KEY POINTS
Key Points
-
Open spinal cord defect that protrudes dorsally, is not covered by skin, and is associated with spinal-nerve paralysis.
-
Current prevalence in the United States is still 0.5 to 1 case per 1000 pregnancies.
-
Maternal risk factors include obesity, anticonvulsant medication, short interpregnancy interval, and genetic polymorphisms in various enzymes in the homocysteine methylation pathway.
-
Sonographic findings include a small biparietal diameter, scalloping of the frontal bones, a compressed cerebellum, lateral ventriculomegaly, Chiari II abnormalities, splaying of dorsal vertebrae, and a dorsal sac.
-
Karyotype should be performed, even in isolated neural tube defects.
-
A prospective clinical trial comparing outcomes for open fetal surgery compared to standard postnatal surgery is in progress.
-
Long-term outcome depends on the level of the spinal defect and the presence of associated anomalies.
-
Morbidity has been significantly improved by interventricular shunting for hydrocephalus and clean intermittent bladder catheterization to reduce renal complications.
-
Most neural tube defects are isolated and have a multifactorial inheritance pattern.
CONDITION
Myelomeningocele is an open spinal cord defect that protrudes dorsally, is not covered by skin, and is usually associated with spinal nerve paralysis. Technically, spina bifida refers to a cleft or opening in the vertebral body; this term is also used to collectively describe a group of disorders that involve the spinal cord. Lumbosacral lipomas are subcutaneous masses of fat in the lumbosacral region (Shurtleff and Lemire, 1995). Myelomeningoceles are malformations that result from failure of the neural tube to fuse during early embryogenesis, between 25 and 28 days postovulation, when the anterior and posterior neuropores close. Skin-covered defects, such as lipomyelomeningocele, result from abnormalities in secondary neurulation and retrogressive differentiation that occurs between 28 and 56 days postovulation (Shurtleff and Lemire, 1995).
The first medical report of a myelomeningocele was made by a Dutch physician, Nicholas Tulp, who practiced between 1593 and 1674. He described a series of six cases of patients with spina bifida (Tulp, 1716).
“Open” neural tube defects are myelomeningoceles that are not covered by skin. Leakage of α-fetoprotein (AFP) from the cerebrospinal fluid (CSF) into the amniotic fluid results in an increased transport of AFP into the maternal circulation. Screening by maternal serum AFP analysis has resulted in an increased ability to detect these lesions prenatally.
Herniation of the spinal cord probably reduces intraspinal pressure. This allows the hindbrain to become downwardly displaced, resulting in the hindbrain herniation that is part of the Chiari type II malformation, which is seen almost exclusively in patients affected with myelodysplasia. It is characterized by caudal movement of the cerebellar vermis, brainstem, and fourth ventricle. The Chiari type II malformation is responsible for many of the early deaths in patients affected with myelodysplasia. Chiari, a professor of pathology in Prague, wrote two papers in 1891 and 1896 that described four types of pathologic changes occurring in 40 affected patients (Rauzzino and Oakes, 1995). These changes concerned the abnormal position of the cerebellum in relation to the foramen magnum. Arnold, in contrast, published a single case report in 1894 that described a single patient with myelodysplasia and other congenital anomalies (Rauzzino and Oakes, 1995).
INCIDENCE
The incidence and livebirth prevalence of myelomeningocele are correlated strongly with ethnic and geographic factors. The highest frequencies of neural tube defects are found in Great Britain, Ireland, Pakistan, Northern India, Egypt, and Arab countries. The lowest incidences are found in Finland, Japan, and Israel. Even in the United States, a geographic distribution of these defects occurs, with the frequency being the highest in the East and the South, and the lowest in the West (Harmon et al., 1995). There is an increased incidence of neural tube defects in Hispanics, especially if the mother was born in Mexico (Shaw et al., 1994).
