The past few years have seen extraordinary advances in prenatal genetic practice led by 2 major technological advances; next-generation sequencing of cell-free DNA in the maternal plasma to noninvasively identify fetal chromosome abnormalities, and microarray analysis of chorionic villus sampling and amniotic fluid samples, resulting in increased cytogenetic resolution.
Noninvasive prenatal screening of cell-free DNA has demonstrated sensitivity and specificity for trisomy 21 superior to all previous screening approaches with slightly lower performance for other common aneuploidies. These tests have rapidly captured an increasing market share, with substantial reductions in the number of chorionic villus sampling and amniocentesis performed suggesting that physicians and patients regard such screening approaches as an equivalent replacement for diagnostic testing. Simultaneously, many clinical programs have noted significant decreases in patient counseling.
In 2012 the Eunice Kennedy Shriver National Institute of Child Health and Human Development funded a blinded comparison of karyotype with the emerging technology of array comparative genomic hybridization showing that in patients with a normal karyotype, 2.5% had a clinically relevant microdeletion or duplication identified. In pregnancies with an ultrasound-detected structural anomaly, 6% had an incremental finding, and of those with a normal scan, 1.6% had a copy number variant.
For patients of any age with a normal ultrasound and karyotype, the chance of a pathogenic copy number variant is greater than 1%, similar to the age-related risk of aneuploidy in the fetus of a 38 year old. This risk is 4-fold higher than the risk of trisomy 21 in a woman younger than 30 years and 5- to 10-fold higher than the present accepted risk of a diagnostic procedure. Based on this, we contend that every patient, regardless of her age, be educated about these risks and offered the opportunity to have a diagnostic procedure with array comparative genomic hybridization performed.
Progress in science predictably leads to improvements in medical care. Historically, new laboratory tests and procedures go through 2 major phases. First, there is a phase of development in which investigators have an idea leading to a testable hypothesis. This is followed by testing, refinement, patent applications, and publication of results. Once proof of concept is accepted and the product is ready for translation to care, creation of a market occurs based on demand for the new approach. This is followed by a phase of diffusion in which the new concept moves into the broader community.
Predictably, as the new concept is adopted by practicing physicians and their patients, there is a rapid expansion of utilization with variable levels of physician training and understanding, resulting in performance somewhat less than that initially projected. Eventually, with increasing community exposure, there is improvement in performance and better recognition of the appropriate integration into care.
Over the past several years, 2 important tools in screening and testing for fetal chromosomal abnormalities have developed on separate tracks: microarray analysis of chorionic villus sampling and amniotic fluid samples, resulting in increased cytogenetic resolution, and next-generation sequencing of cell-free DNA in the maternal plasma to noninvasively identify common fetal chromosome abnormalities ( Table 1 ).
Methods | Description |
---|---|
Array comparative genomic hybridization (also known as microarray) | Array comparative genomic hybridization is a molecular cytogenetic technique used for the determination of DNA copy number (deletions or duplications). Array comparative genomic hybridization can interrogate for the presence or absence of much smaller segments of DNA than can be seen by karyotype. There are enormous differences in the resolution of array comparative genomic hybridization methods varying from nearly whole chromosomes that are virtually indistinguishable from levels provided by fluorescent in situ hybridization to very high resolutions. Low resolution is used for fresh transfers in preimplantation diagnosis with in vitro fertilization and by some laboratories on amniocentesis and chorionic villus sampling specimens. High resolution is currently possible only on direct fetal material such as amniocentesis and chorionic villus sampling. The higher the resolution, the greater the finding of abnormalities but the greater the incidence of variants of uncertain significance segments. By lowering the resolution, the variants of uncertain significance segment percentage goes down, but so does the finding of abnormalities. Because the amount of DNA is much higher in chorionic villus sampling specimens than amniocentesis, chorionic villus sampling array comparative genomic hybridization usually has a faster result time than amniocentesis. |
Next-generation sequencing (also called Next Gen) or massively parallel sequencing | These are similar approaches of modern sequencing techniques through which an entire genome can be sequenced within a day. Next-generation sequencing platforms sequence millions of small fragments of DNA simultaneously in parallel. Bioinformatics can then identify the origin of the fragments and map them to the reference human genome. Each of the DNA bases are sequenced multiple times so that variation in the genome can be detected. |
