Our concept of nucleic acid biology has advanced dramatically over the past two decades, with a growing appreciation that cell-free DNA (cfDNA) fragments are present in all body fluids including plasma. In no other field has plasma DNA been as rapidly translated into clinical practice as in noninvasive prenatal testing (NIPT) for fetal chromosome abnormalities. NIPT is a screening test that requires confirmation with diagnostic testing, but other applications of cfDNA provide diagnostic information and do not require invasive testing. These applications are referred to as noninvasive prenatal diagnosis (NIPD) and include determination of fetal sex, blood group and some single gene disorders. As technology advances, noninvasive tests based on cell-free nucleic acids will continue to expand. This review will outline the technical and clinical aspects of NIPT and NIPD relevant to the daily practice of maternity carers.
Highlights
- •
Cell free DNA – the biological basis and the sequencing techniques behind NIPT.
- •
Clinical performance of NIPT for the common automosomal trisomies and beyond.
- •
Essential pre-test counselling for NIPT.
- •
When NIPT is not be the preferred test.
- •
“No-call” and discordant results.
Introduction
Cell-free DNA (cfDNA) and RNA fragments are present in all body fluids in a dynamic state of constant turnover. CfDNA is comprised of small fragments of extracellular DNA that circulate freely in the bloodstream . Technological progress in molecular genetics has enabled scientists to utilize this uniquely accessible genetic material for a whole new generation of blood tests that detect and monitor disease. In the field of obstetrics, this circulating DNA can be used for the noninvasive detection of fetal genetic abnormalities such as trisomy 21. In what has been a very rapid translation into clinical practice, noninvasive prenatal testing (NIPT) has revolutionised prenatal screening. Providers of prenatal care need to be familiar with the technical and clinical aspects of NIPT and these will be reviewed here.
Voluntary prenatal screening for fetal anomalies is a key component of antenatal care in developed countries and offers couples the option of obtaining more genetic information about their pregnancy. Although for some this information is not desired, for others, screening for common chromosome conditions such as Down syndrome (trisomy 21) provides an important opportunity to inform management. As diagnostic testing with amniocentesis or chorionic villus sampling (CVS) carries a cost and a procedural risk of miscarriage, screening tests are offered to better target diagnostic testing. Traditional screening approaches for trisomy 21 have considerable room for improvement, with detection rates of approximately 80–90%, for an average false positive rate of 5%. These performance characteristics translate to a substantial proportion of missed diagnoses and false positive “high risk” results leading to unnecessary invasive diagnostic testing .
Research efforts over the past two decades have therefore focused on developing a noninvasive yet highly accurate screening test that would increase detection and reduce false positive results. The combination of the discovery of cfDNA of fetal/placental origin in 1997 and the rapid advances in DNA sequencing technology have combined to make this noninvasive approach a clinical reality in less than 15 years.
Biology of cfDNA
The majority of cfDNA in plasma is derived from haematopoietic cells that release fragments of DNA during cell turnover, but a large variety of solid organs also contribute to the circulating plasma pool . In the non-pregnant state, the genomic profile (i.e. the proportional representation of each chromosome) in plasma cfDNA simply reflects the individual’s karyotype and the size of each chromosome. During pregnancy however, cfDNA from the placenta is also released into the maternal plasma and this can be used to detect fetal chromosomal or genetic abnormalities ( Figure 1 ).

The existence of cfDNA of fetal/placental origin in maternal plasma was first demonstrated through the detection of Y-chromosome specific sequences . These DNA fragments, which originate from the outer cytotrophoblast, are released into the maternal circulation during apoptosis . CfDNA arising from the placenta – so-called “cell-free fetal DNA” (cffDNA) – is detectable in maternal plasma from as early as 5 weeks gestation and comprises 10–15% of the total plasma cfDNA . CffDNA levels rise gradually with increasing gestation and are cleared quickly from the maternal circulation after delivery . The circulating fetal DNA therefore represents the current pregnancy and does not persist from prior pregnancies.
