Neurosurgery of the Newborn
Joseph R. Madsen
David M. Frim
Anne R. Hansen
Treatment of structural and functional abnormalities of the neonatal central nervous system (CNS) provides unique challenges to both the neurosurgeon and neonatologist caring for the infant. Considerations of several interrelated pathophysiologic processes can provide an understanding of the nature of the abnormality and goal of treatment. This understanding forms the basis for a successful interdisciplinary team approach to the management of these infants.
THE PATHOPHYSIOLOGY OF NEONATAL NEUROSURGERY
Almost all neurosurgical interventions in the neonate can be discussed in terms of four categories of technical intervention: (a) drainage or diversion of fluids, (b) closure of openings (including neural tube defects), (c) removal of tissue (including neoplasms and anomalous masses), and (d) opening of fusions (such as craniosynostoses). In the management of many newborn patients, two or more of these inventions may be necessary. Some neonatal nervous system problems, such as vascular disorders, do not yet have a workable neurosurgical intervention.
When applying these inventions to neonatal care, their use must be guided by pathophysiologic mechanisms unique to the neonate, such as (a) the biomechanics of neonatal brain tissue and a distensible skull, (b) recognized and unrecognized congenital anomalies (microscopic and macroscopic), and (c) the plasticity of neonatal CNS tissue and its effects on response to injury. Recent advances in antenatal diagnosis allow unprecedented opportunity to manage these processes even before birth. Frequently, solutions to a neonatal neurosurgical problem must address combinations, or complex interactions, of these pathophysiologic mechanisms.
The biomechanic nature of neonatal brain tissue and a distensible skull accounts for one of the best-known cardinal signs of neurosurgical difficulty in the neonate, namely abnormal head growth. The prodigious ability of the skull to grow and sutures to widen can allow some of the most severe cases of hydrocephalus to result in relatively mild pressure effects, even with an obvious need for cerebrospinal fluid (CSF) shunting for long-term management. These same considerations in the type and nature of response of the tissues to abnormal fluid build-up can result in dramatic structural difficulties once the pressure is relieved. The certainty that the brain and skull biomechanics will change over time adds particular challenge to management of these problems.
Obvious congenital anomalies, especially those involving open neural-tube defects and exposed CNS tissue, may require urgent neurosurgical intervention. A complicating aspect is the potential occurrence of concurrent microscopic abnormalities, such as widespread synaptic miswiring. In many cases, neurologic deficits may be present in a patient with macroscopic structural abnormalities, but major neurologic disability may come from less obvious problems with tissue development, currently not treatable by neurosurgical means.
The details of the plasticity and vulnerability of the neonatal CNS is discussed in Chapter 50. It is well known that the ability to recover function after CNS injury is age dependent and seems to be better in younger individuals. Interaction between the age-dependent variables of CNS plasticity, recoverability, and vulnerability make the task of estimating prognosis after an injury or neurosurgical intervention extremely difficult.
Antenatal diagnosis has changed many aspects of neurosurgical conditions. Obstetric management may be altered, for example, site and mode of delivery in the setting of a known neural-tube defect. In addition, the psychologic issues around the time of neurosurgical therapy for the newborn are altered, as the child’s family now has the opportunity to meet the neurosurgeon prenatally and fully discuss and decide upon any contemplated care
plan before birth. Antenatal surgery for some neurosurgical conditions (such as neural-tube defects and hydrocephalus) may one day be routine, but at this time these techniques are still available only in experimental, randomized trials.
plan before birth. Antenatal surgery for some neurosurgical conditions (such as neural-tube defects and hydrocephalus) may one day be routine, but at this time these techniques are still available only in experimental, randomized trials.
