Positron emission tomography, single-photon emission tomography, and magnetic resonance spectroscopy (MRS) are powerful tools for the monitoring of diverse neurochemical functions. Neuroimaging studies targeting neurotransmitter systems in autism have provided clues about how differences in development of these systems might lead to new intervention approaches. Direct measurement of diverse neurochemicals with MRS provides unique probes of neuronal integrity in vivo. Future directions include the combination of imaging modalities made possible by advances in software and hardware. Many tracers have not been applied in autism, and new molecules and signaling pathways might be targeted as genes associated with autism are identified.
Underlying the spectrum of autistic behaviors are multiple causes, only a small fraction of which have been identified. The reliance on this behavioral definition results from the lack of biologic markers for most individuals with autistic behavior and is a source of difficulty in the design and reproducibility of imaging studies of brain neurochemistry. Although there are various causes for autistic behavior, the possibility of alteration in common signaling pathways, shared by multiple causes of autism, seems be supported by new genetic studies. Neurotransmitters in autism have been examined for more than 50 years. Methods for measuring neurotransmitters in autism have spanned biochemical measures of bodily fluids and postmortem brain, molecular imaging, and genetic measures of genes involved in neurotransmitter synthesis, transporters, receptors, and signaling pathways. There is some evidence for alteration in many neurotransmitters in autism. This review focuses on evidence for a role of altered neurotransmission in autism measured through neuroimaging techniques. The role of these neurotransmitters during different periods of brain development is discussed. Understanding the roles of each neurotransmitter in the brain across the lifespan and how those functions might be altered in autism will offer new routes for pharmacologic intervention in autism.
Neuroimaging technology has 3 primary tools to study neurochemistry in vivo: positron emission tomography (PET), single-photon emission computed tomography (SPECT), and magnetic resonance spectroscopy (MRS). Three neurotransmitter systems have been studied in autism spectrum disorders (ASD) using PET or SPECT imaging: serotonin, dopamine, and γ-aminobutyric acid (GABA). PET and SPECT make use of radiolabeled tracers to measure neurochemical metabolism and receptor binding. MRS involves the direct measurement of a small number of neurochemicals, including N -acetylaspartate (NAA), glutamate, glutamine, and GABA. Advantages of in vivo measures of neurochemistry over postmortem biochemical measures include the ability to take neurochemical measures and behavioral measures in the same individual, there is the possibility of longitudinal measures, and the issue of postmortem degradation and other effects of perimortem changes associated with mode of death is avoided. Structural imaging beginning with computed tomography (CT) and subsequently with magnetic resonance imaging (MRI) and studies of brain function by measuring changes of blood flow in the brain under different conditions, first by using oxygen 15 ( 15 O) labeled water with PET and subsequently by measuring blood oxygenation with MRI using a method called functional MRI, are beyond the scope of this review; recent reviews are available on these topics. This review begins with a discussion of the neuroimaging technologies used to measure neurochemistry in vivo, followed by a review of neurochemistry in ASD studies with neuroimaging, and ends with a discussion of future directions.
PET and SPECT methodology
PET and SPECT are techniques that can measure and image the distribution of tracers that are designed to track biochemical and molecular processes in the body after intravenous injection or inhalation. This goal is accomplished by radiolabeling compounds of interest with positron-emitting or single-photon–emitting radionuclides. PET uses short-lived isotopes (most frequently used isotopes include fluorine 18 [ 18 F] with a half-life of 110 minutes, carbon 11 [ 11 C] with a half-life of 20 minutes, 15 O with a half-life of 2 minutes) that emit positrons during nuclear decay, which in turn collide with surrounding electrons, resulting in annihilation of both particles and the release of 2 high-energy (511 keV) γ-rays. The 2 γ-rays generated by a single event travel in opposite directions and are recorded by multiple pairs of oppositely situated detectors that constitute the PET camera. For SPECT, single-photon–emitting radionuclides are used, in particular technetium 99m (Tc 99m) (half-life of 6 hours) and iodine 123 (half-life of 13 hours). SPECT imaging is performed using a γ-camera to acquire multiple two-dimensional images from different angles, and the data acquired are subjected to a tomographic reconstruction algorithm yielding a three-dimensional dataset. Thus, the time course of changes in the distribution of the radiolabeled tracer in the body is recorded by the scanners after the radioactive decay of the radionuclide. Using the dynamic tissue time-activity data obtained in this manner and appropriate kinetic modeling of tracer behavior, physiologic parameters of interest (eg, receptor density, neurotransmitter synthesis rate) can then be calculated. Absolute quantification and estimation of relevant parameters of a given biochemical pathway in a noninvasive manner distinguish PET from SPECT when absolute quantification is difficult.