Biology of cfDNA
The majority of cfDNA in plasma is derived from haematopoietic cells that release fragments of DNA during cell turnover, but a large variety of solid organs also contribute to the circulating plasma pool . In the non-pregnant state, the genomic profile (i.e. the proportional representation of each chromosome) in plasma cfDNA simply reflects the individual’s karyotype and the size of each chromosome. During pregnancy however, cfDNA from the placenta is also released into the maternal plasma and this can be used to detect fetal chromosomal or genetic abnormalities ( Figure 1 ).
The existence of cfDNA of fetal/placental origin in maternal plasma was first demonstrated through the detection of Y-chromosome specific sequences . These DNA fragments, which originate from the outer cytotrophoblast, are released into the maternal circulation during apoptosis . CfDNA arising from the placenta – so-called “cell-free fetal DNA” (cffDNA) – is detectable in maternal plasma from as early as 5 weeks gestation and comprises 10–15% of the total plasma cfDNA . CffDNA levels rise gradually with increasing gestation and are cleared quickly from the maternal circulation after delivery . The circulating fetal DNA therefore represents the current pregnancy and does not persist from prior pregnancies.
Nomenclature
Prenatal testing based on analysis of cffDNA in the maternal plasma has been called noninvasive prenatal testing (NIPT) or noninvasive prenatal screening (NIPS) to distinguish it from traditional diagnostic methods of directly assessing the fetal karyotype using cells obtained via amniocentesis or CVS. Testing for fetal aneuploidy with cffDNA in maternal plasma is a screening test – it does not achieve diagnostic accuracy for aneuploidy and requires confirmation with pre or postnatal karyotyping.
Some applications of cffDNA can provide fetal diagnostic information and do not require subsequent confirmation with invasive testing. These applications are called noninvasive prenatal diagnosis (NIPD) and are currently primarily confined to the detection of paternally inherited conditions in the fetus (i.e. unique fetal DNA sequences that are not present in the woman’s genotype), though research in the diagnosis of recessive conditions and de novo mutations continues to progress .
Traditional aneuploidy screening methods
The autosomal aneuploidies trisomy 21 (Down syndrome), trisomy 18 (Edward syndrome) and trisomy 13 (Patau syndrome) are the three most common chromosome abnormalities to affect ongoing pregnancies. Trisomy 21 makes up approximately half of all prenatally diagnosed chromosome abnormalities and individuals with this condition have intellectual disability and a range of medical morbidities. Maternal age is the most important risk factor for trisomy 21. By the maternal age of 40, the risk of delivering an affected term newborn with trisomy 21 is 1% . For the next two most common autosomal trisomies, trisomies 18 and 13, stillbirth or death in the first year of life is the usual outcome. For these reasons, screening for the common autosomal aneuploidies is offered routinely in most developed countries .
Screening approaches for aneuploidy have evolved significantly over the last few decades. Combined first trimester screening (cFTS) can be performed at 11–13 + 6 weeks of gestation and incorporates maternal age, the ultrasound measurement of the fetal nuchal translucency and measures of the maternal serum analytes pregnancy-associated plasma protein A (PAPP-A) and free β-HCG, to generate overall risk figures for trisomies 21, 13 and 18 . In the second trimester between 15–20 weeks of gestation, maternal age in combination with four serum analytes can similarly provide an overall risk figure for trisomy 21, 18, 13 or the presence of a neural tube defect . Some countries offer integrated screening pathways where components of both the first trimester and second trimester tests are used to provide an overall risk estimate or, alternatively, to provide a stepwise risk assessment.
The traditional cFTS was until recently the screening test with the highest detection rate (85–90%) and lowest false positive rate (5%) for trisomy 21 . Additional markers such as the fetal nasal bone, the ductus venosus waveform and tricuspid valve flow were subsequently developed to improve the detection rate for trisomy 21 to 96% and lower the false positive rate to 2.5%, but these markers were not widely used outside large tertiary centres .
In 2011, noninvasive prenatal testing using cffDNA in maternal plasma became clinically available in the United States and China and disrupted the traditional prenatal screening paradigm. Over the past five years, this innovative test has spread globally to over 60 countries, and its use has expanded to both high risk and general risk populations.
