KEY POINTS
- 1.
Neonates and infants often are treated with medicines that have not been approved by regulatory authorities for use in this age group.
- 2.
The inclusion of infants in drug development studies and clinical trials is limited by difficulties in enrollment, concerns for adverse effects due to developmental limitations, and the lack of universally accepted response variables in many conditions.
- 3.
Neonatal randomized controlled trials (RCTs) are needed to evaluate the impact of interventions, from practice standards to devices to novel therapies or therapies that were developed for nonneonatal indications.
- 4.
There is a need for RCTs not only to assess effectiveness and safety of neonatal therapies but also to focus on operational issues and outcomes for regulatory consideration.
- 5.
Clinical investigations that evaluate interventions/procedures that present the prospect of direct benefit but carry a possibility of greater than minimal risk need review by institutional review boards. There is a need to ensure that the benefit-risk assessment is more favorable than that with alternative approaches.
Introduction
Neonates and infants often are treated with medicines that have not been approved by any regulatory authorities for use in this population. High-risk neonates and children in general are seen as vulnerable, with their participation in drug development and clinical trials limited by numerous factors. Some of the reasons that so few products have been developed for neonates and children include gaps in understanding complex pathophysiology, a small market with many conditions involving rare diseases, challenges in the design and execution of clinical trials (including the need to wait for long periods of time to assess some clinical outcomes), and difficulties with the assessment of safety and efficacy. This has resulted in children being referred to as “therapeutic orphans.”
This chapter will review fundamentals of the design and conduct of clinical trials in neonates and young infants. Although randomized controlled trials (RCTs) are considered the “gold standard,” the use of alternative designs (e.g., cluster randomized trials) will also be discussed ( Fig. 98.1 ). Ethical considerations, including issues related to informed consent, will be presented along with the need for engagement of parents and neonatal intensive care unit (NICU) staff in the trial design process.

Clinical Trial Designs
The likelihood that a clinical study will answer the research questions posed in a reliable manner, meaningful for decision makers and patients, while preventing important errors, can be dramatically improved through prospective attention to the design of all components of the study protocol, procedures and associated operational plans. —From the International Council for Harmonisation (ICH) E8(R1) Draft Guideline
Randomized, double-masked or blinded, placebo- or standard of care–controlled clinical trials have provided many of the key advances in neonatal therapeutics. Surfactant replacement for respiratory distress syndrome, caffeine for apnea of prematurity, therapeutic hypothermia for hypoxic-ischemic encephalopathy, and inhaled nitric oxide for persistent pulmonary hypertension of the newborn are among the most significant therapeutic successes in neonatology. Despite these successes, RCTs of preventive agents or therapies for conditions such as bronchopulmonary dysplasia and neonatal hypotension have not produced clear evidence of clinically meaningful benefit for any of the therapies studied.
RCTs remain an essential tool for assessing the effectiveness and safety of neonatal therapies, both novel therapies and those whose efficacy and/or safety may be unclear. It has been recognized that clinical trials may either fail to show an effect or fail to provide useful data due to problems with elements of design and implementation. “Quality by design” is an approach that relies on sound prospective design and execution plans such as (1) focusing on protecting human subjects, (2) determining predefined objectives, (3) selecting appropriate study participants, (4) minimizing bias, (5) controlling confounding, (6) choosing measurable and well-defined study endpoints and methods of assessment, and (7) addressing operational and feasibility criteria. ,
Human Subjects Protections and Neonatal Clinical Trials
The U.S. Department of Health and Human Services (HHS) and the Food and Drug Administration (FDA) require special protections for the inclusion of children in research. These protections are defined under HHS 21CFR§50, Subpart D, as the “Additional Safeguards for Children in Clinical Investigations” and under FDA 45CFR§46, Subpart D, as the “Additional Protections for Children Involved as Subjects in Research.” The principles are the same, but the HHS and FDA Subpart D guidelines differ slightly in scope. The HHS regulation applies to “all research involving children as subjects, conducted or supported by HHS,” whereas the FDA regulation applies only to studies of an FDA-regulated product.
Under Subpart D, an institutional review board (IRB) can approve clinical investigations involving children that fall into the following categories:
- 1.
not greater than minimal risk,
- 2.
greater than minimal risk but presenting the prospect of direct benefit to individual subjects, or
- 3.
greater than minimal risk and no prospect of direct benefit to individual subjects but likely to yield generalizable knowledge about the subjects’ disorder or condition.
