Clinical
Electrodiagnostic
Facial bulbar weak
Respiratory distress
Other
Contractures
NCS
Rep stim/SFEMG
EMG
Anterior horn cell
Spinal muscular atrophy
Common
Possible
Bell shaped chest
Uncommon
Low CMAP
Normal
Neurogenic
Riboflavin transporter deficiency
Common (later)
Late finding
SNH loss early sign
No
May be normal
Normal
Neurogenic
SMARD
Common
Typical
No
May be normal
Normal
Neurogenic
Amyoplasia congenita
No
No
Facial hemangioma
Yes
Low CMAP
Normal
Neurogenic
Peripheral nerve
Charcot-Marie-Tooth
No
No
Areflexic
Possible
Low SNAP CMAP
N/a
Neurogenic
Guillain-Barré syndrome
Possible
Possible
Areflexic
Uncommon
Low SNAP CMAP
N/a
Normal or neurogenic
Neuromuscular junction
Congenital myasthenia
Common
May have apneas
Ptosis
Possible
May be normal
Abnormal
Normal
Transient myasthenia gravis
Common
May have apneas
Ptosis
Uncommon
May be normal
Abnormal
Normal
Infantile botulism
Typical
Typical
Constipation, ptosis
No
Low CMAP possible
Abnormal
Normal
Muscle
Congenital myopathies
Common
Possible
CK normal
Possible
Normal
Normal
Myopathic
Congenital muscular dystrophies
Dystroglycanopathy
Common
Possible
CK elevated, brain, eye findings
Possible
Normal
Normal
Myopathic
Infantile FSH
Typical
Uncommon
CK normal or elevated
Possible
Normal
Normal
Myopathic
Congenital myotonic dystrophy
Common
Possible
CK normal
Possible
Normal
Normal
Myopathic
Mitochondrial disease
Possible
Possible
Lactate, alanine high
Possible
Normal
Normal
Myopathic
Peroxisomal disease
Possible
Uncommon
Possible seizures, renal or hepatic cysts
Uncommon
Normal
Normal
Myopathic
Pompe disease
Common
Possible
Cardiomegaly, CK elevated
Uncommon
Normal
Normal
Myopathic
Infantile botulism does not present at birth, but has been diagnosed in infants as young as a few days old [37, 38]. More commonly, infants will present between 1–6 months old. This age group is particularly vulnerable since the immature gut lacks the protective bacterial flora and bile acids which inhibit Clostridum growth [39]. Infants will typically present with constipation followed by progressive feeding difficulty (due to bulbar weakness), ophthalmoplegia including sluggish pupillary responses, descending paralysis, and apnea. RNS is also helpful at diagnosing this condition particularly higher frequency (20–50 Hz) RNS which can demonstrate an incremental CMAP due to the presynaptic nature of this problem. Early diagnosis is important since treatment with human botulism immune globulin can hasten recovery and reduce the overall duration of hospitalization [40].
Congenital myasthenic syndrome typically presents at or shortly after birth, though there are rare reports of patients presenting in adolescence [41, 42]. Typical clinical features include onset at birth or early childhood and fatigable weakness particularly affecting oculobulbar musculature. Classic electrophysiologic findings include abnormal decrement on RNS and/or increased jitter on stimulated single fiber EMG testing (SFEMG) [43]; the latter is covered in Chap. 10. Some infants with congenital myasthenic syndrome due to choline acetyltransferase (CHAT) mutations can present with sudden episodes of apnea that can be triggered by infection [44]. Care must be taken to differentiate congenital myasthenic syndrome from autoimmune myasthenia gravis in young children so as to avoid the inappropriate use of immunosuppressant therapies and the withholding of medications such as 3,4 di-aminopyridine, salbutamol and/or fluoxetine that may show benefit in some cases [45]. Gene panels are becoming more widely available for patients who demonstrate clinical and electrophysiological evidence for CMS.