FLUID COLLECTIONS AND THEIR MANAGEMENT
Disorders of Cerebrospinal Fluid Accumulation
The most common neurosurgical consultation for newborn patients is to evaluate and treat enlargement of the ventricular system. The clinical signs of progressive enlargement of the ventricular system include excessive increase in the head circumference (HC), fullness in the anterior fontanelle (especially when the patient is upright, the venous and fontanelle pressures should be low), episodic apnea and bradycardia, general lethargy, and abnormalities of ocular movement, especially restricted upgaze. Many of these findings are nonspecific and can they be seen with hydrocephalus of any etiology. At a practical level, the most important distinction is between progressive and static abnormalities in ventricular volume. This is relatively easy to determine using serial cranial ultrasound examinations (Fig. 51-1). The recent addition of resistive index (RI) measurements adds a physiologic dimension to the anatomic images gathered by ultrasonography (1). In our experience, the presence of dramatic changes in the Doppler-measured flow signals in the anterior cerebral artery with gentle, brief compression of the anterior fontanelle correlates extremely well with the intracranial pressure by measurement and the probability of eventual need for a ventriculoperitoneal (VP) shunt (1).
The vast majority of hydrocephalus cases seen in neonates are associated with, and traditionally thought to result from, abnormal resorption of CSF. However, Egnor (2) has persuasively argued the contrasting view that abnormalities in the pulsatile dynamics of the CNS may play a role in the etiology of communicating hydrocephalus. In this view, the purpose of CSF and the ventricular system is to absorb the kinetic energy of the arterial pulsations and transfer this energy out of the skull, primarily via the venous system. After intraventricular hemorrhage (Fig. 51-1), traditional thinking is that partial occlusion of the normal resorptive pathways of CSF through the arachnoid villi causes hydrocephalus. Pulsatile theories suggest that breakdown of the resonance of pulsations could also play a role. Thus, relative blockade of bulk CSF absorption or blockade of absorption of the pulsations could raise the steady state to a relatively higher pressure. In the neonate with open sutures, this results in a larger intracranial volume. It also follows that there would be some cases of mild increase in resistance to absorption of fluid (or pulsations) that would not require specific treatment, whereas higher grades of failure to absorb fluid would cause substantial increases in pressure, leading to neurologic symptoms if not treated.
Placement of a shunt in borderline cases may result in atrophy of physiologic resorption systems and, thereby, cause more permanent obstruction to resorption of CSF. This is conceptualized as atrophy of arachnoid granulations or failure to develop normal resorptive pathways, but the detailed pathology is not well known. A related paradox of shunt physiology, particularly applicable to neonates, is that, with currently available hardware, it is impossible to distinguish between a well-functioning shunt and nonfunctioning shunt in a patient who is no longer shunt dependent. Technologies that accurately measure the flow through the shunt, or through some physiologic pathway such as the aqueduct of Sylvius, or a ratio of these two numbers, could be a reliable descriptor of shunt dependence. It is theoretically possible that such measurements could be made from dynamic magnetic resonance imaging (MRI) studies. So far, such a technique has not been applied reliably.
One of the problems with assessing the growth of ventricular size in the neonate is the possibility that the enlargement of the ventricles may at least partially reflect loss of tissue in the brain, rather than increase in pressure. This can become a very complicated situation, as prolonged hydrocephalus itself certainly can cause loss of tissue bulk (3), and the value of shunt placement may bemore difficult to determine when the tissue loss is not obviously the result of high pressure. The specific loss of myelin or white matter, called periventricular leukomalacia, is discussed in Chapter 50. The relationship between periventricular leukomalacia and hydrocephalus is of particular concern in neurosurgical decision making. When a preterm infant, especially one who has sustained an intracranial hemorrhage, shows lateral ventricular enlargement, it is difficult to determine to what extent this is a result of impaired CSF flow or absorption versus diffuse white matter damage or inadequate density of axons. In general, the absence of macrocephaly is an indicator of the atrophy. More specific strategies to rescue white matter in such circumstances probably will evolve and may diminish the call for shunts in some of these patients (4).
Nevertheless, assessing for macrocephaly is complicated by the changing growth rate of the normal neonatal head (5). In general, an HC growth of less than 1 cm/week is acceptable, and an HC growth of more than 1.5 cm/week is considered excessive. Head growth must be interpreted in the context of overall somatic growth measured by weight and length. Babies can have preferential brain catch-up growth, but it is suspicious if the rate of HC increases at the high end of normal while the weight and length remain relatively unchanged. Because major intervention decisions are based on the intrinsically imprecise measurement of HC, any effort to improve its precision is worthwhile. Minimizing the number of care providers obtaining the measurement and overtly standardizing the measurement site and technique improves accuracy. The effect of interpreter variability can be dampened by comparing the HC to the previous day and also to 1 week earlier to calculate average daily growth by dividing by seven. Using a correct and accurate growth curve is also important (6,7).