Many PET radiopharmaceuticals have been developed over the past 30 years for the study of normal physiologic processes and for application to the study of many disorders. More than 1400 PET tracers have been produced, and the rate of development of new tracers seems to continue in the exponential growth phase. Radiolabeled tracers can be divided into several categories. The first group includes normal metabolic substrates or analogues of these substrates, such as glucose, amino acids, fatty acids, nucleotides, and oxygen. Another group of PET tracers includes ligands that bind to proteins such as receptors and transporters. Newer PET probes include antibodies, oligonucleotides, and tracers to image reporter genes to monitor gene therapy. The traditional PET tracers were predominantly small molecules and were produced using synthetic chemistry techniques. These techniques have produced many specific tracers useful for clinical purposes. However, increasingly PET radiopharmaceuticals are being produced by bioengineering techniques, paralleling similar developments in the drug industry. The PET tracers produced by these techniques are typically large-molecular-weight biotechnology probes. These techniques are often flexible enough to produce designer probes with the desired specificity, affinity, and other properties. Such targeted imaging can lead to imaging precise molecular abnormalities in humans in a noninvasive manner. However, these new technologies have been predominantly applied to disorders outside the brain, because the blood-brain barrier bars entry of these larger probes. Several groups of investigators are working on strategies for facilitating brain entry of these large-molecular-weight tracers. For example, targeting tracers to endogenous brain endothelial transporters such as carrier-mediated transporters, active efflux transporters, or receptor-mediated transporters has been attempted to improve brain tracer delivery.
Studies of neurotransmitter function with PET and SPECT in autism
Given the number of radiolabeled probes available for the study of neurotransmission with PET and SPECT, it is surprising that few have been used in the study of autism. Studies investigating alterations in neurotransmitters with PET in autism have focused on dopamine, serotonin, and GABA.
Studies of neurotransmitter function with PET and SPECT in autism
Given the number of radiolabeled probes available for the study of neurotransmission with PET and SPECT, it is surprising that few have been used in the study of autism. Studies investigating alterations in neurotransmitters with PET in autism have focused on dopamine, serotonin, and GABA.
Dopamine precursor and transporter studies
Ernst and colleagues studied 14 medication-free autistic children (8 boys, 6 girls, mean age 13 years) and 10 healthy children (7 boys, 3 girls, mean age 14 years) with 18 F-labeled fluorodopa (FDOPA) using PET. FDOPA is a precursor of dopamine, which is taken up, metabolized, and stored by dopaminergic terminals. Ernst and colleagues’ calculated ratio of activity measured between 90 and 120 minutes after tracer administration in the caudate, putamen, midbrain, and lateral and medial anterior prefrontal regions (regions rich in dopaminergic terminals) to that in occipital cortex (a region poor in dopaminergic terminals). They reported a 39% reduction of the anterior medial prefrontal cortex/occipital cortex ratio in the autistic group, but there were no significant differences in any other region measured. These investigators suggest that decreased dopaminergic function in prefrontal cortex may contribute to the cognitive impairment seen in autism. More recently, the dopamine transporter was studied in children with autism (10 boys, 3–10 years) and 10 age-matched and gender-matched healthy children with the tracer Tc 99m TRODAT-1 (a tropane derivative) imaged by SPECT. This study reported a whole brain increase in dopamine transporter binding in the autism group, whereas the striatum/cerebellum ratio showed no differences between the groups. Nakamura and colleagues measured dopamine transporter binding in adults with autism (20 men, 18–26 years) using [ 11 C]WIN-35,428 imaged with PET. Dopamine transporter binding was significantly higher in orbital frontal cortex in the autism group compared with 20 age-matched and IQ-matched control subjects. Makkonen and colleagues reported no difference in striatal dopamine transporter binding in 15 children with autism (14 boys, 1 girl; 5–16 years) compared with 10 nonautistic children using the tracer [ 123 I]nor-β-CIT, which labels both the dopamine and serotonin transporter, imaged by SPECT. Although this tracer labels both dopamine and serotonin transporters, the investigators made the assumption that binding in the striatum was predominantly due to dopamine transporter binding caused by the relative concentration of the transporters in this brain region. Together these studies suggest altered dopaminergic function in frontal cortical regions but not in striatum in children and adults with autism.