Massively parallel sequencing | This uses a next-generation platform and bioinformatics to categorize the origin of fragments. By determining the number of fragments from specific chromosomes (or portions of chromosomes) and comparing this with the expected contribution, additional or missing chromosomal material can be diagnosed. For example, chromosome 21 normally contributes about 1.32% of all the DNA. If there is 2% in a sample, this suggests 3 copies of chromosome 21. This is one approach used in noninvasive prenatal screening, also called cell-free fetal DNA. |
Selective sequencing and selective probes | In another approach to noninvasive prenatal screening, fragments from only selected chromosomes of interest (eg, 21, 18, 13) are sequenced or probed. These probes or sequencing for chromosome (eg, 21, 18 and 13) combined with the fraction of fetal DNA in the maternal circulation are used to screen for fetal aneuploidy. Noninvasive prenatal screening methods can all vary by the depth of discrimination detected and/or reported (ie, how small a fragment difference they can evaluate). All of these have high sensitivities for detecting trisomy 21 but lower performance for other trisomies (eg, trisomies 13 and 18 and sex chromosomes) and much lower performance for microdeletions and duplication syndromes (eg, DiGeorge, Prader-Willi, Angelman). |
Single-nucleotide polymorphisms | These are single base pair differences in the genome and are the most common type of DNA variation in man. |
Copy number variants | These reflect changes in sequences of DNA: duplications or deletions. These copy number variants can be clearly pathological, ambiguous, or benign. |
Variants of uncertain significance | The percentage of ambiguous copy number variants are called variants of uncertain significance. These have decreased in percentage from about 2% several years ago to now less than 1% as with increasing experience, formerly variants of uncertain significance segments can now be classified as pathological or benign. |
These technologies have had widely divergent pathways toward incorporation into prenatal practice. Before widespread introduction into care, fetal microarray analysis was vetted through blinded National Institutes of Health–funded trials performed by agnostic investigators. This approach was similar to that taken with other paradigm-changing prenatal diagnostic and therapeutic modalities such as chorionic villus sampling, first-trimester aneuploidy screening using biochemistry and nuchal translucency, antenatal steroids for fetal lung maturation, progesterone for the prevention of preterm birth, and many others.
On the other hand, cell-free DNA screening has been a laboratory-developed test marketed by the developers to the practitioner and patient communities without multiple, independent studies and trials validating its performance prior to its introduction into the marketplace.
This commentary represents the opinions of the 3 authors who have each been involved in prenatal diagnosis and screening for more than 30 years. It is intended to give our perspective on the relative advantages and disadvantage of the emerging technologies and to present potential pathways to clinical translation. Because many of the terms used may be new to many readers, we provide a short explanation of relevant ones ( Table 1 ).
Improved diagnostic capability: chromosomal microarrays
The resolution of prenatally performed standard karyotypes is limited by the use of light microscopy and is typically quoted at about 7 million base pairs but in clinical, prenatal practice may be 10 million base pairs or higher. Molecular cytogenetic analyses such as array comparative genomic hybridization (array comparative genomic hybridization, or microarrays) does not require direct visualization of the chromosomes and has the ability to identify much smaller cytogenetic changes such as microdeletions or duplications (ie, copy number variants). Technically, microarrays can identify copy number variants less than 200,000 base pairs (200 Kb), but in clinical prenatal practice, findings of 500 kb to 1 million base pairs are routinely reported.
Over the past decade, because of its increased diagnostic yield (sensitivity) array, comparative genomic hybridization has virtually replaced the karyotype in the evaluation of dysmorphic neonates and infants and children with neurocognitive disorders such as autism, intellectual disability, and epilepsy. The probability of finding the underlying cause is more than triple that of the standard karyotype: from about 3–4% by karyotype to 15–20% by array comparative genomic hybridization.
Prenatally, array comparative genomic hybridization is now the recommended first-tier test in the evaluation of fetuses with an anatomic anomaly diagnosed by ultrasound. Studies have shown that in these patients, array comparative genomic hybridization will identify all unbalanced cytogenetic abnormalities identified by a karyotype in addition to finding a clinically relevant copy number variant, not seen on karyotype, in 6–8% of patients.
Although specific microdeletions or duplications are individually rare, collectively they are more common than the classic trisomies and can have equally serious phenotypes. For women younger than 35 years, these microdeletions and duplications are more common than standard chromosome abnormalities such as Down syndrome.