NIPT for detection of trisomy 21, 18 and 13
The fundamental principle of NIPT for trisomy 21 is the detection of an excess number of DNA fragments from chromosome 21 in the maternal plasma (i.e. a counting approach). A fetus affected with trisomy 21 will release relatively more chromosome 21 DNA fragments into the maternal circulation compared to other chromosomes because of the presence of a third copy of chromosome 21 in the trophoblast. DNA sequencing of maternal plasma cfDNA, which contains a mixture of maternal and fetal DNA, allows a precise estimate of the fetal dosage of chromosome 21 (i.e. two or three copies) by counting the fragments arising from each chromosome. The number of fragments counted from chromosome 21 are then compared to a reference derived from other presumed diploid chromosome(s), and statistical analysis is performed to determine if there are more chromosome 21 counts than expected.
There are a number of different NIPT platforms for aneuploidy detection, including random whole genome or massively parallel sequencing (MPS), targeted or chromosome-selective sequencing (CSS) and single nucleotide polymorphism (SNP)-based sequencing. As the technology disseminates globally, variations in sequencing methods and bioinformatic algorithms are appearing, but the three main approaches are summarized here.
- a)
MPS or random whole genome sequencing
In the whole genome MPS approach, short stretches, or “reads”, of randomly selected plasma DNA fragments are performed simultaneously (“massively parallel”), enabling millions of DNA molecules to be sequenced quickly at single nucleotide resolution . Only a fraction of each DNA fragment is actually sequenced (typically 36 base pairs) but this is sufficient to map a DNA fragment to a unique location in the human genome and thus identify its chromosome of origin .
In 2008, two groups demonstrated that fetal trisomies could be identified using random MPS of maternal plasma . Although in this technique, the entire plasma mixture of maternal and fetal DNA fragments is sequenced without distinguishing between their origins, the precision of MPS is sufficient to detect the small proportional increase in chromosome 21 fragments due to the trisomic placenta.
Statistically, MPS methods calculate the standard deviation of the expected count from each chromosome and allocate a “z-score” for each chromosome. If the number of DNA fragments from chromosome 21 in the test sample is more than 3 standard deviations away from expected (i.e. z-score >3), this is considered a high risk result for trisomy 21 .
- b)
Chromosome-selective (targeted) sequencing
The CSS approach was developed to improve the efficiency of the sequencing (or counting) step. In this method, the plasma DNA fragments undergo an enrichment process, so that only preselected fragments from chromosomes 13, 18 and 21 and the sex chromosomes are read and sequenced, rather than the entire plasma mixture which contains DNA from all chromosomes. The enrichment step involves a PCR (polymerase chain reaction)-based technique that amplifies segments of DNA that are unique to the targeted chromosome. The final DNA mixture that undergoes sequencing is therefore highly concentrated for chromosomes 21, 18, 13, X and Y, thus reducing the cost and time of DNA sequencing . The statistical method for CSS (Fetal fraction optimized risk of trisomy evaluation, FORTE) also differs from MPS. CSS uses the woman’s prior risk of aneuploidy (based on maternal and gestational age), the target chromosome counts, and the fetal fraction to calculate a final risk using an odds ratio approach. The NIPT result is provided as a risk result (rather than a categorical result), with a risk of 1 in 100 or greater being defined as high risk .
- c)
SNP-based NIPT
A SNP is a variation in a single base pair in a given stretch of DNA that occurs in >1% of the population. SNPs are widely used in forensics as biological markers for “DNA fingerprinting” of individuals. In SNP-based NIPT, DNA is extracted separately from the maternal plasma (containing mixed maternal and fetal cfDNA) and maternal leucocytes (pure maternal cellular DNA). Targeted amplification and analysis of ∼20,000 SNPs on chromosomes 21, 18, 13, X and Y is performed on these samples and the variations at each SNP locus measured. The fetal genotype is then deduced by comparing the sequencing results from maternal leucocytes with plasma. By comparing the observed SNP distributions in plasma to those expected, the most likely fetal karyotype can be identified . The SNP-based method is therefore different to the MPS and CSS methods as it distinguishes between maternal and fetal sources of the DNA and can also incorporate paternal information.
