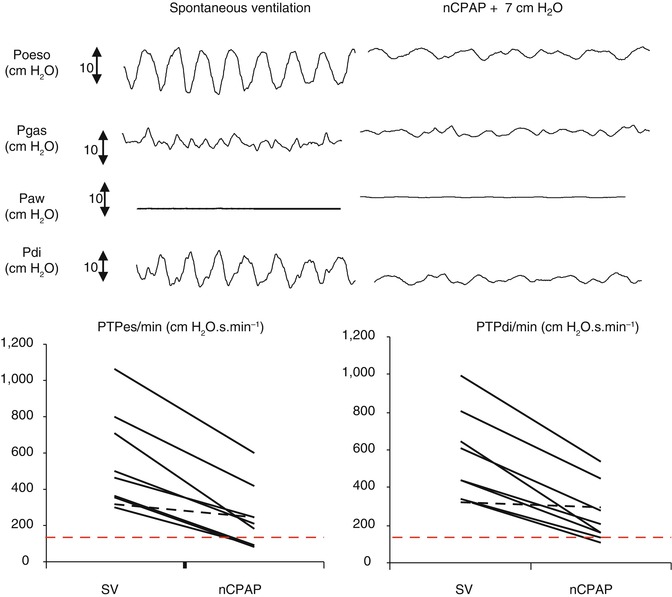
Fig. 41.1
Physiological effect of nasal CPAP support (nCPAP) on respiratory effort evaluated by PTPes and PTPdi measured during spontaneous breathing (SB) and nCPAP
An additional question is unanswered: Is the level of CPAP support dependant of the age and weight of the patient? The only published data (neonates excluded) are issued from patients with upper airway obstruction. We showed in ten patients that there was no correlation between optimal level of CPAP, defined by the maximum decrease in oesophageal and diaphragmatic PTP and patient age (Essouri et al. 2005).
41.1.4 CPAP Failure Criteria
Incidence of CPAP supports failure, usually defined by the necessity to support respiratory function through invasive ventilation (i.e. orotracheal intubation and mechanical ventilation), has progressively decreased, from 17 to 33 % in the early years of the CPAP therapy for bronchiolitis (Campion et al. 2006; Larrar et al. 2006; Javouhey et al. 2008) to 5 % in the most recent years. Factors associated with the reduction of CPAP failure are now becoming clearer: early CPAP, improvement of interface and health-care provider training in NIV (Essouri et al. 2011).
Despite the recent decrease in failure of CPAP for bronchiolitis, early identification of CPAP failure remains critical as patients requiring invasive ventilatory support have increased morbidity. Some studies have been performed in paediatric patients but are referring to bi-level NPPV (see Sect. 2.2.4). Concerning CPAP support, only retrospective studies are available and concerns acute bronchiolitis (Bernet et al. 2005; Campion et al. 2006; Larrar et al. 2006). A prospective study of NIV (both CPAP and NPPV) in 116 paediatric patients identified three independent risk factors for NIV failure: type 1 acute respiratory failure (defined by Teague (2003)), high PRISM score, and a lower respiratory rate decrease during NIV (Mayordomo-Colunga et al. 2009). However, this study did not look separately to CPAP and NPPV failure. Interestingly, we have reported that high PRISM score during the first 24 h and no and/or a small decrease in PCO2 during CPAP support were significantly associated with CPAP failure during acute viral bronchiolitis (Larrar et al. 2006). Similar results were found by Campion et al., who showed additionally apnea and higher PCO2 as risk factors for CPAP failure (Campion et al. 2006) (Table 41.4).