An IRB may determine that a study presents no more than minimal risk if all the interventions or procedures within the clinical investigation do not exceed minimal risk. Such studies may proceed with the permission of one parent or guardian. Minimal risk is difficult to define but is indexed to the risks for the population in their daily life, including routine examinations. In the case of hospitalized neonates, a single blood draw (preferably with routine labs), bagged urine specimen, or a single chest radiograph may be considered minimal risk.
Interventions or procedures within a clinical investigation that convey greater than minimal risk but present the prospect of direct benefit (PDB) are reviewed by IRBs to ensure that the risk is justified by the anticipated benefit and that the benefit-risk assessment is at least as favorable to participants as would be available with alternative approaches. Permission of one parent or guardian must also be obtained for research approved under this category. Protocols should clearly describe available alternatives to the investigational therapy and any adverse events and long-term consequences if known. A comprehensive understanding of therapeutic alternatives is critical to benefit-risk assessments for IRBs and regulators. An example of a study in this category may include evaluation of a novel therapy in a pediatric population for which the PDB to the child has been established and justifies the risk of exposure. Such an assessment should include whether there is adequate evidence to establish proof of concept to support a benefit and whether the proposed dose and duration of therapy are sufficient to offer a potential clinical benefit ( Table 98.1 ).
Factors That May Support a Prospect of Benefit for a Novel Therapy | Tools to Support Benefit and Minimize Burdens/Risks |
---|---|
Evidence to support proof of concept | |
Biological plausibility and scientific justification for the proposed mechanism of action and its expected effect in the condition of interest | Evaluate existing scientific rationale, summarize available experimental and observational data |
Nonclinical evidence ( in-vitro mechanistic studies, in-vivo studies in animal models) | In vivo data in animal models of disease can provide insight into the impact of the intervention on pathophysiology as well as dosing strategy |
Clinical evidence in adults or older children with similar and/or relevant conditions | Efficacy responses may support benefit and data on adverse effects can provide important information to guide monitoring in a neonatal clinical trial |
Structure of the study intervention | |
Dosing justification including evidence to support that the proposed doses are likely to have the intended treatment effect |
|
Trial duration |
|
Interventions or procedures in a clinical investigation that pose greater than minimal risk (referred to as a “minor increase over minimal risk”) without a PDB for individual subjects but that is likely to yield important, generalizable knowledge about the participant’s condition may be approvable if the minor increase is commensurate with expected medical experiences for the subject. Clinical investigations approved under this category require permission of both parents.
An example of a clinical investigation that might be allowed to proceed with a minor increase over minimal risk would be the evaluation of a single dose of an investigational product solely to collect pharmacokinetic data for which adequate safety data exist to characterize the risk as no more than a minor increase over minimal risk. For a clinical investigation to proceed under this category, all the interventions and procedures in the protocol (e.g., blood draws or any diagnostic testing) also need to be no more than a minor increase over minimal risk. This analysis is called a component analysis of risk , used to determine the overall acceptability of the clinical investigation including the risks and anticipated benefits of the interventions that are listed in the protocol (which must be analyzed individually as well as collectively). ,
Selection of Study Population
For RCTs of interventions to treat a disease or condition, study participants should have the disease or condition with clear and reproducible diagnostic criteria. If the disease or condition is ill-defined either physiologically or clinically, enrolled patients may be a heterogeneous group. Identifying a potential treatment effect may be impossible in a study that enrolls patients with variable manifestations of a condition.
For RCTs of interventions to prevent a disease or condition, study participants must be at significant risk for developing that disease or condition. When the risk of a disease is low in a given population, participants could be enrolled in a study and exposed to an experimental intervention to prevent an infrequent outcome. In this scenario, understanding the safety of the study intervention is critical to any benefit-risk assessment. Strategies to identify a population at higher risk of the condition should reduce the proportion of enrolled neonates who would likely not have developed the condition. Enrichment strategies may include well-evaluated biomarkers and/or risk scores that are derived from clinical data.
Inclusion and exclusion criteria should be clearly defined and used to capture the population for whom the intervention is intended. Early-phase studies of a novel therapy in neonates may require study in a relatively homogeneous population to balance the unknown risks with the potential for individual benefit. Once more is known about the product’s safety profile, later-phase RCTs may be able to enroll a more heterogeneous sample of patients that is similar to the intended use population.