Each NIPT approach described above has particular features that may affect the clinician’s choice of assay. The random MPS method is able to provide dosage information on any chromosome, not just those reported in the standard NIPT panels. These “off-target” abnormalities can be maternal or fetal in origin, and involve whole chromosome or subchromosomal abnormalities depending on the depth of sequencing and bioinformatics used . However, the targeted approaches (CSS or SNP-based NIPT) will not detect off-target abnormalities, limiting the potential for incidental findings. SNP-based NIPT is the only assay able to detect triploidy and can also be used for parent of origin analysis of aneuploidy. It is unsuitable however for situations where there are more than two possible genotypes represented in plasma – such as twin pregnancy, egg donor pregnancy, or past maternal organ transplant.
Fetal fraction
An important factor influencing the laboratory performance of NIPT is the fetal fraction, which is the proportion of total cfDNA in maternal plasma that is derived from the fetus/placenta . If the fetal fraction is low, it is more difficult for NIPT to detect an aneuploid fetus. There are several biological factors that influence fetal fraction, including gestational age, maternal weight, and fetal aneuploidy . Maternal weight has a well-recognized negative impact on fetal fraction: approximately 7% of women weighing 100 kg have a fetal fraction <4% which is a minimum threshold for issuing a report for some laboratories. This increases to 50% when maternal weight reaches 160 kg . Further clinical implications of failed NIPT results due to low fetal fraction are discussed later in this paper.
Clinical performance of NIPT
- a)
Sensitivity and specificity
The performance of NIPT for aneuploidy screening is highly accurate and far better than standard prenatal screening approaches. A systematic review and meta-analysis published in 2016 found the pooled sensitivity of NIPT for trisomy 21 was 99.3%, for trisomy 18, 97.4% and for trisomy 13, 97.4% . The American College of Medical Genetics and Genomics accordingly recommends informing all pregnant women that NIPT is the most sensitive screening option for trisomies 13, 18 and 21 .
The specificity of NIPT is also very high at 99.9% for trisomy 21 . A highly specific test means fewer false positive results, which is desirable given the anxiety and additional testing that occurs when women receive a “high risk” screen result. When looking at the performance of NIPT panels as a whole, false positive rates are cumulative with each additional chromosome assessed. The X chromosome in particular, contributes the most to the false positive rate due to maternal age-related loss and undiagnosed maternal mosaicism. The overall false positive rate for tests assessing chromosomes 21, 18, 13 and the sex chromosomes is 0.72% or approximately 1 in 140 .
- b)
Positive predictive value
The positive predictive value (PPV) is the chance of a woman with a high risk NIPT result actually having an affected fetus. Although sensitivity and specificity are inherent to the test itself, the positive and negative predictive values depend on the population being tested and the pre-test probability of disease. The PPV correlates with the background prevalence of disease and will therefore be lower in low risk women. The PPV is lower for chromosomes 13, 18 and the sex chromosomes as these all have a higher false positive rate than chromosome 21.
The clinical validity of NIPT was initially established in high risk populations, in which the PPV would be expected to be high (80–90% for trisomy 21) . Recent data show that NIPT far outperforms cFTS in the general all risk population as well . In a cohort with average maternal age of 30 years (where the risk of trisomy 21 is 1 in 700), the PPV for NIPT was 45.5% for trisomy 21 and 40% for trisomy 18 . Accordingly, all professional bodies recommend that high risk results be confirmed with diagnostic testing before decisions such as termination of pregnancy are made .
- c)
Negative predictive value
If a couple receives a negative (“low risk”) screen result, the likelihood that the fetus does have one of the aneuploidies in question is very low . Although a low risk result is very reassuring, it does not completely exclude an affected fetus. False negatives for trisomy 21 would be expected to occur at a rate of approximately 1 in 10,000 in a high risk population with a 1 in 100 prevalence. This rate of false negatives appears to be borne out in the few clinical studies that are large enough to contain a number of false negative cases, though no large study has attempted completed ascertainment on women with low risk results .