Serotonin precursor, transporter, and receptor studies
Although there is evidence for the potential involvement of several neurotransmitters in autism, the most consistent abnormal neurotransmitter findings involve serotonin. Schain and Freedman first reported increased blood serotonin in approximately one-third of autistic patients in 1961. Chugani and colleagues applied α[ 11 C]methyl- l -tryptophan (AMT) as a PET tracer in autistic children. AMT, which was developed as a tracer for serotonin synthesis with PET, is an analogue of tryptophan, the precursor for serotonin synthesis. Two fundamentally different types of serotonergic abnormality were found in children with autism. The first is a difference in whole brain serotonin synthesis capacity in autistic children compared with age-matched nonautistic children. Serotonin synthesis capacity was greater than 200% of adult values until the age of 5 years and then declined toward adult values in nonautistic children. In contrast, serotonin synthesis capacity in autistic children increased gradually between the ages of 2 years and 15 years to values 1.5 times the adult normal values. These data suggested that humans undergo a period of high brain serotonin synthesis capacity during early childhood, and that this developmental process is disrupted in autistic children. The second type of abnormality reported relates to focal abnormalities in brain serotonin synthesis. Asymmetries of AMT uptake in frontal cortex, thalamus, and cerebellum were visualized in children with autism, suggesting a role of the dentatothalamocortical pathway in autism. Subsequently, the same group measured brain serotonin synthesis in a large group of autistic children (n = 117) with AMT PET and related these data to handedness and language function. Cortical AMT uptake abnormalities were objectively derived from small homotopic cortical regions using a predefined cutoff asymmetry threshold (>2 standard deviations of normal asymmetry). Autistic children showed several patterns of abnormal cortical involvement, including right cortical, left cortical, and absence of abnormal asymmetry. Groups of autistic children defined by presence or absence and side of cortical asymmetry differed on a measure of language as well as handedness. Autistic children with left cortical AMT decreases showed a higher prevalence of severe language impairment, whereas those with right cortical decreases showed a higher prevalence of left-handedness and mixed-handedness. These results suggest that global as well as focal abnormally asymmetric development in the serotonergic system could lead to miswiring of the neural circuits specifying hemispheric specialization.
Decreased serotonin transporter binding has been reported in both children and adults with autism. Makkonen and colleagues using the SPECT tracer [ 123 I]nor-β-CIT labeling both the dopamine and serotonin transporter described earlier, reported reduced serotonin transporter binding capacity in medial frontal cortex, midbrain, and temporal lobes. Similarly, Nakamura and colleagues reported decreased serotonin transporter binding throughout the brain in adults with autism (20 men, 18–26 years) using [ 11 C]McN-5652 imaged with PET. Furthermore, the reduction in binding in anterior and posterior cingulate cortices was correlated with impairment in social cognition, whereas the reduction in serotonin transporter binding in the thalamus was correlated with repetitive or obsessive behavior.