Therapeutic Intervention
Neonatal RCTs may evaluate the impact of interventions, from practice standards to devices to novel therapies or therapies that were developed for nonneonatal indications. Subpart D guidelines, as discussed above, help guide the benefit-risk assessment for a trial protocol. An intervention that conveys more than a minor increase over minimal risk must provide a PDB to participants, with rare exceptions. In this category, clinical equipoise is assumed—the “honest, professional disagreement among expert clinicians about the preferred treatment” and IRBs must determine whether the potential benefit justifies the risks. PDB can be established based on information from the literature, animal models, other nonclinical studies, and/or proof-of-concept studies in humans. Study protocols should include all available nonclinical and clinical evidence to support both PDB and safety to inform IRBs and regulatory bodies.
Nonclinical toxicology studies that support drug approval in adults or older children may not be sufficient to inform the safety of a treatment in the neonatal period. When a drug’s impact on developing organ systems is unknown, juvenile animal studies can inform safety and/or dosing parameters. Safety and tolerability information from healthy adult volunteer studies may also be needed to support neonatal studies if the drug has not been previously given to humans.
The importance of establishing effective and safe dosing strategies prior to initiation of large RCTs cannot be overstated. Thoroughly evaluating existing pharmacokinetic information and drug absorption, distribution, metabolism, and excretion data is critical to optimizing the dosing strategy and reducing the chance of trial failure due to suboptimal dosing. Although dose selection is typically confirmed in phase 2 studies, later RCTs may incorporate more than one dosing regimen for confirmation.
Choice of Endpoint or Response Variable
The choice of a primary endpoint is fundamental to the quality of the study. The primary endpoint should be the variable capable of providing the most clinically meaningful and convincing evidence directly related to the primary objective of the trial. The outcome that parents, neonatologists, and clinical staff most desire is a healthy child with optimal long-term cognitive, social, and physical abilities. Unfortunately, these efficacy objectives may be too distant to feasibly measure for most interventional trials, and there are numerous intervening factors between the NICU hospitalization and later childhood that may influence the effects of any neonatal intervention.
The prespecified primary endpoint should be well-defined in the protocol and its rationale explained. If there are several coequal primary outcomes, then the sample size calculation and analysis plan must account for multiplicity by controlling the type I error rate (the probability of rejecting at least one null hypothesis if the treatment has no benefit). If the sample size is not sufficient for testing more than one primary outcome, then the other measures of interest can be captured as secondary endpoints and may be supportive to the primary endpoint.
It is rare in neonates to be able to design a trial with a simple primary efficacy endpoint. In studies enrolling neonates of extremely low gestational age or other high-risk patients, death is a competing outcome that must be analyzed. The difference between two groups’ proportions of a composite of “death before 36 weeks’ postmenstrual age or morbidity” is a straightforward endpoint for powering an RCT, but results may be difficult to interpret if the two elements of the composite go in opposite directions. An alternative approach would be a composite that ranks the potential outcomes from best to worst (or worst to best), incorporating the input of family members as well as clinicians and researchers. If the two elements were death and morbidity, then stakeholders would most likely rank death as the worst outcome, followed by surviving with morbidity, and finally surviving without morbidity (best). This approach can be extended to scenarios in which data collection is richer, such as when survival is captured as a time-to-event outcome and/or morbidity is measured longitudinally.
Other composite endpoints, made up of multiple variables, have been used to assess a group of undesired outcomes, any one of which would constitute a negative result. Morbidity counts could provide an additional way to differentiate negative outcomes. One caveat is that composites can be challenging to apply clinically, because a result for the composite does not imply a result for the individual elements. An alternative to composites is to collect and analyze mortality and morbidity as time-to-event outcomes. Then, hazard ratios and cumulative incidence functions can be estimated for the individual elements, accounting for the competing risk. The illness-death model (also called the semicompeting risk model) is an extension of the competing risk model and may be used if a patient could die before or after developing the morbidity of interest.
Biomarkers, or surrogate endpoints, can be useful in assessing pharmacodynamic effects in a proof-of-concept trial prior to designing a fully powered RCT to evaluate clinically meaningful endpoints. Biomarkers to help establish proof of concept could include imaging studies or laboratory tests. In rare cases in neonatology, a biomarker may be used as a primary efficacy endpoint. Continuous video electroencephalogram monitoring to evaluate reduction in seizure burden was accepted by a data-driven, expert consensus as a primary endpoint for neonatal seizure trials. Additional biomarkers developed using clinical data and consensus processes will streamline clinical trials for other neonatal conditions.