The livebirth prevalence of infants with myelomeningocele has changed dramatically since the advent of widespread maternal serum screening for AFP. Before 1980, the livebirth prevalence of infants with neural tube defects was between 1.5 and 4.5 per 1000 livebirths. After 1980, this decreased to 0.74 to 2.5 per 1000 livebirths (Shurtleff and Lemire, 1995). In the United States, the current prevalence of neural tube defects is 0.5 to 1 per 1000 pregnancies (Shaer et al., 2007). The lowest incidence of neural tube defects is in African blacks, who have a livebirth prevalence of 1 per 10,000 (Shurtleff and Lemire, 1995). Because the screening tests detect anencephaly (see Chapter 7) as well as myelomeningocele, and many parents decide to terminate pregnancies affected with the uniformly fatal anencephaly, the incidence of myelomeningocele has increased as a percentage of all cases of neural tube defects (Rieder, 1994).
In a review of factors associated with neural tube defects in the NIH-sponsored Collaborative Perinatal Project that reviewed the pregnancies of 53,000 pregnant women, Myrianthopoulos and Melnick (1987) demonstrated that maternal diabetes mellitus, heart disease, lung disease, and use of diuretics, antihistamines, or sulfonamides were all associated with an increased risk of neural tube defects. In addition, women who had a short interval between the end of the previous pregnancy and the current pregnancy had an increased incidence of neural tube defects.
More recently, it has been shown that maternal use of the anticonvulsants valproic acid and carbamazepine, or the folic acid inhibitor aminopterin, is also associated with neural tube defects. Similarly, a maternal history of prepregnancy obesity or type II diabetes increases the chance of conceiving a child with neural tube defect (Shaw et al., 2000; Watkins et al., 2003). Neural tube defects are also associated with low socioeconomic status, a positive family history, and twinning. In general, females are affected more often than males (Källén et al., 1994).
Maternal polymorphisms or mutations in various enzymes in the homocysteine remethylation pathway confer an increased risk for fetal neural tube defects. The enzyme 5,10methylenetetrahydrofolate reductase (MTHFR) is important in the production of the circulating form of folic acid. Specific polymorphisms in the gene for MTHFR, such as maternal homozygosity for the 677T allele, or compound heterozygosity for the C677T/A1298C alleles, carry an increased risk (Peadar et al., 2004). Maternal variation in the gene for another enzyme, methionine synthase (MTRR) also carries an increased risk for affected fetuses when present in combination with an MTHFR polymorphism or low B12 levels (van der Linden et al., 2006).
The etiology of myelomeningocele is thought to be multifactorial in most cases; however, women who preconceptually take at least 400 μg of folate a day for 3 months have 70% to 80% reduction in risk of their fetuses having open neural tube defect (Centers for Disease Control and Prevention, 1992). The Center for Disease Control and Prevention estimated that the rates of open neural tube defects, both anencephaly and myelomeningocele, have fallen by 26% in the United States, compared to before 1998 when mandatory folate fortification of cereal grain products began (Matthews et al., 2002; Centers for Disease Control and Prevention, 2004).
SONOGRAPHIC FINDINGS
Prior to the mid-1980s, sonographic diagnosis of myelomeningocele relied on the meticulous scanning of the fetal vertebrae for abnormalities. Using this method, neural tube defects were missed. More recently, the prenatal sonographic diagnosis of myelomeningocele has been enhanced by the recognition of specific brain abnormalities that generally precede detection of the spinal lesion (Blumenfeld et al., 1993) (Table 19-1).
