Over the past several decades, invasive fetal therapy has evolved from an appealing concept to a dedicated field of medicine that bridges the disciplines of obstetrics, pediatrics, anesthesiology, and surgery. Since description of the first case of fetal therapy by Liley in 1963 involving transfusion of a hydropic fetus affected by Rhesus alloimmunization, advances in prenatal diagnosis and surgical innovation have resulted in breakthroughs in the management of congenital anomalies or conditions historically considered to be lethal or severely life-limiting.1 The advent of prenatal ultrasonography and video fetoscopy, the refinement of needle-based procedural techniques, and innovations in anesthesia and surgical practices have each been critical to the arrival of modern fetal therapy.
The first published guidelines establishing suitable candidacy for fetal therapy were promoted by the group that would later become the International Fetal Medicine and Surgery Society (IFMSS)2,3 (Box 28-1). These recommendations, which remain relevant to this day, require a certain and severe fetal diagnosis amenable to prenatal intervention, a multidisciplinary approach to care, and an intent that maternal risk be minimized with all fetal therapy undertakings.
Box 28-1 INTERNATIONAL FETAL MEDICINE AND SURGERY SOCIETY GUIDELINES FOR CONSIDERING FETAL THERAPY (AS SUMMARIZED BY CHESCHEIR)2,3
The disorder must be of a significant nature and should be a simple structural defect that interferes with organ development whose alleviation might allow for fetal development to proceed normally.
The diagnosis must be complete and accurate, such that patients whose fetus is so severely affected that intervention is futile as well as those whose fetus is so mildly affected that postnatal treatment would be effected are equally excluded; the defect should be isolated, with a normal karyotype and absent other anomalies.
The fetus should be a singleton without concomitant anomalies according to Level II ultrasonographic examination and amniocentesis for karyotype, amniotic fluid alpha fetoprotein, and viral cultures.
An animal model for the disorder and intervention should exist.
There should be access to a Level III high-risk obstetrics unit, an intensive care unit, and bioethical and psychosocial counseling.
A multidisciplinary team, including a perinatal obstetrician experienced in fetal diagnosis and intrauterine transfusion, an ultrasonographer experienced in the diagnosis of fetal anomalies, and a pediatric surgeon and neonatologist who will manage the infant after birth, should concur on the plan for innovative treatment and obtain the approval of an institutional review board.
The family should be fully counseled about risks and benefits and should agree to treatment, including long-term follow-up to determine efficacy.
Maternal risks should be minor and acceptable.
As is the case with all medical innovation, a major challenge is to ensure that fetal therapies are both ethically justifiable and scientifically validated prior to acceptance as standard of care.4 Scientific validation is a particular challenge with invasive fetal therapy, as the overall rarity of conditions that might be suitable for fetal therapy has limited the production of high-quality prospective data to support practices that are largely backed by retrospective series and clinical wisdom.5 Notable exceptions to this include well-conducted randomized trials demonstrating benefit to fetoscopic laser therapy for treatment of twin-twin transfusion syndrome (TTTS) and open fetal surgery for myelomeningocele (MMC) repair.6,7
This chapter is intended to provide detailed information on selected invasive procedures that may be offered at contemporary fetal therapy centers. Due to space constraints, it does not promise to be an exhaustive summary of all existing fetal therapies. In fact, many less commonly performed invasive procedures have been intentionally omitted from this review. Furthermore, some invasive procedures are covered elsewhere in this text, such as cordocentesis for fetal blood transfusion or multifetal pregnancy reduction. Medical treatments, such as those for fetal cardiac arrhythmias and alloimmune thrombocytopenia, are also not considered in this chapter.
This chapter also does not provide detailed set-up or explicit step-by-step instructions for performing invasive fetal therapies. Rather, it is meant to serve as a primer to assist the clinician in the identification, evaluation, and initial counseling of potential candidates for fetal therapy prior to timely referral to established therapy centers for further counseling and care. This chapter includes a description of selected minimally invasive therapies followed by an introduction to open fetal surgery.
TREATMENT OF TWIN-TWIN TRANSFUSION SYNDROME: FETOSCOPIC LASER PHOTOCOAGULATION AND AMNIOREDUCTION
TTTS is a severe complication involving an unbalanced shunting of blood between monochorionic twins across the fetal surface of their shared placental mass.8 The condition impacts 10% to 15% of monochorionic, diamniotic (MCDA) twins and involves uncompensated unidirectional flow through pathogenic arterio-venous anastomoses that connect fetal circulations. Absent intervention, early-onset, advanced-stage TTTS has a perinatal mortality rate that is in excess of 70%, and survivors face substantial risk for profound neurological disability.9
TTTS is an ultrasound-based diagnosis dependent upon the essential requirements of donor-twin oligohydramnios (defined as maximum vertical pocket [MVP] under 2 cm) and recipient-twin polyhydramnios (defined as MVP over 8 cm). A widely accepted staging system described by Quintero exists for TTTS in which sonographic findings are used to identify evidence of donor or recipient in utero deterioration (Box 28-2).10 While the Quintero staging system is imprecise at predicting disease course on a case-by-case basis, advancing disease severity is generally associated with lower rates of disease stability or regression and overall poorer prognosis without therapy.11 The Quintero staging system importantly allows for standardized communications among providers, comparisons between research studies, and identification of appropriate candidates for fetal therapy.
Box 28-2 QUINTERO STAGING SYSTEM FOR TWIN-TWIN TRANSFUSION SYNDROME10
Stage I: Polyhydramnios-oligohydramnios sequence (POS), defined as a maximum vertical pocket of fluid greater than 8 cm for the recipient twin and less than 2 cm for the donor, as well as visible urine-filled bladders and normal Doppler studies.