Table 41.4
Failure of CPAP and NPPV supports
CPAP failure criteria | NPP failure criteria | |
---|---|---|
Before NPPV | During NPPV | |
Required FiO2 ≥80 % | Type 1 ARF | Increase FiO2 needs |
No or small decrease in PCO2 | ARDS | Lower RR decrease |
Lower RR decrease | High PRISM score | Lower PCO2 decrease |
Recurrent apnea | ||
High PRISM score at H24 of PICU stay |
41.1.5 Undesired Side Effects
Paediatric patients are prone to a wide range of undesired side effects due to physiologic characteristics related to immaturity of the airway protective reflex, high nasal resistance and tendency to mouth breathe (Teague 2003). Frequent side effects are summarised in the Table 41.5. However, most studies involving young children showed an excellent tolerance to nCPAP. Indeed, skin damage (bridge of the nose, nasal mucosa and upper lip) remains the most frequent complication of CPAP. Fauroux et al. observed that in a population of children with chronic NPPV, skin damage was related to the use of commercial masks (Fauroux et al. 2005). Therefore, reducing the risk of skin damage is among the priority in order to improve tolerance of CPAP. Among the strategy to prevent skin damage, avoiding excessive tight fit is mandatory (Nava et al. 2009). Although frequently mentioned as a complication of CPAP, no pneumothorax was described in the six published clinical studies looking at nCPAP support for acute bronchiolitis (Campion et al. 2006; Larrar et al. 2006; Martinon-Torres et al. 2006; Cambonie et al. 2008; Javouhey et al. 2008; Thia et al. 2008). Agitation and difficulty to adapt to the interface were described but disappeared with the use of oral sedation (30–56 %) (Campion et al. 2006; Thia et al. 2008; Mayordomo-Colunga et al. 2009).
Table 41.5
Main side effects of CPAP and practical ways to reduce them
Undesired side effects of CPAP | Ways to reduce |
---|---|
Skin damage | Adapted size, protection by hydrocolloid |
Excoriation around the nose | Rotate various types of interfaces |
Air leaks | Good position |
Eye irritation | Good position |
Nasal dryness causing thick secretions | Humidification and reducing airflows if possible |
Gastric distension | Gastric tube deflation |
41.1.6 Practical Hints for CPAP Applications
A protocol designed for the medical and nursing staff is required. Clear indications for CPAP initiation and technical hints for how to initiate and maintain CPAP should be established based on the following suggestions (see Fig. 41.2):
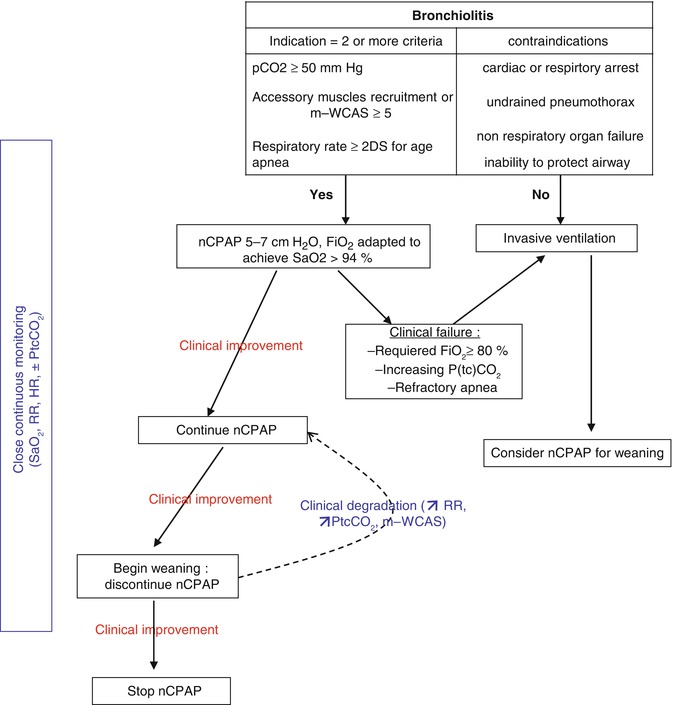
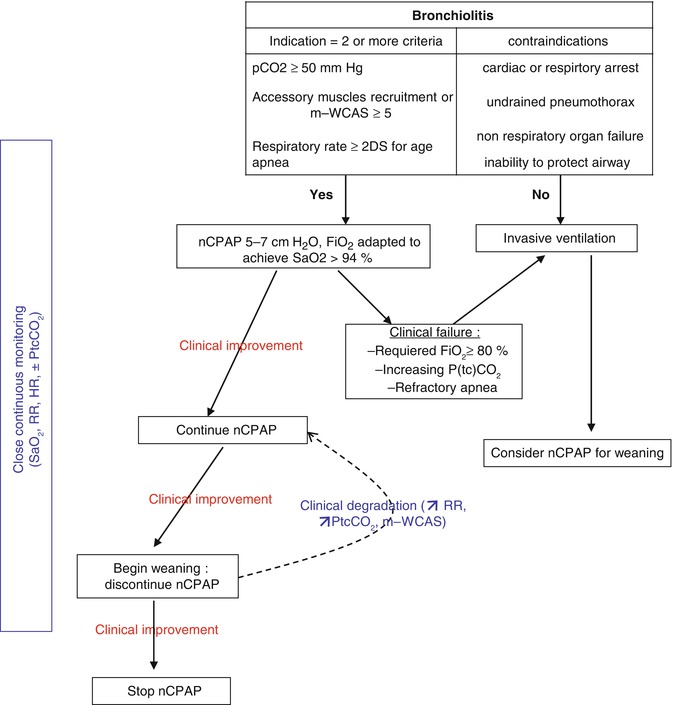
Fig. 41.2
Practical use of nCPAP for severe acute bronchiolitis
Evaluate indication for CPAP.
Explain to the parents.