Serotonergic neurotransmission was also studied using tracers for receptor binding. Murphy and colleagues measured 5HT2A receptors in 8 men with Asperger syndrome (mean age 26 years) using the SPECT tracer [ 123 I]5-I-R91150, compared with 10 healthy age-matched men. The group with Asperger syndrome had significantly reduced serotonin receptor binding in total, anterior, and posterior cingulate cortex, bilateral in frontal and superior temporal lobes and in the left parietal lobe. There were significant correlations with qualitative abnormalities in social interaction with binding reductions in anterior and posterior cortices, as well as right frontal cortex. Goldberg and colleagues compared the parents of children with autism (19 parents from 11 families, 8 women, 11 men) with adults who do not have children with autism (9 women, 8 men). The cortical 5HT2 binding potential, using [ 18 F]setoperone, to measure cortical serotonin type-2 receptor (5-HT2) using PET, was significantly lower in the autism parent group compared with the control group. Furthermore, the 5HT2 binding potential was inversely correlated with platelet serotonin levels in the parent group. These results are interesting in light of family members having what has been described as the broader phenotype of autism.
Neuroimaging studies provide convincing evidence of altered serotonergic neurotransmission in both children and adults with autism. Altered serotonin synthesis, serotonin transporter, and serotonin receptors have been measured using PET and SPECT and different tracers in small groups of children and adults with autism compared with age-matched controls.
GABA A receptor binding studies
Cytogenetic studies reported the abnormalities in chromosome 15 in autism, specifically 15q11 to 15q13, the region encoding several GABA A receptor subunit genes (GABRB3, GABRA5, and GABRG3). Menold and colleagues reported 2 single nucleotide polymorphisms located with in the GABRB3 gene in autism. Moreover, symptoms of autism can be associated with both Prader-Willi and Angelman syndromes, both of which involve alterations in the chromosome 15q11 to 15q13 region (for review see Ref. ). Deletion of the maternal chromosome in this region results in Angelman syndrome, which is characterized by severe mental retardation, epilepsy, a puppetlike gait, and lack of speech. Deletion of the paternal chromosome 15q11 to 15q13 results in Prader-Willi syndrome, which is characterized by mild or moderate mental retardation, hypotonia, obesity, and genital abnormalities. This region of chromosome 15 encodes GABA A receptor subunit genes GABRB3, GABRA5, and GABRG3. [ 11 C]Flumazenil PET has been used to examine whether there are GABA A receptor binding abnormalities in patients with Angelman syndrome and Prader-Willi syndrome. Patients with Angelman syndrome with a maternal deletion of 15q11 to 15q13 leading to the loss of β3 subunits of the GABA receptor showed significantly decreased binding of [ 11 C]flumazenil in frontal, parietal, hippocampal, and cerebellar regions compared with a patient whose deletion did not include the GABRB3 gene. Lucignani and colleagues studied 6 adults with Prader-Willi syndrome and found decreased [ 11 C]flumazenil binding in insula and cingulate, frontal, and temporal neocortices compared with normal control subjects. Pearl and colleagues studied 7 patients with succinic semialdehyde dehydrogenase deficiency with [ 11 C]flumazenil, compared with 10 unaffected parents and 8 healthy controls. Succinic semialdehyde deficiency is characterized by autistic behaviors, seizures, intellectual impairment, hypotonia, and hyporeflexia. Decreased GABA A receptor binding was lower in amygdala, cerebellar vermis, frontal, parietal, and occipital cortices in patients compared with both control groups. Thus, these imaging studies show decreased GABA A receptor binding in all of these genetic disorders in which autistic behavior is present. These studies show the usefulness of PET in elucidating the functional consequence of specific genetic abnormalities.