Intracranial Findings Small biparietal diameter Small head circumference Lemon sign—scalloping of frontal bones Banana sign—compressed cerebellum Lateral ventriculomegaly Chiari II abnormalities Beaking of tectum Hindbrain herniation—cerebellar vermis through foramen magnum Elongation and kinking of medulla Obliteration of cisterna magna |
Spinal Findings
Splaying of dorsal vertebral elements Meningocele sac Myelomeningocele sac Presence of placode on surface of sac Neural elements bridging across the sac |
The central nervous system (CNS) abnormalities described in neural tube defects include cerebral ventriculomegaly, microcephaly, abnormalities of the frontal bone, and obliteration of the cisterna magna with an apparently absent cerebellum or an abnormal concavity of the cerebellar hemispheres. These latter abnormalities have been referred to as the “fruit” findings, which include the lemon and the banana signs. The lemon sign (Figure 19-1) describes a concave or flattened frontal contour of the fetal calvarium rather than a normal convex frontal contour. The banana sign (Figure 19-2) describes the posterior convexity of the cerebellum within the posterior cranial fossa (Nicolaides et al., 1986). The lemon sign has been described in 1% of apparently normal fetuses, whereas the banana sign is not found in normal fetuses. The abnormal CNS sonographic findings are a consequence of the Arnold–Chiari malformation. In a prospective analysis, Campbell et al. (1987), who studied 436 fetuses at high risk for spina bifida, identified 26 fetuses with an open neural tube defect. Of the 26, 17 (62%) had a small biparietal diameter for gestational age, 9 (35%) had an abnormally small head circumference, and 100% had a positive lemon sign. In addition, 25 of 26 fetuses (96%) had a cerebellar abnormality. Of these, nine had an absent cerebellum, and 16 had a positive banana sign. Only one fetus in the study with an open neural tube defect had a normal cerebellum. These findings were further defined by Van den Hof et al. (1990), who demonstrated that the CNS abnormalities seen in myelomeningocele evolve with gestation. These authors studied 130 fetuses with open spina bifida and demonstrated a relationship between gestational age and the presence of the lemon and banana signs. A lemon sign was present in 98% of fetuses with open spina bifida at ≤24 weeks of gestation, although this finding was seen in only 13% of fetuses at >24 weeks. Cerebellar abnormalities were seen in 95% of fetuses at any stage of gestation, although the banana sign was more typical at <24 weeks, and apparent cerebellar absence was typical of fetuses at >24 weeks. Because the lemon sign is due to decreased intracranial pressure because of caudal herniation of the hindbrain contents, lack of the lemon sign may be due to skull maturation. Alternatively, the cerebroventriculomegaly that is very common in open spina bifida may compensate for the loss of the brain mass. This may displace the skull bones.
More recently, Ball et al. (1993) demonstrated that the lemon sign is not specific for myelomeningocele (Figure 19-1B). In this report of 23 cases of a positive lemon sign, 12 were associated with an open spina bifida, and 6 were seen in cases of encephalocele (see Chapter 12). An additional 5 fetuses did not have a neural tube defect, although they had a variety of other abnormalities, including thanatophoric dysplasia, cystic hygroma, and agenesis of the corpus callosum. An additional CNS finding associated with myelomeningocele was effacement of the cisterna magna, which was seen in 19 of 20 fetuses studied with myelomeningocele (Goldstein et al., 1989). In a review of 234 fetuses with open spina bifida diagnosed at <24 weeks of gestation, Watson et al. (1991) demonstrated that all but two fetuses had at least one of the cranial abnormalities described for affected fetuses. They also questioned whether there was a higher positive predictive value for open spina bifida when more than one sign was observed antenatally. These authors also cautioned that evaluation of motor function in the fetus was not predictive of future neuromuscular status.
A common accompanying intracranial finding in myelomeningocele (MMC) is lateral ventriculomegaly, in which the atrial measurements of the posterior horns exceed 1 cm. In the second trimester, the biparietal diameter and head circumference may be below the 5% for gestational age (Shaer et al., 2007). When MMC is diagnosed between 16 and 24 weeks, microcephaly has been observed in up to 69% of fetuses (Campbell et al., 1987; Thiagarajah et al., 1990). As pregnancy progresses, both biparietal diameter and head circumference tend to normalize in late second trimester. Hydrocephalus, present in 75% of cases, tends to progress slowly during the third trimester (Shaer et al., 2007).
As stated in Chapter 88, evaluation of the fetal spine depends on the visualization of the three ossification centers within the fetal vertebra. The centers of the neural arches should be parallel or converging. In the longitudinal plane, the spine should appear like a “railroad track,” with gradual widening toward the fetal head and tapering toward the sacrum. However, the distal part of the spine may not be ossified in healthy fetuses at <22 weeks of gestation (Budorick et al., 1995). Spina bifida can be demonstrated in both the coronal and transverse planes. In the coronal plane, widening of the ossification centers in the neural arch interrupts the normal parallel configuration of the vertebral arches (Figure 19-3A). In the transverse plane, ossification centers in the neural arch either diverge or take on a U-shaped configuration (Figure 19-4). The presence of scoliosis or kyphosis is associated with neural tube defects (Figure 19-3B).