Autoimmune juvenile myasthenia gravis (JMG) can also present with fatigable ptosis, ophthalmoplegia, dysarthria, dysphagia and generalized skeletal muscle weakness. Unlike CMS, this condition is due to an acquired autoimmune cause. RNS and/or SFEMG are important for helping establishing a diagnosis. Whereas antibodies to the nicotinic AChR are found in 80% of adults with myasthenia gravis [46], only 50% of prepubertal and 70% of peripubertal JMG patients have anti-AChR antibodies [47], most likely due to the more frequent occurrence of ocular myasthenia in prepubertal children and generalized myasthenia in peripubertal adolescents. Anti-MuSK antibodies also do not appear to be as common in JMG [48]. Intravenous immunoglobulin (IVIG) or plasma exchange (PLEX) can be used in the acute management of myasthenia gravis. Caution must be taken when initiating corticosteroid therapy in weak patients who are not yet intubated since increased weakness may occur within the first 1–2 weeks of corticosteroid treatment, potentially precipitating a myasthenic crisis [49]. Disorders of neuromuscular transmission are covered in detail in Chap. 21.
Muscle
Primary disorders of muscle are a more common cause of neonatal hypotonia. One series reported that myopathies comprised 20% of all causes of neonatal hypotonia as well as 75% of causes of ‘peripheral’ hypotonia [21]. Muscle disorders can include the following groups of disorders which can be grouped into the following large categories: congenital myopathies, congenital muscular dystrophies, congenital myotonic disorders, and metabolic myopathies. Electrophysiological testing will reveal normal sensory responses as well as normal or reduced CMAP amplitudes and EMG evidence of myopathic units. Muscle disorders are covered in detail in Chap. 22.
Critical Illness Polyneuropathy and Myopathy
Critical illness polyneuropathy (CIP) and critical illness myopathy (CIM) are neuromuscular disorders that develop during the course of a critical illness. This is one of the key features that allow patients with CIP to be differentiated from patients presenting with an acute motor sensory axonal neuropathy (AMSAN), namely the axonal variant of Guillain-Barré syndrome [50]. In CIP, patients do not show a preceding history of sensory or motor deficits. Moreover, they must have an associated critical illness that seems to trigger the underlying neuromuscular disease [51] with onset of weakness in the 1–2 weeks following the critical illness [52].
Critical illness polyneuropathy (CIP) and CIM can be difficult to differentiate on clinical and electrophysiological testing [26]. However there are some distinguishing features (Table 23.2). Since CIP and CIM can co-exist in the same patient, some authors have grouped them together as critical illness polyneuromyopathy (CIPNM) or critical illness myopathy and neuropathy (CRIMYNE) [53].
Table 23.2
Key features differentiating critical illness myopathy and critical illness polyneuropathy
Critical illness myopathy |
Critical illness polyneuropathy | |
---|---|---|
Clinical |
Triggered by critical illness |
Triggered by critical illness |
Limb weakness |
Limb weakness (distal) | |
Onset after PICU admission |
Onset 1–2 weeks after PICU admission | |
Serum CK |
Normal or elevated |
Normal |
NCS |
Decreased CMAP amplitudes |
Decreased CMAP amplitudes |
Preserved SNAP amplitudes |
Decreased SNAP amplitudes | |
EMG |
Myogenic changes (low amplitude motor units with or without fibrillation potentials) |
Neurogenic changes (large amplitude motor units with abundant fibrillation potentials) |
Decreased excitability on direct stimulation | ||
Muscle biopsy Histology |
Loss of ATPase reaction in type I fibers |
Acute & chronic denervation changes (i.e. angular fibers, fiber-type grouping) |
Type II fiber atrophy common | ||
Necrotizing myopathy with vacuolization | ||
Destruction of thick myosin filaments | ||
Ultrastructure |
Selective loss of thick (myosin) filaments |
|
Disorganization of myofibrils | ||
Nerve biopsy |
Fiber loss and primary axonal degeneration |
In adults, CIP and CIM are the most common neuromuscular conditions that develop during an ICU admission and diagnostic criteria have been created to permit them to be distinguished from other diseases as well as, whenever possible, from each other [51]. One center reviewed all patients admitted to ICU over 15 years who had neuromuscular disease confirmed by neurophysiological testing. Of this group, 13% patients had CIP and 41% had CIM [26]. The incidence of CIP/CIM in children is less clear. A prospective study of all children (3 months to 17 years old) admitted to the PICU of a pediatric tertiary care hospital identified generalized muscle weakness in 1.7% (14/830) patients [54]. Children with pre-existing neuromuscular diseases and Guillain-Barré syndrome were excluded from this cohort. Of the remaining children showing weakness who underwent electrophysiological testing, about half (4 out of 7) showed low CMAP amplitudes and most (4 out of 5) had myopathic units evident upon EMG analysis. When one considers the difference in study design of the adult [26] and pediatric [54] studies (including versus excluding patients GBS) the overall incidence of CIPM appears similar in the two groups, accounting for about half of all patients with confirmed neuromuscular disease in this setting.