Fluid in the subarachnoid space outside the brain, sometimes termed “benign external hydrocephalus,” is probably a normal variant. The increased collection of extraaxial fluid is generally in the frontal regions. The diagnosis of subdural hygroma frequently has been applied to these cases; this diagnosis must be applied with great caution if it is intended to imply a pathologic collection benefiting from drainage (8).
Treatment of Neonatal Hydrocephalus
Due to the disadvantages and complications of VP shunting, the decision to place a shunt must be made only after all nonsurgical options have failed. The fact that the CSF resorption may change with time, and indeed may improve, provides a rationale for conservatism. As a patient grows and the dynamic relationship between CSF production and resorption changes, the need and indications for diversion of CSF may change over the child’s lifetime.
Children born with hydrocephalus due to nonhemorrhagic causes, such as aqueductal stenosis, posterior fossa cysts, holoprosencephaly, or hydranencephaly, can be shunted within 1 to 2 days of birth, pending evaluation of general medical status. Although affected infants usually are born at or near term, a presurgical weight of approximately 1,500 to 2,000 grams is adequate size to support peritoneal drainage of ventricular CSF (9). There are ethic issues that should be raised before placing a VP shunt in a child suffering from hydrocephalus with significantly reduced brain function from either an in utero event or developmental anomaly. Frank discussion with the child’s parents regarding prognosis is critical. Shunting for affected children without other life-threatening anomalies is certainly reasonable for the purposes of controlling head size in the growing infant. With maximal interventive therapy, most of these children are expected to live beyond a few weeks, and such day-to-day issues as whether the child’s head will fit in an infant car seat need to be considered. Shunting at birth is a relatively low-risk method of preventing massive head enlargement and allowing other factors aside from congenital hydrocephalus to determine the child’s outcome (10).
Nearly all acquired hydrocephalus in the premature infant is posthemorrhagic (Fig. 51-1). These infants have usually not achieved adequate weight for placement of permanent shunts, and the final determination of lifelong hydrocephalus has not been made. In this situation, medical management (11) is instituted to temporize until surgical management is both clearly indicated and feasible. The mainstays of medical management are serial lumbar punctures and diuretic therapy, though use of the latter is becoming increasingly controversial (12,13). The use of these maneuvers is discussed in Chapter 50. An additional approach that has been tried for posthemorrhagic hydrocephalus (PHH) is the introduction of fibrinolytic therapy directly into the ventricular system. Enzymes such as streptokinase, urokinase, and tissue plasminogen activator all have been proposed and tested with variable success. Some centers have suggested that the risk of VP shunts can be reduced using this technique (14). Others have been unable to show a decrease in the need for VP shunting with this maneuver (15,16). It is possible that the trials which found no helpful effect from fibrinolytic therapy had less favorable results because of patient selection, with a trend toward enrolling the most severe and potentially intractable cases. Infants enrolled in a recent, small trial combining fibrinolytic therapy with continuous drainage and irrigation had a reduced rate of shunt requirement, disability, and mortality compared to historic controls (17). However, larger trials are needed to further delineate the risks and benefits of this high-risk, labor-intensive treatment. At this point, the underlying difficulty with
fibrinolytic therapy is the lack of overlap of the safety and efficacy windows. This therapy cannot currently be considered standard therapy.
fibrinolytic therapy is the lack of overlap of the safety and efficacy windows. This therapy cannot currently be considered standard therapy.
Another intervention that has been suggested is the use of ventriculoscopic removal of clot, however, based on little published experience, this has not been demonstrated to avert the need for VP shunts. A new technique for the treatment of hydrocephalus caused by obstruction of the aqueduct of Sylvius employs the fiberoptic ventriculoscope to place a fenestration in the floor of the third ventricle to allow the escape of CSF into the subarachnoid space. Indeed, even in such cases of aqueductal stenosis, where eventual therapy using the ventriculoscope works well in older children, most surgeons currently find that the defect in the floor of the third ventricle in the neonate tends to close at a very high rate (18).