Collection of Adverse Event Information
Adverse events (AEs) must be systematically collected in neonatal clinical trials in a similar fashion to other populations. AEs may be difficult to distinguish from typical neonatal complications and morbidities, especially in extremely preterm neonates and those with complicated disease processes. Nonetheless, RCTs provide an important opportunity to investigate differences in the frequency of AEs between active treatments or between treatment and placebo. Understanding background rates of morbidities can provide a critically important perspective when trial rates of specific neonatal morbidities are assessed.
Investigators and sponsors are responsible for collecting and reviewing serious AEs and keeping track of other sources of relevant data on the study intervention. RCT protocols must include a prospective plan for the monitoring and reporting of AEs. Regulatory authorities require timely reporting of serious and unexpected AEs for review. An AE severity scale specific to neonatal clinical trials has been developed and is undergoing validation. Consistent and standardized laboratory values have been established for trials for older children and adults, but standardized neonatal laboratory values do not exist and are urgently needed.
Selection of the Control Group
The purpose of a control group is to allow the researcher to determine whether a difference in outcome between groups is caused by the intervention itself and not due to other factors (natural history, other treatments, comorbidities, etc.). Randomized trials may be designed with a placebo control arm, a standard-of-care control arm without placebo, an active control arm, or a dose-response concurrent control arm. In special cases such as rare and ultrarare diseases, external or historical (nonconcurrent) controls may be considered.
The advantages of placebo- or standard of care–controlled trials, when well blinded or masked, are well understood; with effective randomization, a difference in effect between the intervention arm and the control arm should be attributable to the intervention. When a condition is usually treated with a standard therapy that is thought to be effective, and withholding that therapy would be considered unethical, an active control arm or an add-on design may be used if scientifically appropriate. In an add-on design, both arms receive the standard therapy with the investigational arm also receiving the treatment being evaluated.
Randomization
Randomization helps to ensure that the treatment and control arms are comparable, with similar background characteristics and prognostic factors (whether the factors are measured or not). Randomization also mitigates potential biases that may occur when assigning patients to the intervention group or the control group. Biases may be either conscious or subconscious and may relate to a participant’s prognosis or the investigator’s assumptions about a participant or a treatment. When allocation is biased, the comparison between arms is easily invalidated. Theoretically, if the sample size is large enough, all variables will have the same distribution in each of the study arms. However, due to finite sample size, imbalance is common. Therefore investigators should stratify the randomization on key factors (to the extent possible—see below), check for imbalance between groups, and adjust the analyses for baseline variables that may be related to outcomes and are found to differ between groups ( Table 98.2 ).
Strategy | How It Works | Advantages | Limitations |
---|---|---|---|
1:1 randomization | Participants are randomized 1 to 1 to intervention or control | Efficiency and simplicity | Potential imbalance in important variables |
2 (or more):1 randomization | Participants are randomized 2 (or more) to 1 to intervention or control | More participants receiving the intervention may improve detection of adverse events | Requires more complex statistical analysis |
Block or stratified randomization | Participants are randomized with equal probability in small groups (e.g., 4, 6, 8); may be assigned by key prognostic variable | Helps achieve balance between arms on factors that may change during the course of enrollment (e.g., severity of illness) | Too many strata relative to sample size may not be balanced; requires adjustment for the stratification variable in the analysis |
Cluster randomization | Randomization occurs at the level of the clinical setting (unit or practice) or cluster | Eliminates contamination; able to assess effectiveness in practice | Similarity of patients in a cluster or center to one another; may require complex balancing techniques |
Most trials are randomized with a 1:1 ratio of patients to the intervention or control arms. This is generally the most efficient design, but there are sometimes reasons for using an alternative schema. For example, allocating more patients to the active intervention arm can improve power for detecting AEs, or it may be desirable to compare nonrandomized groups within an arm of an RCT, such as in a surgery arm that incorporates more than one surgical technique.
