Cystic fibrosis transmembrane conductance regulator (CFTR) modulators are clinically available personalized medicines approved for some individuals with cystic fibrosis (CF) to target the underlying defect of disease. This review summarizes strategies used to develop CFTR modulators as therapies that improve function and availability of CFTR protein. Lessons learned from dissemination of ivacaftor across the CF population responsive to this therapy and future approaches to predict and monitor treatment response of CFTR modulators are discussed. The goal remains to expand patient-centered and personalized therapy to all patients with CF, ultimately improving life expectancy and quality of life for this disease.
Key points
- •
Cystic fibrosis transmembrane conductance regulator (CFTR) mutations can be classified into defects that lead to reduced quantity or reduced function of CFTR protein, impairing critical salt and fluid homeostasis in multiple organs.
- •
Classification of mutations is a framework for therapeutic approaches to identify compounds that improve CFTR presence at the cell surface (corrector therapy) or augment channel function of the nascent protein (potentiator therapy).
- •
Ivacaftor (Kalydeco), the first approved CFTR potentiator for individuals with class III (gating) mutations, and Arg117H have demonstrated significant and sustained multisystem improvement.
- •
The combination of ivacaftor and lumacaftor (Orkambi) was approved in 2015 for individuals homozygous for the Phe508del mutation. Its long-term clinical impact is not yet known.
- •
Novel systems and disease markers that address and monitor individualized response to therapies are being developed and will serve as important tools to explore current and future CFTR modulators.
Introduction
The science of personalized medicine is taking shape in the cystic fibrosis (CF) community with genotype-directed therapies available for more than half of the CF population. Personalized or precision medicines approach the treatment of disease by accounting for an individual’s variability in genes, environment, and lifestyle.
This momentum in precision medicine launched with the basic understanding of CF as a result of mutations in the cystic fibrosis transmembrane conductance regulator (CFTR) gene. In the decades since CFTR gene identification, knowledge of CFTR mutations and their pathophysiologic consequences has rapidly expanded, leading to the development of small-molecule therapies that target specific CFTR variants that have some level of CFTR protein produced. These small-molecule therapies are defined as CFTR modulators, a novel class of precision medicines directed to improve CFTR function and/or presence at the cell surface level.
In this review, we focus on therapeutic approaches, known as “potentiators” and “correctors” that explore our understanding of specific CFTR variants and aim to augment or repair function of the CFTR protein.
Introduction
The science of personalized medicine is taking shape in the cystic fibrosis (CF) community with genotype-directed therapies available for more than half of the CF population. Personalized or precision medicines approach the treatment of disease by accounting for an individual’s variability in genes, environment, and lifestyle.
This momentum in precision medicine launched with the basic understanding of CF as a result of mutations in the cystic fibrosis transmembrane conductance regulator (CFTR) gene. In the decades since CFTR gene identification, knowledge of CFTR mutations and their pathophysiologic consequences has rapidly expanded, leading to the development of small-molecule therapies that target specific CFTR variants that have some level of CFTR protein produced. These small-molecule therapies are defined as CFTR modulators, a novel class of precision medicines directed to improve CFTR function and/or presence at the cell surface level.
In this review, we focus on therapeutic approaches, known as “potentiators” and “correctors” that explore our understanding of specific CFTR variants and aim to augment or repair function of the CFTR protein.
Approaching cystic fibrosis transmembrane conductance regulator: review of structure and function
CFTR, located in the apical membranes of epithelial cells in multiple exocrine organs, is a chloride and bicarbonate ion channel that regulates salt and fluid homeostasis. The CFTR glycoprotein has multiple membrane-integrated subunits that form 2 membrane-spanning domains (MSDs), 2 intracellular nucleotide-binding domains (NBDs), and a regulatory (R) domain, which acts as a phosphorylation site. MSD1 and MSD2 form the channel pore walls. Opening and closing of the pore is through ATP interactions with cytoplasmic NBD domains, leading to conformational changes of MSD1 and MSD2. Gating and conductance is regulated through R domain phosphorylation with protein kinase A (PKA).
The intricate regions of CFTR require processing and maturation to allow precise folding. CFTR structure must satisfy rigorous quality standards to be exported from the endoplasmic reticulum and subsequently transported to the cell surface. CFTR that fails to meet these standards is destined to endoplasmic reticulum–associated protein degradation (ERAD). Such a complex quality-control system operates at the detriment of efficiency, decreasing export production of even wild-type CFTR to 33% of similar family cell transporters.