- d)
Interpretation and management of “no call” results
A small number of women who undergo NIPT will not receive a result for reasons including low fetal fraction, sample administration issues and laboratory assay failure . The most common reason for a “no call” is a low fetal fraction. An adequate fetal fraction – approximately 2% to 4% – is essential for an accurate result . Several biological factors such as maternal weight, multiple pregnancy and gestational age influence the fetal fraction . The type of NIPT platform also influences the test failure rate. Published test failure rates are highest in targeted SNP-based assays (6.4%) and lowest in platforms using a whole genome/MPS approach (1.6%) .
Some specific fetal aneuploidies, such as triploidy and trisomy 13, are also associated with a lower fetal fraction and hence a higher test failure rate . Therefore, women with a “no call” result due to a low fetal fraction may actually be at increased risk of aneuploidy and therefore require additional counselling.
Increasing maternal weight reduces the fetal fraction and so obese women are also more likely to get a “no call” result. Overweight women should be counselled pretest about their higher failure rate and in some cases offered alternative screening. In the most recent meta-analysis, the failure rate ranged from 0% to 12.7% and among 5789 women who had an initial test failure and were resampled, 13.9% also failed on repeat testing .
Management of failed results needs to address the potential causes of a low fetal fraction and the woman’s individual risk for aneuploidy. Options include offering (i) an alternative screening test such as cFTS if within the appropriate gestational age window; (ii) detailed ultrasound assessment for signs of fetal aneuploidy; and (iii) diagnostic testing given the association of a low fetal fraction with fetal aneuploidies . Repeat sampling for NIPT may be successful in over 50% of cases but may introduce a deleterious delay in management if repeat NIPT failure occurs, and is not recommended by the American College of Medical Genetics and Genomics . However, if all failed tests lead to invasive testing, the screen positive rate approaches that of conventional cFTS, thus negating one of the key benefits of NIPT .
Clinical implementation models for NIPT
Overall, three models have been proposed for incorporation of NIPT into current screening programs. The first model uses NIPT as a second tier screening test where women with a high risk result from conventional screening are subsequently offered NIPT or diagnostic testing. The advantage here is that only ∼5% of women would be offered NIPT, thus reducing cost . The second model is as a universal primary screening test in combination with a 12 week ultrasound . This provides the highest detection rate of aneuploidy but at a higher cost. A third model attempts to strike a balance between cost and detection rate. In this contingent model women undergo primary screening with cFTS. Those with a high risk result on cFTS (eg ≥ 1 in 100) would be directly offered a choice of diagnostic testing, NIPT or no further testing, and those in the intermediate-risk group (eg 1 in 101–2500) would be offered cfDNA testing or no further testing .
The RAPID project in the UK assessed the performance of NIPT as a contingent screen after a cFTS or second trimester screening result of 1 in 1000 or higher . Offering NIPT as a contingent screen, led to a higher detection rate for trisomy 21, a lower rate of invasive diagnostic tests and fewer miscarriages due to amniocentesis or CVS for a non-significant difference in cost . As a result, the UK National Screening Program has recommended that government funded NIPT be introduced for all women with an initial aneuploidy risk of greater than 1 in 150. Similar evaluations of NIPT are being conducted in the Netherlands and in Canada but at the time of writing, NIPT is not part of a publicly funded prenatal screening program in any country.
In the USA, most Health Maintenance Organisations are funding NIPT and the American College of Medical Genetics and Genomics have given approval for NIPT to be offered to all risk women . The increasing use of NIPT as a screening test for aneuploidy has seen a dramatic drop in the number of invasive diagnostic tests being performed, creating implications for training and skills maintenance of proceduralists .