Block randomization is usually used instead of simple randomization to ensure approximately equal sample size across study arms. Stratification of the randomization on important prognostic factors ensures balance on those factors across study arms. The selection of factors to stratify on depends on the specific study design, outcomes, and sample size. Typical factors include sex and gestational age, and stratification by site can eliminate any influence of site effects on trial results. Any variable used for stratifying the randomization should be adjusted for in the analysis. If the number of strata is large relative to the sample size, stratification may not achieve balance on all factors. In that case, covariate adaptive randomization may be used instead of block randomization.
Two interventions can be tested in the same study using a 2 × 2 factorial design such as testing both a nutritional and a drug intervention for a specific condition. The trial would have four arms (nutrition and drug; nutrition and no drug; no nutrition and drug; no nutrition and no drug) and would be conducted similarly to a four-arm RCT. The effect of each intervention, the additive effect of the interventions, and the interaction of the interventions could be tested. This depends on the study objectives and hypotheses and assumes a sample size that accounts for multiple testing.
Cluster-randomized controlled trials (C-RCTs) are randomized at the level of the cluster or clinical setting. In the case of neonatal trials, the cluster likely represents the neonatal intensive care unit or nursery, depending on the intervention. A C-RCT design can eliminate contamination, which can occur when patients in the control group are inadvertently exposed to the intervention. Even when patients are randomized in an RCT to test efficacy, the trial may be followed by a separate trial to examine effectiveness. This process can assess whether the treatment that was tested in a highly controlled environment maintains its effectiveness under less stringent conditions, such as heterogeneous practice settings. The cluster effect (that is, the tendency of patients in a cluster to be more similar to each other than to patients in other clusters) must be accounted for when determining sample size or analyzing a C-RCT. Achieving balance through allocation of clusters to study arms may require techniques that are more complex than block randomization or stratification, such as minimization or covariate constrained randomization.
Adaptive Strategies
The term “adaptive design” can have numerous definitions. However, there are several common strategies that may be used to adjust a study protocol to incorporate information that was not available at the start of the trial. Adaptive trial strategies should be anticipated prospectively and described clearly in the protocol. Potential advantages of adaptive designs include improved statistical efficiency (modification of sample size or group sequential design), improved understanding of drug effects (adaptive enrichment), ethical considerations (stopping rules), and/or acceptability to stakeholders due to added flexibility. Limitations of adaptive trial designs include the requirement for special analytical methods to avoid introducing bias or making erroneous conclusions, challenges in anticipating all potential outcomes, logistics of maintaining trial integrity, and difficulty with interpretability.
Blinding or Masking of Assignment
Conscious or unconscious biases during the conduct and analysis of a clinical trial may be curbed by masking or blinding the participants’ assigned treatment arms, although the possibility of bias is never eliminated entirely. In the case of neonatal RCTs, the investigators and clinical staff as well as family members should be blinded whenever possible. If a treatment is unable to be adequately blinded or masked, elements of study conduct, including clinical care guidelines (e.g., threshold values for tapering or increasing treatment), ascertainment of endpoints, and reporting safety outcomes, must be clearly specified in the protocol.
Informed Consent
Obtaining informed consent for participation in a research study is a legal, ethical, and regulatory requirement. Inherent in the consent process is the obligation to provide potential subjects or their proxies with adequate information regarding the study to ensure that their permission has been appropriately obtained. Inherent to informed consent in children are certain important concepts: (1) the parent(s)/guardian(s) must have the capacity to decide that their child can participate in a research study; (2) there should be full disclosure of all relevant information, especially the risks and benefits; (3) the study should be presented in the simplest way possible; (4) the decision to participate must be voluntary; and (5) informed consent should be a comprehensive communication process that is not limited to simply reviewing the informed consent form (ICF). Informed consent is even more complicated and necessary in vulnerable populations such as critically ill neonates and children.
Although a comprehensive consent process is important, previous studies have shown that researchers often fall short of developing appropriate ICFs. For example, the length of the ICF has nearly tripled during the past 20 years (along with the complexity of study protocols), primarily due to institutional language relating to legal, ethical, insurance, and financial matters. There is no evidence that these longer ICFs have led to a better understanding of study procedures, risks, or benefits or have improved the safety of research subjects. ICF language continues to be problematic, especially in view of significant health literacy issues in the United States. Finally, although diverse populations must be included in clinical trials, it may be impossible to translate the ICF into enough languages to meet the needs of a clinical trial.