Many adult and pediatric patients who develop CIPM have undergone a solid organ or bone marrow transplant, suffered a traumatic injury (e.g., head injury, burns) and/or have had one or more episodes of bacterial sepsis [26, 54]. The use of corticosteroids and neuromuscular blocking agents is also common. Although the precise mechanism of CIPM is not known, it is believed to result from a systemic inflammatory response syndrome (SIRS) provoked by a severe systemic infection or trauma [51]. Patients exhibit profound changes in cellular and humoral immune responses altering the microcirculation throughout the body. At the microscopic level, mitochondrial dysfunction occurs with the loss of normal ATPase staining in type 1 fibers of a muscle biopsy [51, 54]. Endothelial damage may result in the disruption of the vasa nervorum with resulting ischemia of axons. In muscles this can cause disruption of the function and structure of the basic contractile unit and, in more severe cases, areas of necrosis and vacuolar changes [51]. This evidence of microstructural damage and mitochondrial dysfunction seen on biopsy is congruent with the high rate of long-term morbidity seen in adult patients who have suffered CIM [55, 56]. Prospective studies of adult ICU patients indicate that CIPM occurs in about 50-70% of patients suffering from systemic inflammatory response syndrome [57, 58]. As such, milder cases of CIPM may even be more prevalent than that reported by Lacomis [26] and Banwell [54]. Phrenic nerve conduction studies and needle EMG of the diaphragm and chest wall muscles have been recommended in adult patients with suspected CIPM [51]; however, these particular studies are not commonly performed in pediatric EMG.
Drugs Exacerbating Underlying Neuromuscular Diseases
Medications or toxins can exacerbate underlying disorders of nerve, muscle and neuromuscular transmission.
Chemotherapy Induced Peripheral Neuropathy
Chemotherapeutic agents such as vincristine have been reported to cause significant toxicity in patients with Charcot-Marie-Tooth disease.
Most individuals will not report symptoms of a vincristine-induced sensorimotor neuropathy until after a minimum cumulative dose of vincristine 5–8 mg has been administered [59]. However patients with Charcot-Marie-Tooth disease will show marked sensitivity to this chemotherapeutic agent with dramatic sensory symptoms or even a Guillain-Barré-like phenotype apparent after a cumulative dose of only 2 mg [59, 60]. On rare occasions CMT has presented in previously asymptomatic patients after receiving vincristine to treat lymphoma [61, 62]. Reports exist of adults with no prior neurological symptoms progressing to quadriplegia and bulbar palsy after vincristine therapy [62]. As such, hereditary neuropathies must be considered among individuals showing extreme sensitivity to this drug.