Start monitoring (pulse oximetry, heart rate, respiratory rate, transcutaneous CO2 if available).
Select the adequate interface, hold it in place with the adequate size of cap.
Set up the ventilator/pressure generator (PEEP 5–7 cm H2O, minimal flow 12 L/mn).
Adjust FiO2 to maintain SpO2 between 90 and 98 %.
Perform clinical and gas exchange assessment after 2 h of CPAP.
The success depends of the quality of the health-care training. Regular sessions are required to optimise the success of CPAP implementation.
41.2 Noninvasive Positive Pressure Ventilation
Sandrine Essouri5
(5)
Paediatric Intensive Care Unit, Kremlin Bicetre Hospital, Assistance Publique-Hôpitaux, 78 rue du Général Leclerc, 94270 Le Kremlin Bicêtre, France
41.2.1 Indications
During the last decade, NPPV has been increasingly used to provide ventilatory support in various groups of patients with respiratory failure. In adults, recommendations for NPPV in ARF were published (Evans 2001). In paediatrics, no such recommendation exists, although the French Societies of Paediatric Intensive Care and of Pulmonology have recently published a common statement for NPPV support in children with laryngomalacia. Indeed, NPPV support in children with ARF may provide significant support. Children are at increased risk of respiratory failure due to the relative instability of the developing respiratory system (Hershenson et al. 1990; Devlieger et al. 1991). The functional residual capacity in young infants is close to the total lung capacity because the chest wall is highly compliant (Davis et al. 1988; Papastamelos et al. 1995) and the immature lung has a high elastic recoil (Agostoni 1959). In addition, the diaphragmatic distribution of muscle fibre type 1 is unfavourable with fewer fatigue-resistant units (Le Souef et al. 1988) making young infants prone to respiratory distress and alveolar hypoventilation. However, little has been published on the physiological response induced by the use of NPPV in ARF. As previously shown, respiratory muscle load can be evaluated using the oesophageal and gastric pressure recorded by a nasogastric tube with two integrated pressure transducers (see Fig. 41.1). The diaphragmatic PTP and oesophageal PTP are obtained by measuring the area under the diaphragmatic pressure signal and oesophageal pressure signal between the onset of the inspiration, defined as the point at which occurred the deflection on the Pes trace, and the end of the inspiration defined as the peak of Pdi. Analysis of respiratory mechanics in a group of 12 patients with ARF treated with NPPV, showed in all the patients, a decrease in dynamic compliance and an increase in the different components of the work of breathing regardless of the underlying aetiology (Essouri et al. 2008).