The utility and potential advantage of a more concise and functional ICF was studied by Murray and colleagues in parents whose children had previously participated in or had been eligible to participate in a pediatric clinical trial. The experimental ICF (with key information up front in a two-page summary and the rest of the required information in an appendix) was developed from a previous conventional ICF for a neonatal study. Either the more concise or the conventional ICF was randomly distributed to members of two parental advocacy groups, with 72% (59–82) responding. Significantly more parents in the more concise ICF group found the form “short and to the point” but thought it did not provide enough information. However, there was no evidence that understanding of key study components was impacted by how the information was presented.
The HHS recently revised the Common Rule, a set of federal regulations (Fed Reg) for ethical conduct of human-subjects research. One of the important changes is that similar to the Murray study, key information that is most relevant to a person’s decision to participate is presented up front in the ICF in a concise and focused way. This more concise ICF might also prompt enriched discussion between the investigator and participant, an approach that has been shown to increase research participants’ understanding of study-related material. , It will be important to study parent or guardian comprehension using this new ICF in actual randomized controlled trials.
Other innovative approaches to improving informed consent have been developed. Rosenfeld and associates used visual aids in the consent process and found these had a strong influence on parental comprehension. Multimedia patient educational interventions have also been found to be more effective when added to the traditional informed consent process. Clinical investigators should continue to explore alternative methods of providing enhanced informed consent while maintaining equipoise regarding the range of outcomes that the intervention should be designed to achieve.
Recruitment and Retention of Trial Participants
Clinical trials are becoming increasingly complex, and there are multiple opportunities for failure throughout the lifecycle. Failure can arise from a variety of causes including (1) lack of efficacy, (2) issues related to safety, (3) inadequate funding, (4) inability to maintain strict manufacturing protocols, (5) regulatory issues, (6) a poorly trained workforce, (7) overly restrictive inclusion/exclusion criteria, and (8) problems with patient recruitment, enrollment, and retention. In fact, most clinical trials fail due to the inability to recruit the projected number of subjects (based on sample size calculations) and are unable to enroll a diverse and representative population.
Adequate representation must be included in all National Institutes of Health (NIH)-supported research studies, because the effects of a drug may vary significantly in different gender, racial, and/or ethnic groups. Despite this, many pediatric clinical trials do not report the racial and ethnic composition of their study populations. Nicholson and associates analyzed 165 clinical trials published between 2004 and 2014 and identified barriers to recruitment and retention of underrepresented populations, including language, trust/mistrust of the medical system, inclusion/exclusion criteria, socioeconomic status, and others.
Strategies to improve recruitment include proactive or direct (face-to-face) communication, reactive or indirect (word of mouth, printed material, or broadcast media) communication, and/or multifaceted strategies such as compensation, flexibility, building rapport and trust, and employing an ethnically and culturally diverse research staff. Building trust through community-based participatory research strategies is associated with higher enrollment of potential subjects. There is also increasing use of social media to improve recruitment of eligible study participants. These approaches allow investigators to consider the voices of many key stakeholders such as patients, physicians, disease advocates, disease foundations, researchers, and medical centers, who may all have social media pages and accounts. Topolovec-Vranic and Natarajan reviewed 30 studies that described the use of various recruitment strategies and found in 12 studies that social media was the most effective recruitment approach, especially for hard-to-reach populations and observational studies.
Once neonates are enrolled in a trial, it is essential to maintain the family’s engagement in order to obtain complete and high-quality longitudinal data to support safety and/or efficacy of an intervention. Strategies that have been used to optimize retention efforts in longitudinal clinical trials include (1) incentives (financial), (2) a personal approach (birthday cards), (3) a dedicated phone line (providing cell phones), (4) project identity and logos, (5) participant convenience, and (6) repeated contact with participants.
Long-Term Follow-Up in Neonatal RCTs
“Long-term” is an ill-defined term in neonatal and pediatric research. In neonatal RCTs, long-term is generally considered to be an evaluation at a minimum of 12 months’ corrected gestational age (CGA). The National Institute of Child Health and Development’s Neonatal Research Network has conducted multiple studies evaluating developmental milestones at 18 to 24 months’ CGA using gold-standard examiners. There are other research networks worldwide that evaluate long-term follow-up, particularly for extremely preterm neonates (<28 weeks’ gestation at birth).