To evaluate the efficacy of this technology, the Eunice Kennedy Shriver National Institute of Child Health and Human Development sponsored a multicenter, blinded study published in 2012 that compared array comparative genomic hybridization to karyotype in routine prenatal practice. In pregnancies without ultrasound, anomalies sampled for routine reasons such as advanced maternal age, positive biochemical, and nuchal translucency screening or maternal anxiety, pregnancies with a normal standard karyotype were found to have a pathogenic copy number variant in greater than 1% of cases. Subsequent studies have confirmed these findings.
As a result we believe that routine offering of array comparative genomic hybridization to all patients is a standard of care option. Attempts by some insurance companies to claim that it is still investigational despite numerous publications and hundreds of thousands of cases are not reasonable. Such claims follow a pattern seen several times previously such as with low maternal serum alpha-fetoprotein screening, multiple marker screening, nuchal translucencies, and chorionic villus sampling. In the 1980s and 1990s, many insurance companies fought for several years to avoid paying for these tests long after most obstetricians considered it required to be offered.
The ability to accurately interpret submicroscopic copy number variants was initially questioned because previously undescribed findings of unclear significance were found. However, with experience the clinical relevance of the majority of these findings is now known. For example, the original Eunice Kennedy Shriver National Institute of Child Health and Human Development cohort had a 2.5% frequency of copy number variants interpreted as being uncertain. A review of these same copy number variants today would interpret less than 1% as uncertain: a rate comparable with that seen with standard prenatal karyotype interpretation.
Noninvasive prenatal screening
Background
Since the sentinel observation of Lo et al in 1997 that cell-free DNA fragments of placental origin are detectable in maternal plasma, noninvasive prenatal screening has focused on this source of material for prenatal evaluation. Presently 2 general approaches are used. Multiple parallel shotgun sequencing examines large numbers of DNA fragments (both maternal and fetal), identifies their chromosomal origin, and by counting the number of fragments from each chromosome can reliably determine an increased contribution from a fetal trisomic chromosome. Modifications of this approach include the preselection of fragments from specific targeted chromosomes prior to sequencing and the use of microarray technology rather than sequencing to determine the relative contributions of each chromosome.
In the second approach, single-nucleotide polymorphisms on the chromosomes of interest are utilized to identify the fetal and maternal contributions, and a complex algorithm is used to predict the probability of fetal aneuploidy.
Noninvasive prenatal screening
Background
Since the sentinel observation of Lo et al in 1997 that cell-free DNA fragments of placental origin are detectable in maternal plasma, noninvasive prenatal screening has focused on this source of material for prenatal evaluation. Presently 2 general approaches are used. Multiple parallel shotgun sequencing examines large numbers of DNA fragments (both maternal and fetal), identifies their chromosomal origin, and by counting the number of fragments from each chromosome can reliably determine an increased contribution from a fetal trisomic chromosome. Modifications of this approach include the preselection of fragments from specific targeted chromosomes prior to sequencing and the use of microarray technology rather than sequencing to determine the relative contributions of each chromosome.
In the second approach, single-nucleotide polymorphisms on the chromosomes of interest are utilized to identify the fetal and maternal contributions, and a complex algorithm is used to predict the probability of fetal aneuploidy.
Performance characteristics of noninvasive prenatal screening
For the detection of common aneuploidies, the sensitivity of noninvasive prenatal screening exceeds any other screening approach, with detection of greater than 99% of trisomy 21 pregnancies and 98% of trisomy 18 gestations. This is accomplished with an average false-positive rate less than 1–2 per 1000 (specificity 99.8–99.9%). However, because the vast majority of the initial studies were performed in pregnancies at high risk for aneuploidy or used populations enriched with excess Down syndrome cases, there has been confusion among patients and some providers about the meaning of a positive noninvasive prenatal screening.
The primary misunderstanding in noninvasive prenatal screening interpretation is the failure to consider that the disorders being screened for are rare and that even though the test has very high sensitivity and specificity, a positive result does not necessarily mean an affected pregnancy. In fact, even in a high-risk population such as woman older than 35 years of age, the actual probability of an affected pregnancy following a positive noninvasive prenatal screening (ie, positive predictive value) is only about 67%.
In the general population in which the probability of trisomy 21 is approximately 1 in 800, the positive predictive value is less than 20%. For rarer disorders such as trisomy 18 and 13 with somewhat lower sensitivity and specificity, the positive predictive value would be even less. Failure to consider this has led to a general misconception that a positive noninvasive prenatal screening effectively replaces diagnostic testing such as amniocentesis or chorionic villus sampling.