CF is a result of mutations that alter CFTR in these domains or the way these domains interact with each other. Ultimately, these defects affect the function or quantity of the channel at the cell surface. With nearly 2000 disease-causing mutations identified in CFTR, variants have historically been categorized in 5 or 6 functional classifications ( Fig. 1 ). Class I mutations lead to a lack of protein synthesis, such as those with a premature termination codon present. Class II mutations are unable to mature, leading to early degradation through the mechanism of ERAD and resulting in CFTR rarely reaching the cell surface. Class III mutations are considered gating defects with abnormal regulation that make the pore nonfunctioning. Class IV defects have inefficient CFTR function with defective chloride conductance. Class V and VI mutations are those that lead to decreased quantity of CFTR at the cell surface as a result of promoter or splicing defects (V) or increased turnover from the cell surface (VI).
These categories of molecular mechanisms provide a useful framework to consider personalized therapeutic approaches ( Table 1 ), but present an oversimplification in that a single variant can disrupt multiple functional classes. Phe508del, the most common CFTR allele, is a prime example of this complexity. Deletion of phenylalanine leads to instability and abnormal folding of the NBD1 region and increased ERAD, categorizing it classically as a class II mutation. A small portion of synthesized protein, however, is able to traffic to the cell membrane, but has increased degradation like a class VI mutation. Additionally, Phe508del alters protein stability of NBD1 and MSD2, 2 important structural domains needed to open the channel, leading to defective gating as in a class III mutation. Personalized therapeutic approaches need to consider the potential diversity of effects a single mutation may determine.
Class | Impact on CFTR Protein | Common Mutation (Legacy Name) | Approved Therapy (Dose) | Therapies in Development |
---|---|---|---|---|
I | Lack of protein synthesis (reduced quantity) | Trp1282X (W1282X) | — | Ataluren (Phase 3) |
II | Abnormal processing with misfolding keeping it from reaching cell surface (reduced quantity) | Phe508del (deltaF508) | Lumacaftor/Ivacaftor (400 mg every 12 h/250 mg every 12 h) | VX-661/Ivacaftor (Phase 3) |
III | Reaches cell surface, but gating defect impairs function (reduced function) | Gly551Asp (G551D) | Ivacaftor (6 y+: 150 mg every 12 h; 2–5 y <14 kg: 50 mg every 12 h; 2–5 y ≥14 kg: 75 mg every 12 h) | VX-661/Ivacaftor (Phase 3) |
IV | Reaches cell surface, but conductance defect leads to faulty opening (reduced function) | Arg117His (R117H) | Ivacaftor (6 y+: 150 mg every 12 h; 2–5 y <14 kg: 50 mg every 12 h; 2–5 y ≥14 kg: 75 mg every 12 h) | VX-661/Ivacaftor (Phase 3) |
V | Created in insufficient quantities (reduced quantity) | 3849 + 10 kb C→G | — | — |
VI | Rapid turnover (reduced quantity) | Cys1400X (4326delTC) | — | — |
High-throughput screening to identify cystic fibrosis transmembrane conductance regulator modulators
Identifying modulators has been bolstered by the advent of high-throughput screening (HTS). HTS uses robotic drug screening of more than a million molecules in cell-based fluorescence assays of membrane potential or halide efflux to identify candidate compounds that restore CFTR activity in vitro. HTS defined 2 approaches to identify modulators, termed potentiators and correctors. Potentiators are candidate agents that demonstrate improved CFTR chloride conductance in the absence of preincubation time for synthesis of new protein. It is presumed that potentiators augment ATP activation of the pore of nascent CFTR transcripts that have successfully trafficked to the cell surface. Candidate agents, termed correctors, focus on improved processing and chaperoning of CFTR. The corrector therapies rescue CFTR activity only after incubation time with the agent to promote stability and cell-surface trafficking of CFTR protein.
HTS is an automated process that screens blindly to identify hundreds of chemical compounds with the outcome of CFTR-mediated chloride secretion ( Fig. 2 ). These compounds are deemed “hits” but require multiple cycles of medicinal chemistry, including validation in a second assay, such as human bronchial epithelial cells. Among these “validated hits,” lead compounds are identified with a chemical scaffold that optimizes potency and selectivity. Ultimately, development candidates are refined in animal studies to develop drugs that are tested for safety and efficacy in clinical trials.
Augmenting cystic fibrosis transmembrane conductance regulator activity: the potentiator approach
CFTR variants that benefit from the potentiator approach have absent or limited pore function, characteristic of functional class III or IV mutations. Potentiators can function through enhancing the open configuration or improving the gating of the CFTR channel. Gly551Asp-CFTR is the most prevalent gating mutation, present in 4% to 5% of individuals with CF. This variant is thought to interfere with the NBD1 and its ability to interface with NBD2, a function crucial for pore opening.