Long-term follow-up evaluations may be conducted to evaluate the safety of a study intervention or to evaluate its efficacy. When the primary objective of a trial is to study neuroprotection, neurodevelopmental evaluation at ≥2 years of age is likely to be the primary endpoint for the trial. The multicenter RCTs that established therapeutic hypothermia as the standard of care for moderate-severe neonatal hypoxic-ischemic encephalopathy measured their primary endpoints of death or moderate to severe disability at 18 to 22 months’ CGA. ,
In other cases, post-NICU discharge outcomes may be assessed to establish the durability of the effect or the impact of the therapy on outcomes that are clinically significant. , Trialists should seek to understand whether a therapy that improves physiologic parameters in the short-term has either a neutral, positive, or negative long-term impact on later clinical outcomes. However, in many neonatal trials, the primary endpoint will have been measured sooner than 18 to 22 months’ CGA, with long-term evaluations primarily focused on a safety assessment of the therapeutic product or intervention. Evaluations must be tailored to the study intervention and may include not only neurologic and developmental testing but social and behavioral testing and organ-specific evaluation.
The Caffeine for Apnea of Prematurity (CAP) trial was designed to evaluate a composite primary efficacy endpoint of death, cerebral palsy, cognitive delay, deafness, or blindness at 18 to 21 months’ CGA. Participants in the caffeine arm were less likely to experience the composite endpoint, chiefly driven by decreased rates of cerebral palsy and cognitive delay. The investigators continued long-term follow-up evaluations of the participants to ages 5 and 11. By age 5, the differences between caffeine and placebo arms had disappeared. Academic and behavioral performance were again similar at age 11, but caffeine was associated with a lower rate of motor impairment. These long-term follow-up studies provided support for the safety of this widely used therapy.
Stakeholder Engagement in Neonatal RCT Design
RCTs need to be designed with the rigor required to answer questions that are important to clinicians and families while remaining feasible and acceptable. For this to occur, the voices of parents, nursing and other clinical staff, and when possible, NICU graduates should be included in the design of neonatal trials. Families and patients have lived the short-term and long-term consequences of the neonatal conditions under evaluation. Their experiences may be systematically gathered to help understand how parents would prefer to be approached for trial enrollment, what study procedures will be acceptable, and what endpoints are important to them. Nurses, respiratory therapists, and social workers also provide unique perspectives about families in times of distress and uncertainty.
To develop the tools, standards, and approaches needed to accelerate drug development in neonates and children, the FDA and the Critical Path Institute, with support from the pharmaceutical industry, established the International Neonatal Consortium (INC) in 2015. This organization represents a unique public-private partnership that brings together global regulators (FDA, European meadicines agency [EMA], Health Canada, and pharmaceuticals and medical devices agency [PMDA]), neonatologists, nurses, pharmaceutical companies, funding organizations, and parent/patient advocacy groups to develop tools to address the unmet therapeutic needs of neonates. The INC also established a clear path forward by focusing on parental input in the development of clinical trial protocols, developing master protocols and other tools to streamline clinical trials, and training investigators to conduct high-quality neonatal clinical trials. Operating in the precompetitive space, the INC addresses the need for measurement and assessment of clinical outcomes in neonates through teams that share data and expertise to advance regulatory science. Consortia such as INC have played a key role in addressing the shortfalls of neonatal drug development and clinical trials and have helped design a path forward.
Summary Conclusions
Planning and conducting neonatal RCTs is a complicated, expensive, and labor-intensive process, yet RCTs remain a critical tool for developing and evaluating effective and safe treatments for neonates. Understanding and using the framework of human subject protections and the components of “quality by design” in planning RCTs will help ensure that neonates are not subjected to undue risks without potential benefit. Neonates should only be enrolled in studies that are carefully designed with (1) a well-defined, at-risk population, (2) a clearly defined and clinically meaningful primary endpoint, (3) an evidence-based dosing strategy, (4) a feasible sample size, and (5) clear guidelines for AE collection and reporting. Input from families, nurses, physicians, and other clinical staff should be sought when developing research questions and protocol elements to ensure meaningfulness and feasibility.
Acknowledgment
The authors would like to acknowledge Susan McCune, MD, for her thorough, insightful review of this chapter.