Kollias et al. (1992) assessed the sonographic accuracy of the estimation of spinal level involved in the MMC. Of 28 cases studied, sonographic and pathologic levels were in agreement in 18 (64%) and within one spinal level in 22 (79%).
Other sonographic findings that may suggest a MMC include a cystic meningeal sac, which may have a shimmering effect with fetal motion (Figure 19-5) (Budorick et al., 1995). The sonographer should also examine the fetus’s lower extremities for the possibility of clubfeet.
With the presence of skin covering the neural tube defect, the lesion is considered to be a closed neural tube defect, such as lipomyelomeningocele, which has a different etiology. Closed neural tube defects are not usually associated with Arnold–Chiari II malformations and they have a much more favorable prognosis (Tortori-Donati et al., 2000; Ramin et al., 2002).
The adjunctive use of magnetic resonance imaging (MRI) of the fetus has provided additional and complementary information to ultrasound examination alone (Figure 19-6). The results of at least two studies suggest that fetal MRI is superior to ultrasound examination for prenatal diagnosis of the intracranial abnormalities associated with MMC (Dinh et al., 1990; Levine et al., 1999). In a comparison of sonography and MRI, both were equally accurate in assignment of MMC level (Aaronson et al., 2003). MRI may be a particularly helpful adjunct to ultrasound examination when there is a large maternal body habitus, oligohydramnios, low position of fetal head, or posterior position of fetal spine present (Glenn and Barkovich, 2006).
DIFFERENTIAL DIAGNOSIS
The differential diagnosis for MMC includes isolated hemivertebrae (see Chapter 88). The lemon sign, as stated earlier, has also been seen in encephalocele (see Chapter 12), thanatophoric dysplasia (see Chapter 90), cystic hygroma (see Chapter 31), and craniosynostosis (see Chapter 10 and Figure 19-1B). The demonstration of a mass near the fetal sacrum should also suggest a possible diagnosis of sacrococcygeal teratoma (see Chapter 115). Sacrococcygeal teratomas are large cystic or solid masses arising from the coccyx. These masses may be associated with fetal hydrops or polyhydramnios. If the fetal sacral bones cannot be visualized, considerations in the differential diagnosis also include the caudal regression syndrome and sirenomelia (see Chapter 87).
ANTENATAL NATURAL HISTORY
With the presence of an open neural tube defect there is increased in utero lethality. For example, at 8 weeks of gestation, one fourth of all conceptuses with neural tube defects will be liveborn, one-fourth will be stillborn, and one half will spontaneously abort (Main and Mennuti, 1986).
The formation of the neural tube begins at approximately day 19 with formation of the primitive streak. Epiblasts transform to ectoderm along the dorsal midline of the embryo. This gives rise to the neural plate, which then enfolds to form the neural groove. In the middle of the 4th week, neural folds on each side of the neural groove begin to fuse, thus forming the neural tube (Rieder, 1994). Current evidence suggests that there are two parallel processes that occur. At the level of the fifth somite, where the brain and spinal cord meet, the normal folds join in a zipperlike fashion that proceeds cranially and candally (Shaer et al., 2007). A second closure site appears in the forebrain; fusion also occurs at that site in two directions, and meets the zipper process proceeding from the hindbrain. In parallel, the zipper process moves to close the most rostral part of the forebrain. Lack of signaling between the neural tissue and overlying ectoderm and mesoderm may result in the bony defect that overlie the unfused sections of the neural tube (O’Rahily and Muller, 2002).