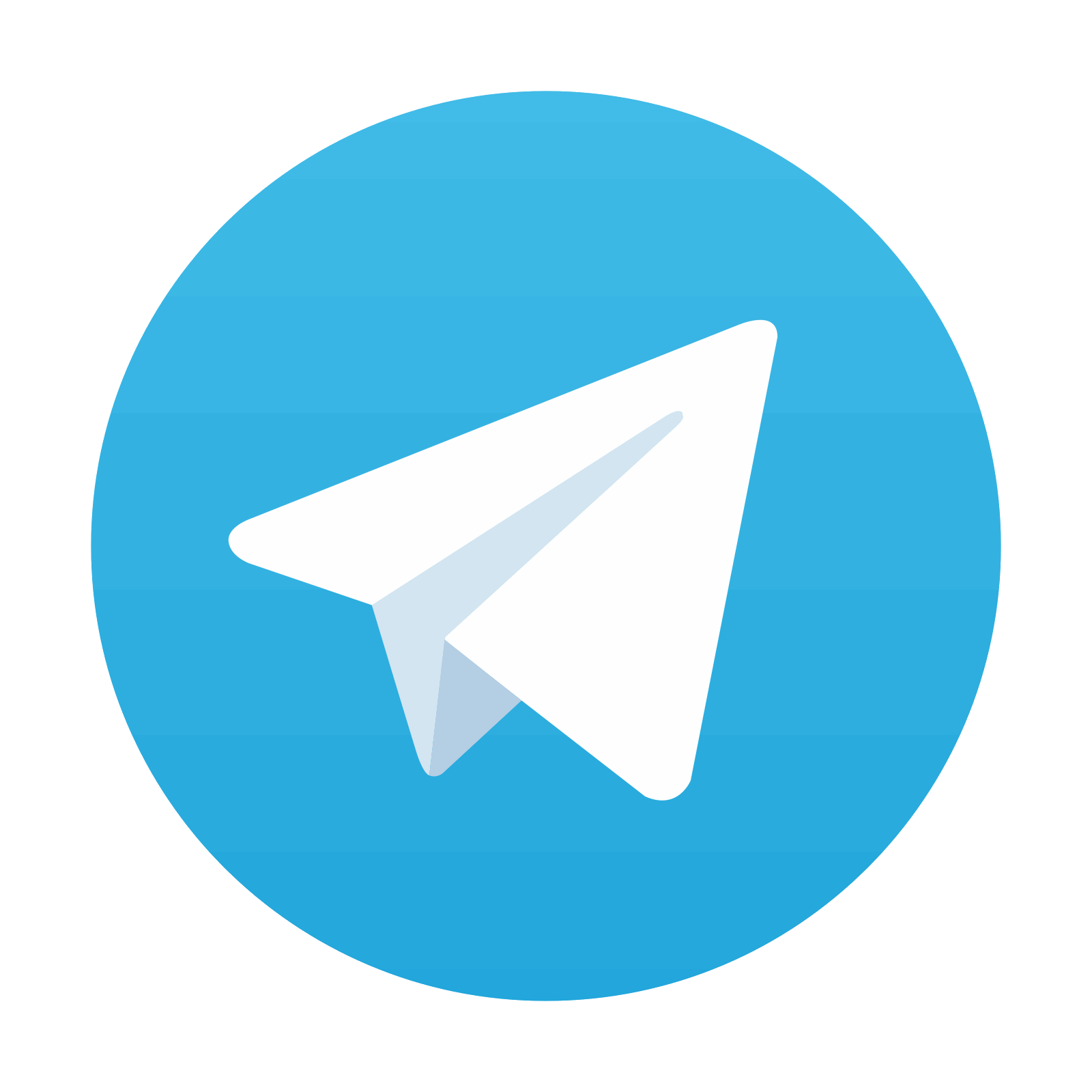
Stay updated, free articles. Join our Telegram channel

Full access? Get Clinical Tree
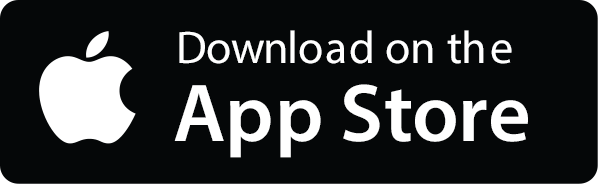
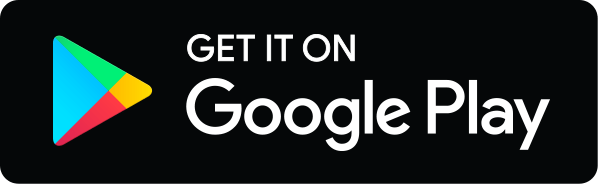