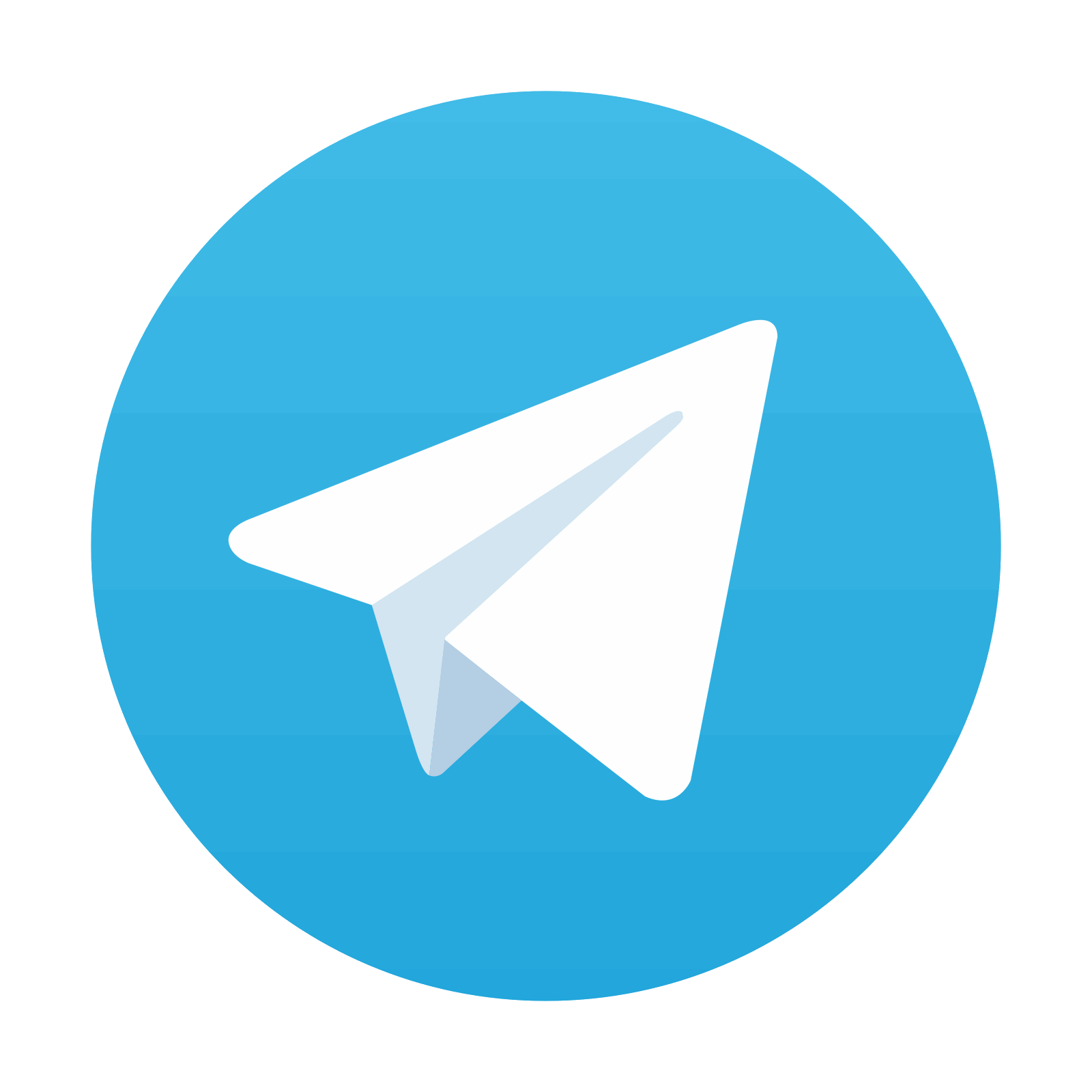
Stay updated, free articles. Join our Telegram channel

Full access? Get Clinical Tree
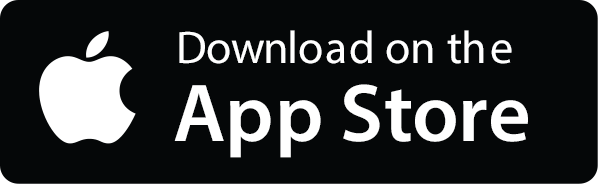
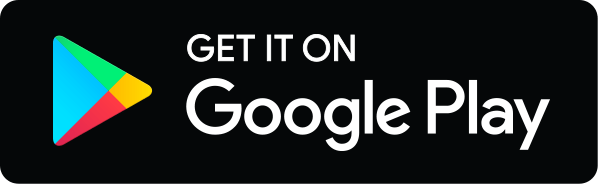