More than 80% of children with a neural tube defect can be detected by maternal serum AFP screening before birth (Brock and Sutcliffe, 1972). Although the determination of amniotic fluid acetylcholinesterase can be helpful, ultrasound examination is the method of choice for the diagnosis of neural tube defects. Direct visualization of the fetal spine can usually be accomplished by 16 weeks’ gestation. Since the 1980s, the sonographic diagnosis of MMC has been enhanced by the introduction of high-resolution imaging tools and by recognition of specific brain abnormalities (Blumenfeld et al., 1993). First described by Arnold and Chiari at the end of the 19th century (Arnold, 1894; Chiari, 1895), the Arnold–Chiari II malformation is defined as the maldevelopment of a small posterior fossa and the herniation of the cerebellar vermis and brainstem (including the fourth ventricle) through an enlarged foramen magnum. Additionally, agenesis of the corpus callosum, enlargement of the massa intermedia, cortical heterotopia, and polymicrogyria can be seen. The origin of the Arnold–Chiari II malformation remains in dispute. The predominant hypothesis maintains that an imbalance of hydrodynamic forces occurs secondary to loss of CSF from the lesion (Padget, 1968; McLone and Knepper, 1989; Paek et al., 2000; Bouchard et al., 2003). An alternative theory interprets the hindbrain herniation as the consequence of a traction injury caused by cranial growth imbalance (Penfield and Coburn, 1938; Lichtenstein, 1942; Hoffman et al., 1975; McLone and Knepper, 1989). Overgrowth of the cerebellum and brainstem, along with a posterior fossa that is smaller than normal, leads to downward dislodgment of these structures, resulting in Arnold–Chiari II malformation (Barry et al., 1957). Despite the controversies about the origin of MMC-associated hindbrain herniation, these lesions are identifiable in the embryo from as early as 8 weeks of gestation and are established in the fetus by the 12th week.
Pathologic studies of human embryos and fetuses with MMC in earlier stages of gestation reveal an open neural tube but undamaged neural tissue with almost normal cytoarchitecture (Patten, 1953). This suggests that neural degeneration occurs at some point later in gestation (the “two-hit” hypothesis) (Ehlers et al., 1992; Hutchins et al., 1996). The first “hit” is the failure of neurulation early in gestation. The second “hit” is the spinal cord injury resulting from prolonged exposure of the neural tissue to the intrauterine environment. In theory, this secondary event can be prevented if an adequate prenatal covering of the exposed neural tube can be provided. To have the best outcome, this repair must be fashioned before the onset of irreversible neural damage. There are several observations in human embryos, fetuses, and infants to support this premise (Neumann et al., 1994; Meuli et al., 1997).
In a pathologic examination of spinal cords of stillborn human fetuses with MMC (19–25 weeks of gestation), varying degrees of neural tissue loss at the site of the lesion were observed, but the dorsal and ventral horns were normal proximal to the defect (Hutchins et al., 1996). This group was among the first to suggest the two-hit hypothesis because they attributed these alterations to injuries occurring subsequent to the failure of primary neural tube formation. A study of 10 additional fetuses had similar findings (Meuli et al., 1997). Additional support exists for the two-hit hypothesis from in vitro studies. Drewek et al. (1997) reported that damage to open neural tissue appears to be progressive and results from exposure to toxic substances in the amniotic fluid during the third trimester.
Korenromp et al. (1986) observed that fetuses with MMC exhibited leg movement at 16 to 17 weeks. These investigators suggested that the affected fetuses did have good function at that point in gestation. No follow-up could be reported in this series because pregnancies were interrupted. Furthermore, Sival et al. (1997) compared the leg movements of 13 fetuses with MMC prenatally and postnatally. Only one of the 13 had abnormal leg movements before birth, but 11 demonstrated abnormal leg movements postnatally. Two possible explanations for this phenomenon exist. The prenatal leg movements could be secondary to spinal cord reflex rather than of cerebral origin, thus permitting motion without electrical impulses conducted through the damaged spinal cord tissue. In addition, leg movements early in pregnancy could result from cerebral function conducted through an exposed spinal cord that is not yet damaged. However, even extremely experienced sonographers find it difficult to distinguish between spontaneous and reflex-based fetal leg movements (Filly, 1994).