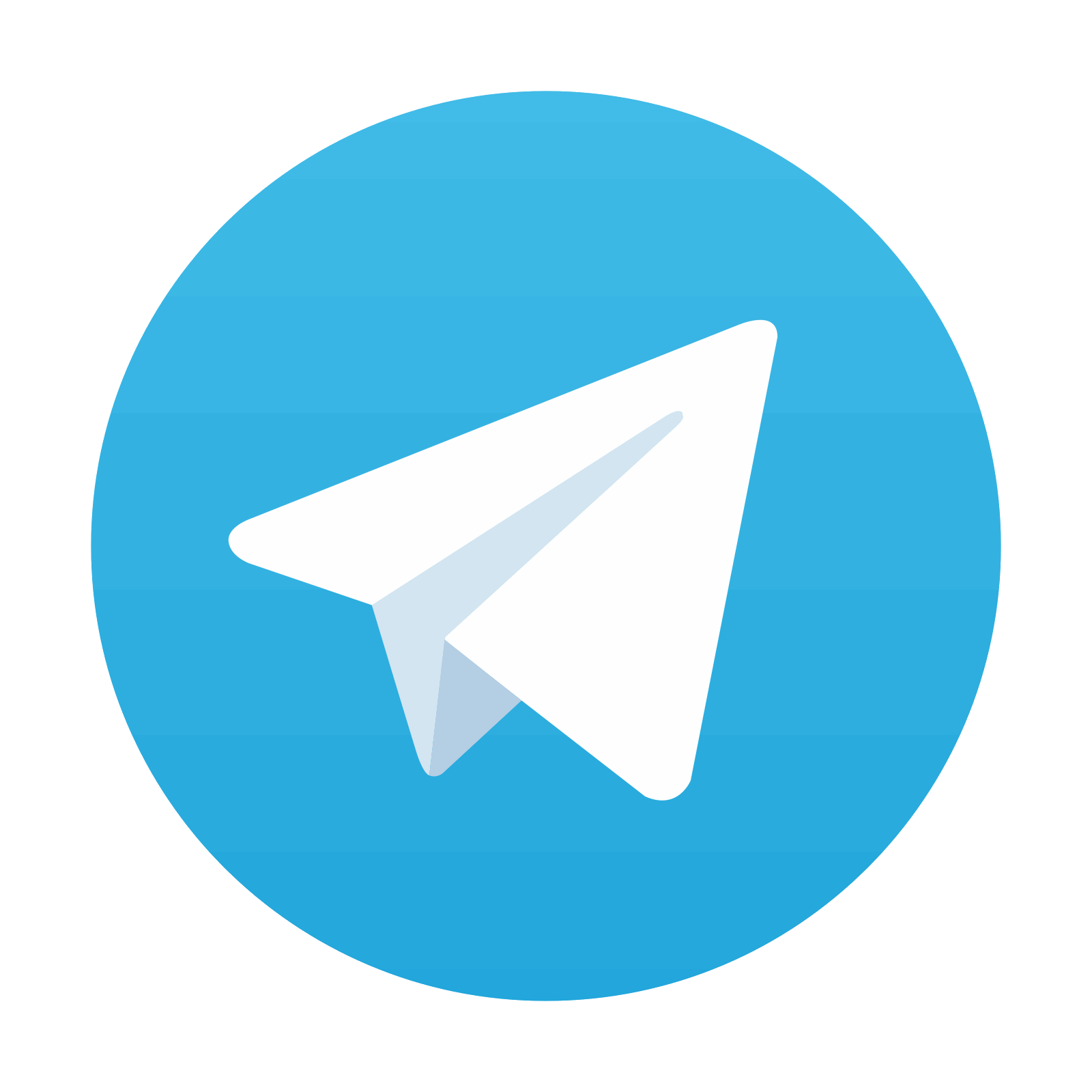
Stay updated, free articles. Join our Telegram channel

Full access? Get Clinical Tree
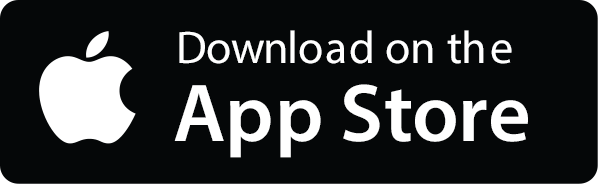
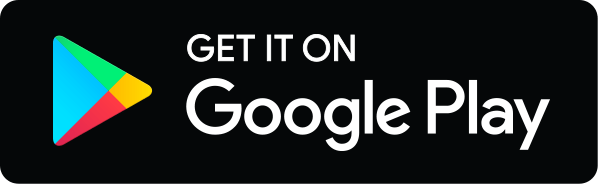