Production of ivacaftor (Kalydeco) is proof-of-concept of CFTR modulation. Hundreds of thousands of chemical compounds were screened through HTS to identify CFTR potentiator candidates and of these, ivacaftor (formerly known as VX-770) was selected for further development because of its favorable pharmacokinetic profile and its ability to augment various CFTR mutants. Ivacaftor was rigorously tested in cell models and demonstrated an increase in chloride secretion for Gly551Asp/Phe508del-CFTR by approximately 10-fold, nearly 50% of activity seen with wild-type CFTR. This improvement was also reflected in improved mucociliary beating and increased apical fluid level. With these encouraging in vitro data, initial human trials with ivacaftor were started.
The safety profile of ivacaftor was evaluated in a phase 2 randomized placebo-controlled trial of 39 adults with at least 1 copy of Gly551Asp-CFTR. In addition to a reassuring safety profile with similar adverse event rates in placebo and treated groups, subjects demonstrated a significant improvement in lung function, the most responsive at a dose of 150 mg/d. Additionally, markers of CFTR activity, including nasal potential difference and sweat chloride concentration, were shown to have significant improvements. With this evidence, ivacaftor was evaluated in a phase 3 trial, known as STRIVE, that enrolled 161 subjects, 12 years and older, with at least 1 copy of the Gly551Asp mutation. Individuals were randomized in a double-blind trial to receive 150 mg ivacaftor or placebo twice daily for 48 weeks. Within 2 weeks of therapy, the ivacaftor group demonstrated a 10.6% improvement in the primary end point: change from baseline of percent of predicted forced expiratory volume in 1 second (FEV 1 ). This effect was sustained through the 48 weeks of the study. Important secondary outcomes included a 55% reduction in pulmonary exacerbations through week 48, an increase in 2.7 kg of weight through week 48, and improved quality of life in respiratory domains, as determined by validated questionnaire. Biomarkers as in the phase 2 trial also demonstrated improvement in sweat chloride with ivacaftor, and the adverse event rates were similar in both groups. In fact, the serious adverse event rate was lower in the treated group compared with placebo (24% vs 42%) primarily driven by a decreased number of pulmonary exacerbations in the treated group. Similar magnitude of outcomes were confirmed and validated in a second phase 3 trial, known as ENVISION, which enrolled children ages 6 to 11 years with Gly551Asp-CFTR.