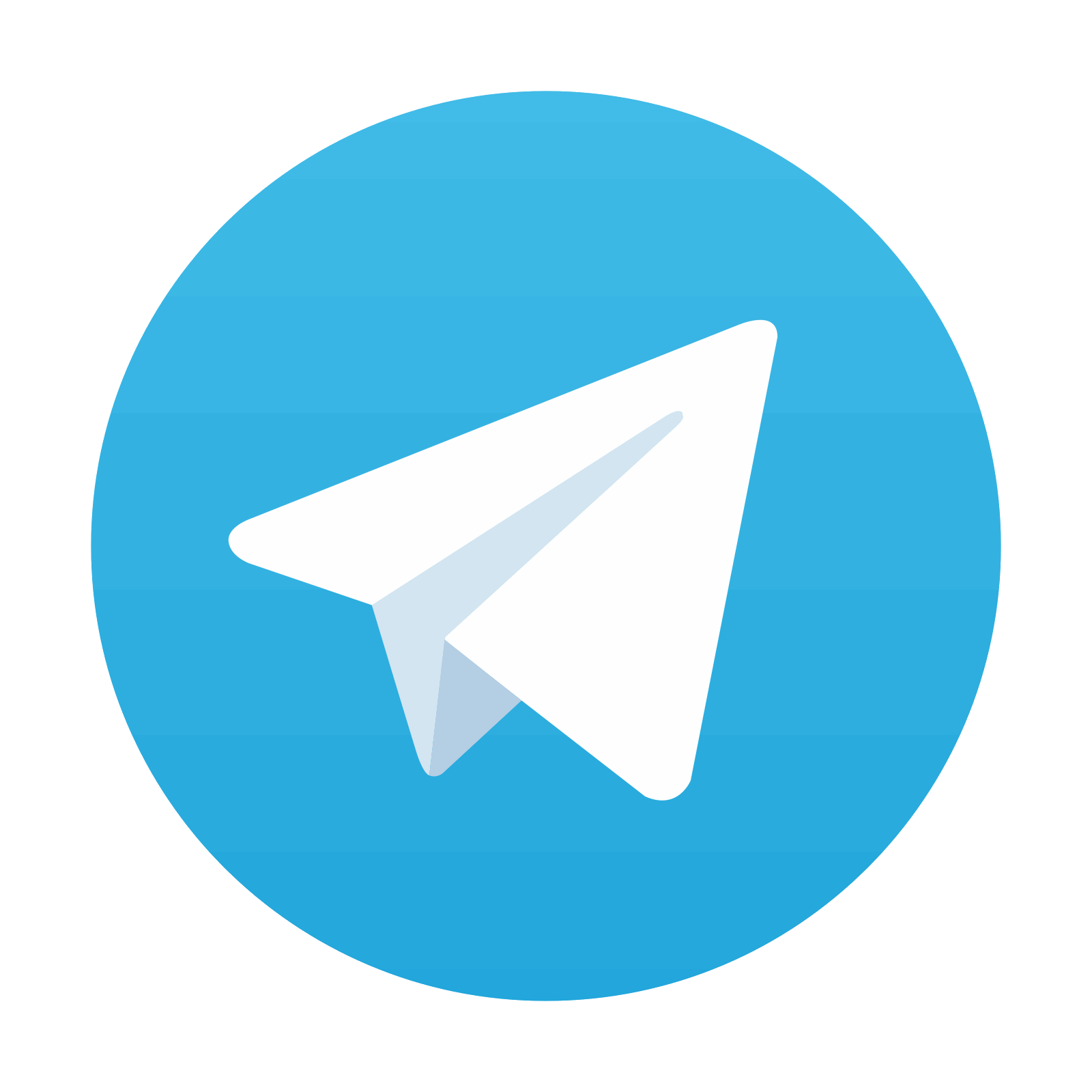
Stay updated, free articles. Join our Telegram channel

Full access? Get Clinical Tree
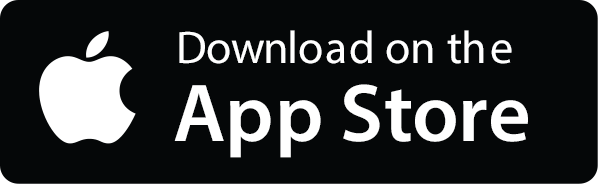
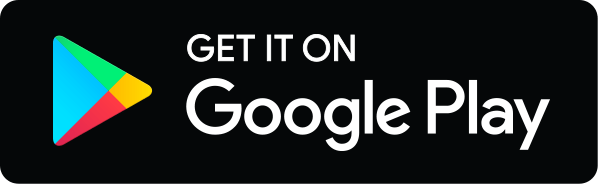