The risk of PHH correlates with the severity of intraventricular hemorrhage. Although other grading systems have been described, we use the Papile four-level system of intracranial hemorrhage (19): grade I, subependymal hemorrhage only (no increased risk of PHH); grade II, intraventricular hemorrhage without ventriculomegaly (low risk of progressive PHH); grade III, intraventricular hemorrhage with ventriculomegaly (moderate risk of progressive PHH); and grade IV, intraventricular hemorrhage with intraparenchymal extension (generally at high risk of need for VP shunting, depending on degree of intraventricular component).
There are many published management protocols for children with PHH (9-11,19,20). Evaluation usually includes neurosurgical consultation, ultrasound of the head to assess the size of the ventricles, and lumbar puncture to measure CSF pressure. During a head ultrasound, it can be helpful to measure the RI defined as

Concern regarding hemodynamic compromise is raised either by an RI above the normal value of 0.5 to 0.7 or a rise in RI with gentle compression of the anterior fontanelle.
Interventions are tailored to maintain the lumbar or ventricular CSF pressure at approximately 5 to 8 cm of water while evaluating the need for permanent shunt placement. The medical approaches to this problem have been described (11,21). The goal of medical management is to decrease CSF volume either by decreasing CSF production via diuretic therapy or removing CSF in bulk.
Mechanical CSF drainage by serial lumbar puncture or direct ventricular access is the mainstay of treatment of elevated intracranial pressure while awaiting the appropriate time for VP shunt insertion. The frequency of CSF drainage procedures is determined by measuring opening CSF pressures during lumbar taps with a goal of less than 10 cm of water (10). For example, if an every third day tapping schedule allows the CSF pressure to rise to greater than 10 cm of water, the frequency of CSF withdrawal should be increased to every other day. Fontanelle tenseness, HC, and ultrasonographic RIs also are acceptable indications of intracranial pressure (1). Approximately 10 to 15 mL/kg should be removed to leave the closing CSF pressure as low as is feasible, usually less than 3 cm of water. This approach is based on cerebral perfusion management rather than measures of HC or ultrasonographic documentation of ventricular size.
HC, ultrasonographic criteria, and other physical examination criteria provide additional data to guide CSF drainage therapy. Data interpretation and management decisions should be based on the overall goal of maximizing the potential for neuronal development in an environment of low intracranial pressure and minimal periventricular axonal stretch.
Although serial lumbar punctures may be useful in many cases, they often fail because of abnormalities within the spinal system that block CSF flow, particularly common after intraventricular hemorrhage. These have been documented with spinal ultrasound (22).
Acetazolamide and furosemide reduce CSF production. Though theoretically their effects are synergistic, this combination of diuretic therapy is now used with more caution because of concerning studies that found possibly worse neurologic outcome secondary to the use of these medications together (12,13). There are extremely limited data regarding acetazolamide alone. In a sample size of only five, short-term improvement was appreciated in the three patients who did not require the medication to be discontinued secondary to metabolic acidosis (23). Thus, its safety and efficacy as a single agent are essentially unknown. In certain circumstances when serial lumbar punctures are either not possible or not feasible, acetazolamide therapy may play a role. Furosemide should be added with great caution as it adds potential risk with no documented benefit.
Medical treatment with CSF drainage and/or diuretic therapy is continued to determine the natural history of the condition and allow for adequate growth of the infant to permit safe placement of a VP shunt, should that be necessary. During this period, the requirement for CSF drainage will accelerate, remain stable, or decrease. This is ascertained by continuing to measure CSF pressures. If there is no increase in pressure between taps, then it is likely that CSF is being reabsorbed internally. In this situation, the ultrasound image of the ventricles, as well as the HC measurements, is helpful in confirming that the reabsorptive surface is functioning adequately.
Implantation of devices that allow easier intermittent (Fig. 51-2), or continuous CSF drainage, should be considered when the need for drainage accelerates to the point when the neonatal intensivist or neurosurgeon is performing lumbar punctures daily or with such frequency as to endanger an infant’s medical stability. Additionally, serial lumbar puncture can cause arachnoid scarring and lead to an inability to obtain adequate CSF drainage from a lumbar approach. In cases of noncommunicating PHH, CSF removal from the lumbar region is not an option. In all of these cases, direct approach to the ventricles either by percutaneous tap or implanted access device is required.