The Food and Drug Administration (FDA) approved ivacaftor in 2012 for those 6 years and older with 1 copy of Gly551Asp-CFTR. Commonly reported side effects and monitoring recommendations on the prescribing label are summarized in Box 1 . An open-label trial, known as PERSIST, monitored those on STRIVE and ENVISION, noting sustained benefits of 9.4% and 10.3% of absolute change in FEV 1 and weight gain at both 96 and 144 weeks. Pulmonary exacerbation rate decreased in adolescents and adults, but not in the younger cohort, who had a much lower baseline frequency of pulmonary exacerbations.
Ivacaftor | |
Absorption | Take tablet with fat-containing food. |
Elevated liver transaminases | Monitor alanine and aspartate transaminases before initiation, every 3 mo in the first year, and annually thereafter. |
Cataracts | Eye examination before initiation and follow-up for patients younger than 18 y. |
Drug interactions (St John wort, rifampin) | Decreases exposure to ivacaftor and coadministration is not recommended. |
Drug interactions (ketoconazole, fluconazole) | Increases exposure to ivacaftor. Dose adjustment of is needed. |
Drug interactions (Seville oranges, grapefruit) | Increases exposure to ivacaftor. Avoid food containing grapefruit or Seville oranges. |
Lumacaftor-Ivacaftor | |
Absorption | Take tablet with fat-containing food. |
Elevated liver transaminases | Monitor alanine and aspartate transaminases before initiation, every 3 mo in the first year, and annually thereafter. |
Liver disease | Childs-Pugh score classification and dose adjustment may be necessary. Caution in patients with advanced liver disease. |
Cataracts | Eye examination before initiation and follow-up for patients younger than 18 y. |
Chest discomfort, dyspnea, breathing difficulties | Additional monitoring recommended for patients with forced expiratory volume in 1 s % predicted <40 during therapy initiation. |
Drug interactions (hormonal contraceptives) | Decreased efficacy of hormonal contraception. Alternative methods of contraception and anticipation of menstrual-related adverse reactions. |
Drug interactions (benzodiazepines, immunosuppressants, digoxin, corticosteroids, antidepressants, proton pump inhibitors) | Decreases efficacy of these medications. Dose adjustment, level monitoring if applicable, or alternative agent recommended. Coadministration not recommended. |
Drug interactions (St John wort, rifampin, phenytoin) | Decreases exposure to lumacaftor-ivacaftor and coadministration is not recommended. |
Drug interactions (ketoconazole, itraconazole, voriconazole, clarithromycin) | Increases exposure to lumacaftor-ivacaftor. Dose adjustment may be needed when initiating therapy. |
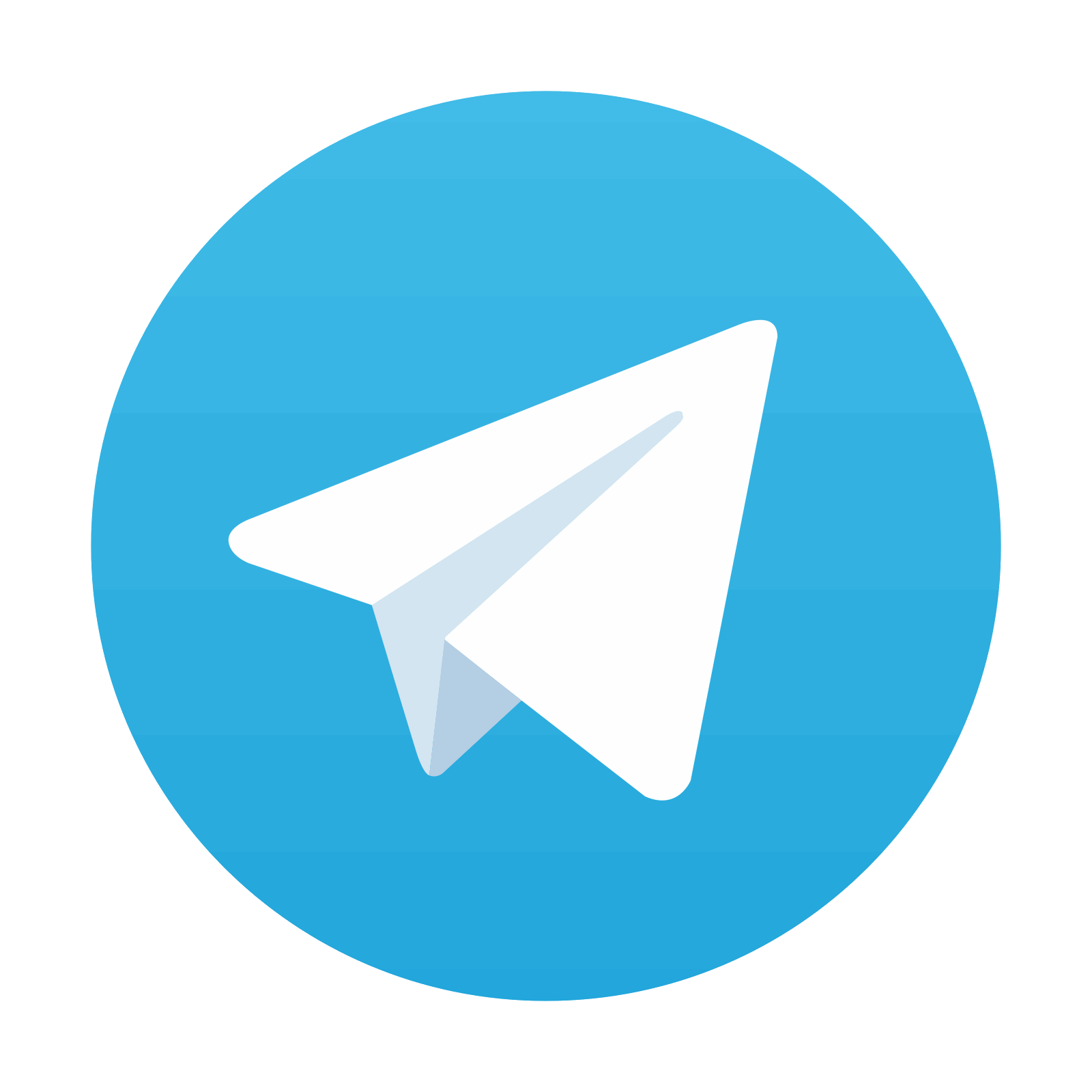
Stay updated, free articles. Join our Telegram channel

Full access? Get Clinical Tree
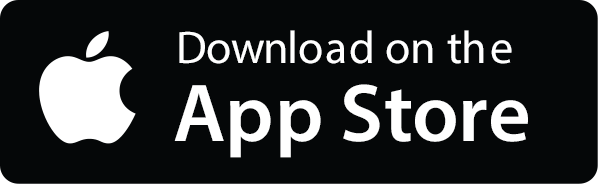
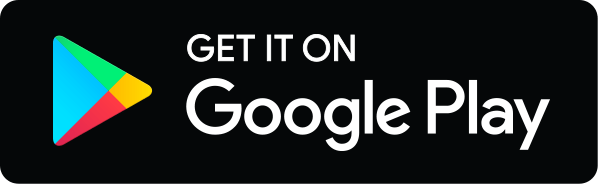