Published experience of NPPV support in children with ARF is heterogeneous. Respiratory failure was secondary to a variety of pathology, including severe community-acquired or hospital-acquired pneumonia, post-extubation ARF, status asthmaticus, acute chest syndrome in sickle-cell disease (ACS), chest trauma and acute respiratory distress syndrome (ARDS). In addition patient characteristics, such the age and weight also varied indeed, despite the wide heterogeneity of the studied population, NPPV seemed to be well tolerated, with a low rate of intubation (between 9 and 43 %) (Fortenberry et al. 1995; Padman et al. 1998; Thill et al. 2004; Bernet et al. 2005; Chin et al. 2005; Essouri et al. 2006). However, published data do not suggest that all causes of ARF do well respond to NPPV. In a large cohort of 114 children, ARDS was identified as an independent risk factor of NPPV failure in children (Essouri et al. 2006). This is in agreement with data in adults (Antonelli et al. 2001; Ferrer et al. 2003) and was confirmed in a recent prospective study looking at 116 children with NPPV support for ARF (Mayordomo-Colunga et al. 2009). They found that type 1 ARF (characterised primarily by hypoxaemia with a low arterial PaO2 and normal to low arterial PaCO2), and a high PRISM score were independent risk factors for NPPV failure. Therefore, acute hypoxaemic respiratory failure does not seem to be a valid indication for NPPV use. All other causes of ARF seem to respond to NPPV. Current indications for NPPV in children with ARF are summarised in Table 41.6.
Table 41.6
Indications and contraindications of NPPV in acute respiratory failure in children
Indications of NPPV | Contraindications of NPPV |
---|---|
Post-extubation ARF | Cardiac or respiratory arrest |
Atelectasis, postoperative diaphragmatic dysfunction | Nonrespiratory organ failure |
Community-acquired or health-care associated pneumonia | Severe encephalopathy (e.g. GCS <10) |
ARF in immunocompromised patient | Severe upper gastrointestinal bleeding |
Acute chest syndrome (sickle-cell disease) | Hemodynamic instability |
Status asthmaticus | Facial surgery, trauma or deformity |
Left ventricular dysfunction | Fixed obstruction of upper airway |
Near drowning | Inability to cooperate/protect the airway |
Inability to clear respiratory secretions | |
High risk for aspiration | |
No-drained pneumothorax | |
Excessive secretions | |
Uncooperative or agitated |
41.2.2 Initiating Noninvasive Positive Pressure Ventilation
Three parameters should be taken into account for NPPV success: the choice of an adapted interface, the ventilator and the ventilatory mode. A well-fitted comfortable interface is crucial to ensure success of NPPV. Nasal masks have advantages over oronasal masks, such as greater comfort, reduced risk of claustrophobia and better expectoration. However, nasal masks are more prone to air leakage through the mouth and have been associated with a higher rate of initial intolerance during acute applications of NPPV in adults (Kwok et al. 2003). Because children with respiratory failure breathe mostly through the mouth, in order to bypass high nasal resistance, during ARF oronasal interfaces are preferred. In adults with hypoxaemic respiratory failure, the helmet has been demonstrated to be as efficient as the face mask. Physiological data suggested that, when compared to the face mask use, helmet resulted in greater inspiratory muscle effort. Nonetheless, when both pressures support and PEEP level are increased, inspiratory efforts are similar (Vargas et al. 2009). The place of the helmet interface for NPPV with bi-level pressure support has not been established in paediatric patients. Although, the modified helmet (baby body) interface seems to be suitable for CPAP delivery in children, patient-ventilator asynchrony makes its use for NPPV delivery more hazardous. Published experience of NPPV with helmet interface in children is limited. It has been used in immunocompromised children in a small clinical study with four patients, with age ranging between 9 and 17 years old, showing that the use of the helmet circumvents the disadvantages of the face mask, while maintaining the indications and benefits of a true pressure support ventilation (Piastra et al. 2004). Recently, 26 immunocompromised children with ARDS receiving NPPV with either an oronasal mask (n = 10) or an helmet (n = 13) failed to identify any difference between both interfaces (Piastra et al. 2009). In the later study, all children were over 9 years old allowing good patient-ventilator synchrony, even with the helmet interface.