![]() Figure 51-2 Ventricular access device with 30-mm catheter. Implantation allows easy percutaneous access to ventricular fluid. |
Though percutaneous ventricular tap in a neonate is a relatively simple procedure, it should only be performed by experienced practitioners. A 20-gauge intravenous catheter is introduced perpendicularly to a plane tangential to the scalp at the lateral edge of the anterior fontanelle. After penetrating the dura with the inner needle, the soft catheter is advanced over the needle into the ventricle. Drainage then proceeds as with a lumbar puncture.
Ventriculosubgaleal shunts are increasingly being used instead of ventricular access devices to temporize prior to placement of a VP shunt. The device, as well as the surgical implantation technique, is very similar to that of the ventricular access device (Fig. 51-3). The only differences are that the blind end of the catheter is cut off, and the open end is allowed to drain into a subgaleal (essentially subcutaneous) pocket in the infant’s head. The major advantage is that the subgaleal pocket may allow some resorption of CSF and certainly can absorb fluid pulsations. Infants with ventriculosubgaleal shunts typically require far less frequent taps, and sometimes no taps at all (24). If necessary, the reservoir or subcutaneous fluid collection can be tapped, just like a ventricular access device. The cosmetic issue of a visible subcutaneous fluid-filled space can be concerning to parents and care providers who are unfamiliar with this technique, but all can be reassured that this will resolve after placement of a VP shunt, which follows in the vast majority of cases. The indications for tapping ventriculosubgaleal shunt devices are the same as for ventricular access devices, and the follow-up assessment tests are identical, including HC, sonographic assessment of ventricular size, and RI.
Though largely replaced by the subgaleal shunt at many institutions, ventricular access devices (Fig. 51-2) are still occasionally placed. These devices allow direct percutaneous access to the ventricle via the subcutaneous reservoir. The protocol for CSF drainage is identical to that for lumber puncture. Infection of these devices is rare despite frequent percutaneous access. Ventricular access devices can be set up for continuous drainage by placement of a Huber needle into the reservoir, which is connected to a ventricular drainage bag. This maneuver is performed when taps are required more than once a day. During periods of continuous drainage, infants should be treated with prophylactic intravenous antibiotics.
After long intervals between CSF removal, if the pressure is unchanged and the ventricles are not enlarging, it is reasonable to continue to follow ventricular size by ultrasound alone. At that point, any diuretic treatment is weaned and then discontinued if tolerated. If the need for CSF drainage remains unchanged or accelerates as the patient grows, then the diagnosis of uncompensated hydrocephalus is made and a VP shunt is inserted. A patient weight of 1,750 grams is usually sufficient to permit implantation of a permanent VP shunt with minimal complications.
The contraindications to implantation of a permanent VP shunt are similar to those found in older children: evidence of CSF infection, significantly elevated CSF protein, presence of a high CSF red blood cell count which may mechanically obstruct the shunt, peritoneal inflammation, or infection such as necrotizing enterocolitis. These problems necessitate a delay in implantation until the child is healthy. CSF glucose parameters are sometimes quite low (less than 20 mg/dL) in neonates with PHH in the absence of infection. The significance of this finding is unclear; however, there is not an increase in shunt infection in these patients. Similarly, patients with intraventricular hemorrhage, whether adult or neonatal, can manifest fever perhaps from the presence of blood in the CSF. The exact cause of this increased temperature in the neonate is unclear; without positive bacteriologic data, it is unlikely to be infection. Therefore, in the absence of positive culture data, shunt placement should not be delayed.