REFERENCES
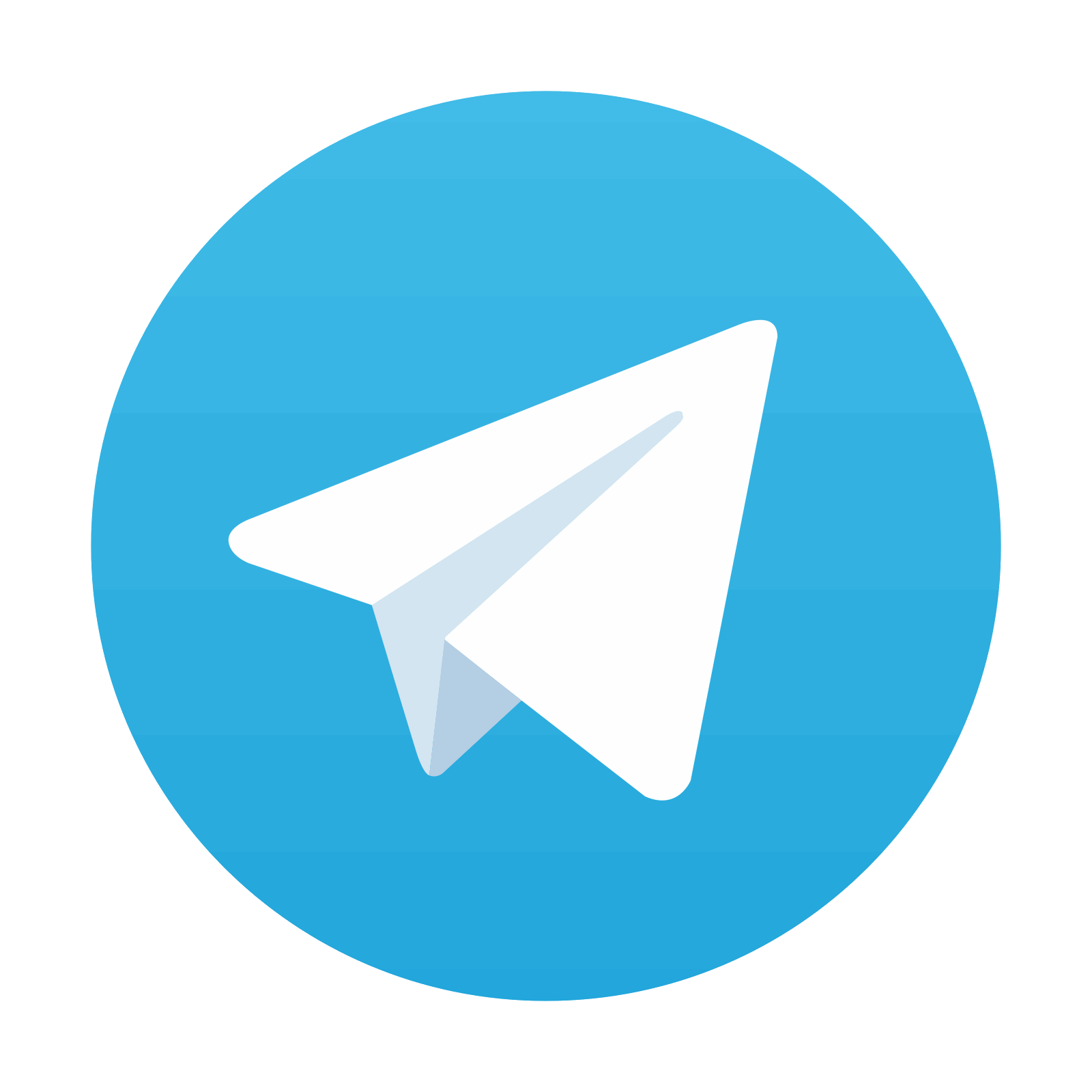
Stay updated, free articles. Join our Telegram channel

Full access? Get Clinical Tree
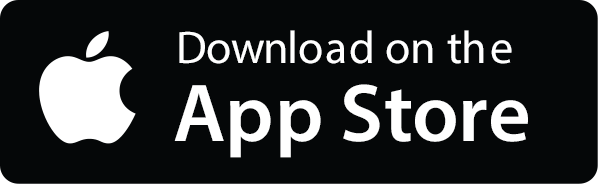
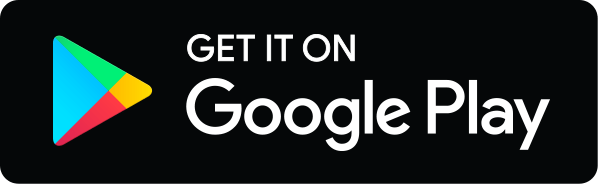