Many clinicians using noninvasive prenatal screening as their primary screening tool are also eschewing combined first-trimester serum screening (free β-human chorionic gonadotropin, pregnancy-associated plasma protein A, and nuchal translucency). In addition to losing the advantage that the first-trimester scan provides of establishing accurate dates and diagnosing multiple gestations, many major structural anomalies easily detected at 12–13 weeks are not being discovered until later in gestation.
In addition, first-trimester combined screening will identify genetic and congenital abnormalities not evaluated by noninvasive prenatal screening such as congenital heart defects, Noonan syndrome, and other cytogenetic abnormalities. For example, Alamillo et al have shown that approximately 20% of patients with positive combined screening for trisomy 21 will have karyotype abnormalities other than the common aneuploidies screened for by noninvasive prenatal screening. In addition, about half of these atypical karyotypes will have a normal nuchal translucency measurement, demonstrating the independent value of the biochemical analytes.
Similarly, Norton et al evaluated more than 1 million woman having sequential, biochemical, and nuchal translucency screening (free β-human chorionic gonadotropin, pregnancy-associated plasma protein A, and nuchal translucency) under the auspices of the California prenatal screening program. Approximately 17% of woman with a positive sequential screen had a karyotype abnormality other than trisomies 21, 18, or 13 or sex chromosome abnormalities, leaving them with a slightly greater than 2% residual risk of aneuploidy if noninvasive prenatal screening had been performed rather than chorionic villus sampling or amniocentesis to evaluate the sequential screen.
This calculation does not include microdeletions or duplications, which, although not screened for, may be present in all pregnancies and would increase the risk of an unidentified cytogenetic abnormality by another 1%.
In another study in which noninvasive prenatal screening and sequential screening were compared in their ability to predict all chromosome abnormalities, sequential screening demonstrated a 10% greater detection rate. In our opinion this strongly suggests that patients having positive combined or sequential screening should be offered a diagnostic procedure as their initial option, and those choosing noninvasive prenatal screening must be made aware of the residual risk if noninvasive prenatal screening is negative. In addition, it is premature to abandon biochemical/nuchal translucency screening, especially in a low-risk population in which the frequency of common aneuploidies is low.
Another evolving practice is that some patients with fetal structural anomalies discovered at a second-trimester scan are being offered noninvasive prenatal screening instead of diagnostic testing with array comparative genomic hybridization. This approach fails to look for all of the chromosome anomalies that can lead to these disorders or the 6–8% of cases in which a microdeletion or duplication will be found. Identification of 1 of these cytogenetic abnormalities may significantly change the prognosis for the pregnancy, may suggest alternative treatment approaches, and will facilitate meaningful counseling.
In recognition of the importance of copy number variants in prenatal screening, the companies currently providing noninvasive prenatal screening in the US market have begun to add a search for microdeletions to their panel. Companies have taken 1 of 2 different approaches to this expansion of their screening capability. Some have added whole-genome evaluation in which the contribution of DNA from all chromosomes is considered and will report any copy number variant over 7 Mb. Others have chosen to identify a limited number of copy number variants with specific well-defined phenotypes.
At present the performance characteristics of these additional tests have not been evaluated in sufficiently sized prospective, clinical trials with full outcome data collected. Because of the rarity and small size of any single microdeletion, the ability of noninvasive prenatal screening to reproduce diagnostic microarray results will require deeper sequencing or the addition of an extensive number of probes.
The evaluation of large numbers of these smaller segments of the genome is likely to cause an overall increase in the false-positive rates, even with a specificity of greater than 99% for detecting each condition. Preliminary experience has already shown that for some microdeletion/duplication syndromes, the positive predictive value will be extremely low.
For example, screening for the relatively frequent microdeletion associated with DiGeorge syndrome, which has a population frequency potentially as high as 1:1000, will have a positive predictive value of only 5%. At present, detection of the limited microdeletions presently available by noninvasive prenatal screening is not equivalent to the performance of testing on direct fetal samples.
By our (unpublished) estimate, the existing data suggest that the microdeletions presently detectable by noninvasive prenatal screening are less than 1/1000th of what a microarray on fetal tissue reveals. Increasing the detection rate to that available by diagnostic testing will necessarily be associated with a substantial increase in false-positive results. Depending on how high that rate is and how many microdeletions can be detected will determine the usefulness of this approach as compared with diagnostic testing.