One of the key differences between invasive and NIV are the air leaks. Air leaks cause problems during the different phases of a respiratory cycle. During the initial phase, it causes delayed triggering or missed trigger effort, auto-triggering. Leaks can also decrease rate of inspiratory pressure rise and when they are large enough, delays cycling to the expiration phase in normal and obstructive conditions. Finally, leaks can reduce positive end-expiratory pressure. Ventilators adapted for NPPV are those able to counteract the above-mentioned consequences of air leaks. There are three general classes of ventilator that can be used for NPPV (Chatburn 2009):
1.
Home care ventilators, such as piston-driven ventilators (e.g. Lifecare PLV-100, Saime Eole 3.), have been the standard home care ventilator for decades. They are typically used in volume mode, but some of them can be set to deliver pressure control ventilation. These ventilators are easy to use, offer adequate alarms and can operate on battery power but require an oxygen bleed-in modification to control FiO2. The major concern is that they are not designed to compensate for leaks which may cause triggering problems, especially in young children. They are therefore not suited for use in ARF.
2.
Devices specially designed for NPPV called “noninvasive ventilators” including: simple noninvasive ventilators, most often used at home in non-acute settings, and advanced NPPV ventilators (e.g. Respironics Vision) which additionally offer control of FiO2, graphic monitoring and alarms. The latter are most often used in intensive care units. These ventilators are adapted for use in children.
3.
Finally, ICU ventilators which were originally designed to ventilate intubated patients with minimal or no leaks. With the growing use of NPPV, some newer ICU ventilators (e.g. Maquet Servo-i, Dräger Evita 4, Evita XL, Hamilton G5, Viasys Vela) have noninvasive ventilatory modes. When activated, these NPPV modes automate leak compensation and deactivate some nuisance alarms. Although NIV modes can compensate most interference, Vignaux et al., in a study evaluating the performance of NNPPV modes in all current ICU ventilators commonly distributed in Europe, showed that leaks still impair several key functions of ICU ventilators (Vignaux et al. 2007). Altogether, best choices for NPPV support of children with ARF are represented by the ICU ventilators with NIV option or advanced NPPV ventilators if available.
In paediatric ARF, no recommendation regarding the mode of NPPV support is available, but in practice, pressure support ventilation is the mode usually use by most of us. Although, tidal volume (Vt) is more stable in volume control mode with changing lung mechanics, pressure control mode permits a more stable volume delivery with leak and better patient-ventilator synchrony because pressure control allows the patient to adjust inspiratory flow (Chatburn 2009). Most ventilators suitable for NPPV offer various ventilatory modes, such as CPAP mode, spontaneous, timed and spontaneous/timed operating modes. In the spontaneous mode, the ventilator responds to a threshold level of inspiratory flow that is initiated by the patient’s spontaneous respiratory effort. The ventilator then delivers additional gas flow to reach the preset inspiratory positive airway pressure. Exhalation occurs after the inspiratory flow peaks and then decreases to a threshold level. In the timed mode, the ventilator does not trigger in response to flow changes but delivers intermittent positive airway pressure at a set rate. In the spontaneous/timed mode, the flow trigger feature is activated; the ventilator cycles in the timed mode only in the event of prolonged apnea or low minute ventilation. Paediatric patients are most often managed with NPPV in the spontaneous/timed mode. A significant decrease in oesophageal and diaphragmatic PTP was found during spontaneous/timed mode NPPV in 12 children with ARF (Essouri et al. 2008). The high trigger sensitivity of the ICU ventilator allows good patient-ventilator synchrony even for small children.