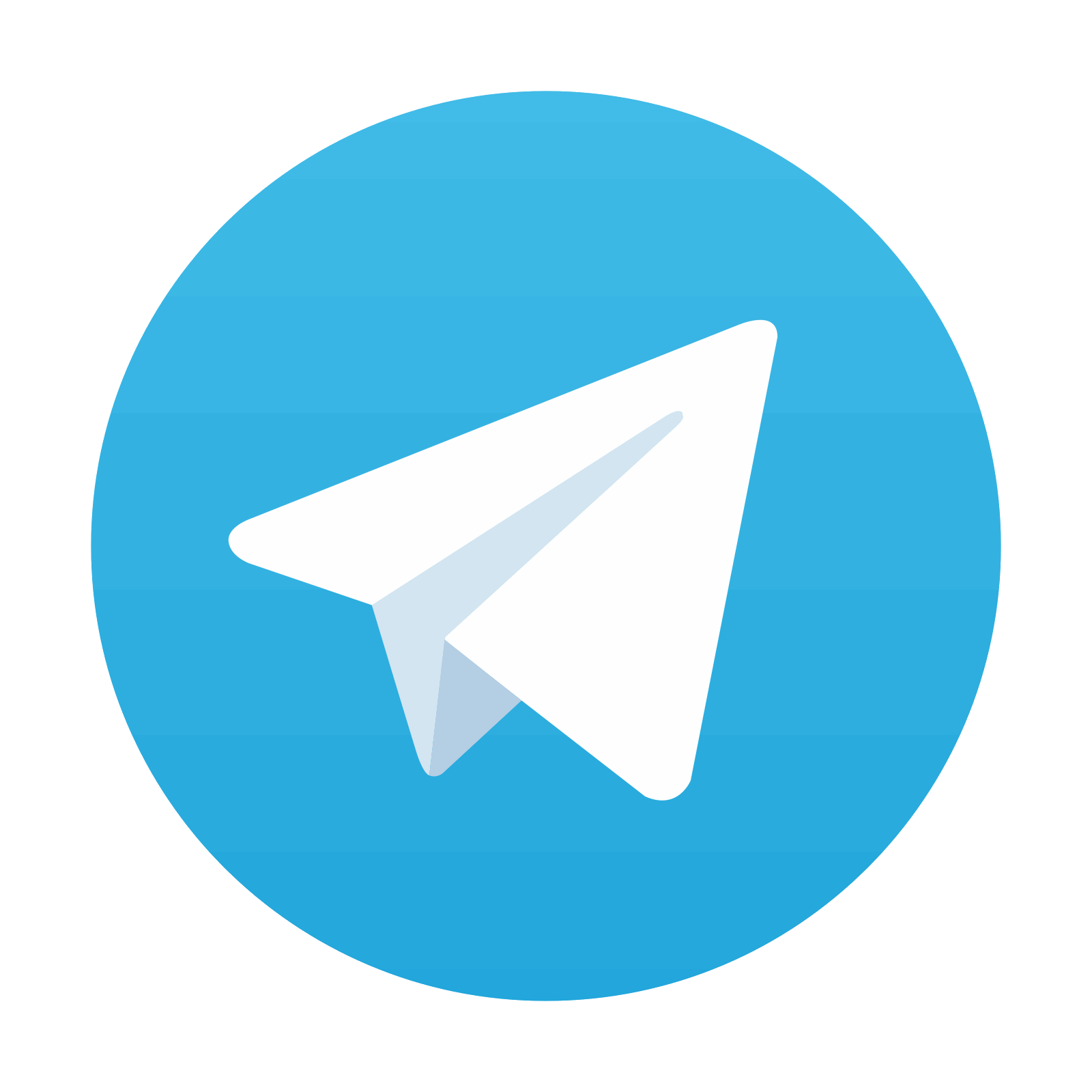
Stay updated, free articles. Join our Telegram channel

Full access? Get Clinical Tree
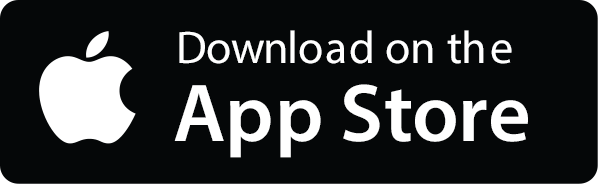
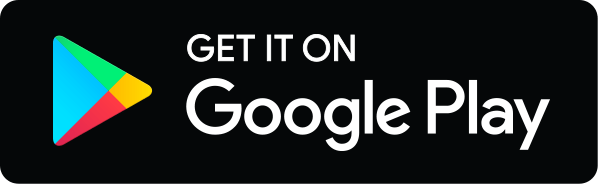