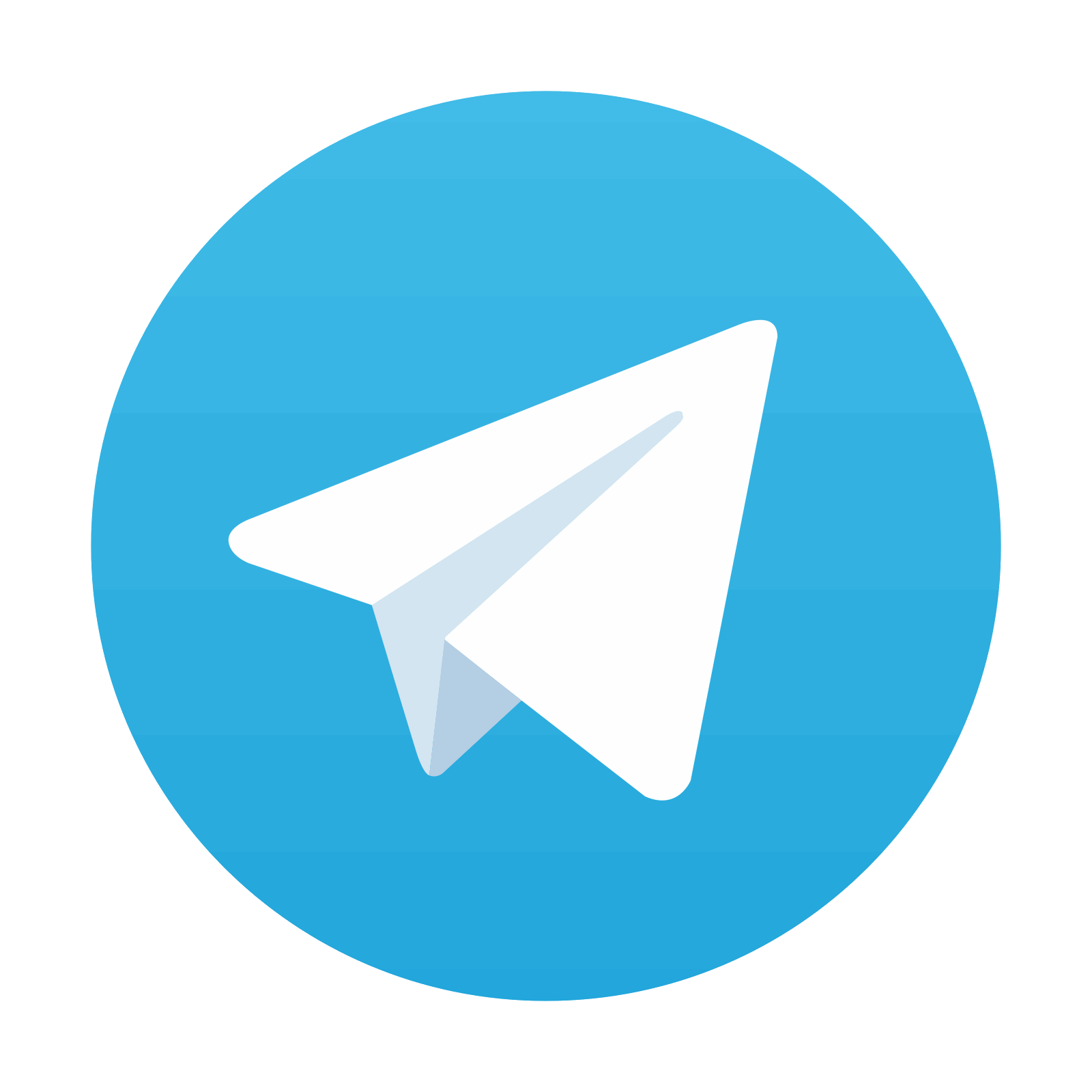
Stay updated, free articles. Join our Telegram channel

Full access? Get Clinical Tree
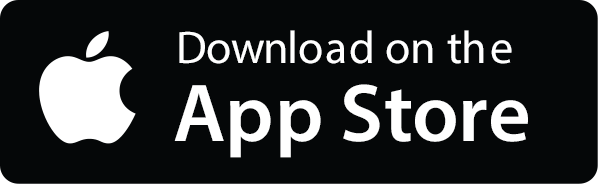
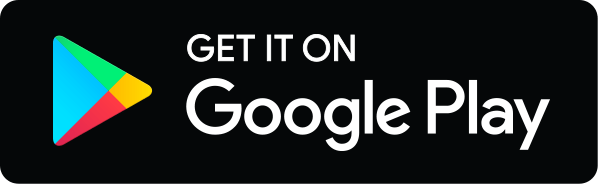