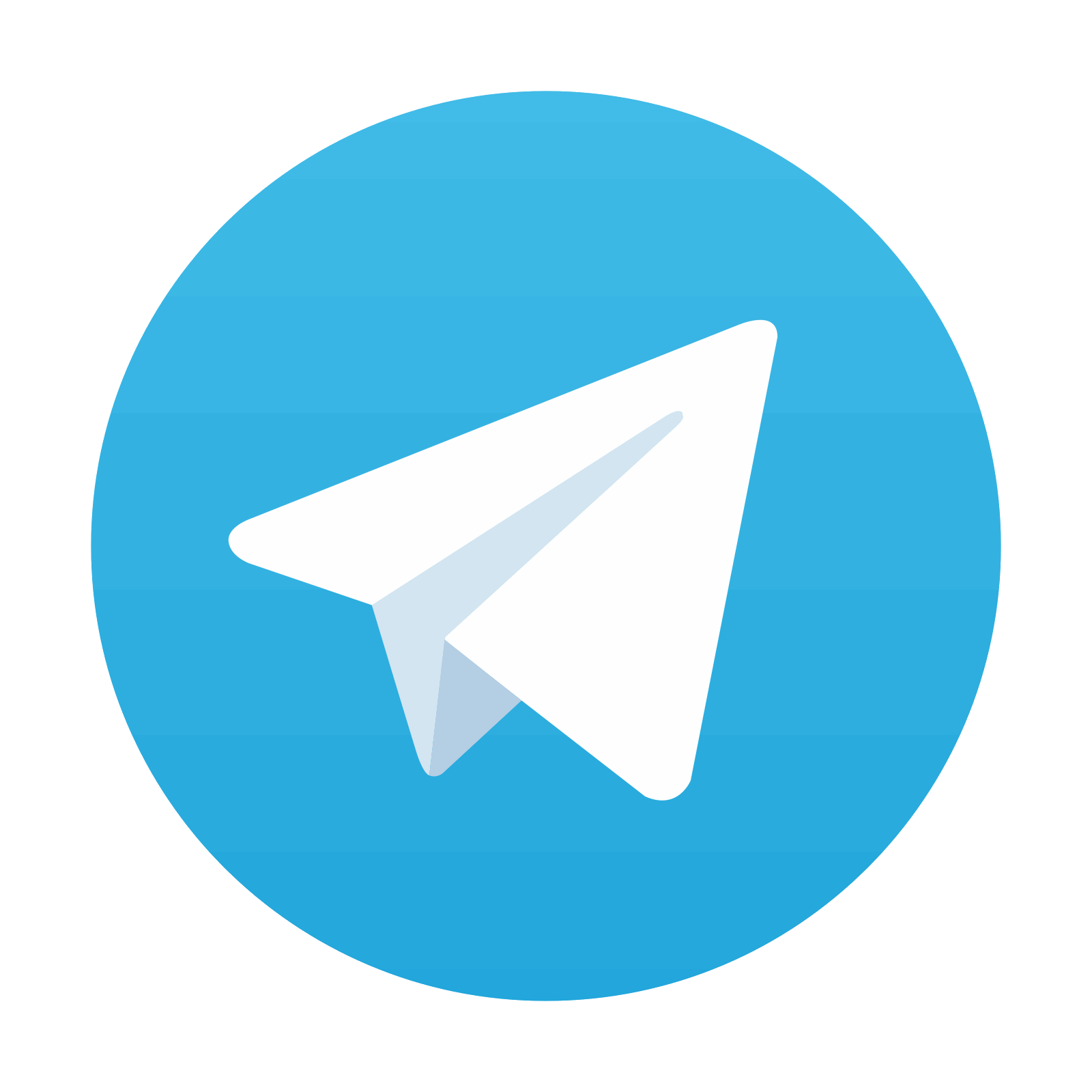
Stay updated, free articles. Join our Telegram channel

Full access? Get Clinical Tree
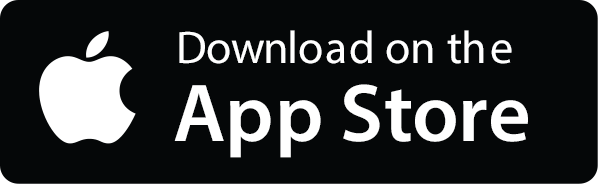
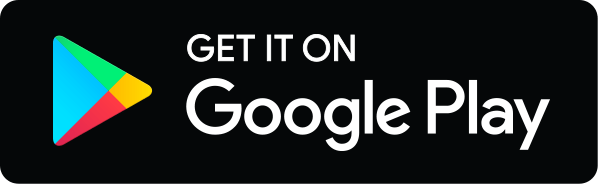