Stage II: POS and the bladder of the donor twin is not visible (during the length of the examination, usually 1 hour), without critically abnormal Doppler studies.
Stage III: POS and Doppler studies are critically abnormal in either twin and are characterized as absent or reversed end-diastolic velocity in the umbilical artery, reversed flow in the a-wave of the ductus venosus, or pulsatile umbilical venous flow.
Stage IV: POS accompanied by the presence of ascites, pericardial or pleural effusion, scalp edema, or overt hydrops.
Stage V: Death of one or both twins.
Until this past decade, the historic mainstay of therapy for TTTS had been therapeutic amnioreduction. Amnioreduction is a temporizing measure in which amniotic fluid is removed from the recipient sac under continuous sonographic guidance, usually with an 18- or 20-gauge needle, until a normal fluid volume is achieved. In addition to the management of TTTS, amnioreduction is also utilized for other pathological conditions leading to extreme polyhydramnios that are not covered in this chapter.
Amnioreduction for TTTS is believed to reduce natural complications attributable to polyhydramnios (such as preterm labor, PPROM, and preterm birth), thereby propagating the pregnancy to a more advanced gestational age at delivery, and it may also transiently improve placental blood flow by reducing pressure within the recipient twin sac. As amnioreduction does not interrupt the underlying vascular shunting between twins, the expectation is that recipient twin polyhydramnios will recur and serial amnioreductions will be required, each procedure with its own risk for complications. However, in a subset of patients, a single amnioreduction has been reported to arrest the TTTS process, and this is referred to as the “amnioreduction paradox.”12 The “amnioreduction paradox” has never been prospectively validated, so it is unclear if this is a true phenomenon. It is alternatively possible that this “paradox” actually represents the unpredictability in individual disease courses, such that some cases of TTTS—and especially early-stage disease—may regress without any therapy at all.
While published case series on amnioreduction have reported a wide range of survival rates from 33% to 83%, a large international registry involving 223 TTTS pairs undergoing amnioreduction demonstrated a 78% live birth rate and 60% rate of survival to 4 weeks of life.13 Among survivors to 4 weeks, 74% underwent head ultrasound due to clinical concerns, with roughly one in four exhibiting abnormal findings. Limited data exist regarding long-term neurological outcomes among survivors of TTTS treated with amnioreduction. In a small series of survivors to at least 2 years of age, 3 (7.1%) of 42 children were describing as having severe neurological outcomes (two cases of cerebral palsy, one case of multilocular encephalomalacia). A different survivor reportedly suffered a “brain infarction” that was not otherwise detailed. Notably, neurological diagnoses in this case were obtained by review of records, and standardized neurological testing of each survivor was not performed as part of the study design. Additionally, multiple survivors were reported to possess “mild” speech or motor delays without further clarification.
Given demonstrated superiority of fetoscopic laser photocoagulation over amnioreduction for treatment of TTTS, amnioreduction is now generally restricted in clinical practice to selected clinical presentations. In the United States, these include TTTS presentations beyond 26 weeks of gestation and situations in which timely referral to a laser center is not possible. Amnioreduction is usually discouraged for potential laser therapy candidates, as potential procedure-related complications such as PPROM, abruption, and amnion-chorion separation may preclude a patient from receiving the superior laser treatment.
Fetoscopic laser photocoagulation is the validated contemporary standard of care for the treatment of early-onset, advanced-stage TTTS.6,14 In most U.S. laser centers, candidacy for therapy involves early-onset, advanced-stage TTTS, defined as Quintero stage II through IV TTTS presenting between 16 and 26 weeks’ gestational age.
Whether laser therapy should be offered for the treatment of stage I TTTS is a source of international controversy and the subject of an ongoing randomized, controlled trial. Due to reports indicating a high rate of disease stability or regression with expectant observation,15 immediate laser therapy may introduce excess harm over a strategy involving close observation and therapy for a change in status. A literature review involving 262 total twin pairs additionally determined that overall survival rates were 77% after amnioreduction, 85% after laser therapy, and 86% with expectant management.16 While many U.S. centers currently do not offer laser therapy for unselected early-stage TTTS presentations, some will consider laser when stage I disease is further complicated by maternal symptomatology, a short cervix, or a patient living a great distance from the treatment center.17
Another controversy involves whether there is a role for laser therapy at extremes of the commonly accepted gestational age treatment window. While one retrospective study observed comparable outcomes between “early” (<17 weeks), “late” (>26 weeks), and “conventional” treatment groups,18 many U.S. centers continue to adhere to established gestational age ranges with rare exceptions.
A criticism of the Quintero system is that it fails to consider the recipient twin within advanced-stage TTTS until severe pathology is present in the form of overt heart failure (ie, hydrops) or critical Doppler velocimetry findings within the ductus venosus. Echocardiography-based systems have been developed to detect recipient-twin cardiomyopathy, and some centers use these systems to effectively “up-stage” potential candidates with early-stage TTTS and recipient twin acquired cardiac dysfunction for therapy.19,20
Laser therapy utilizes operative fetoscopic equipment, which is essentially laparoscopic equipment that has been modified to be long (to maneuver within large polyhydramniotic sacs) and narrow (to minimize risk of invasive complications such as PPROM).21 The procedure can be performed under a range of anesthesia types, from local to general. Treatment involves percutaneously inserting a 10-Fr or 12-Fr cannula under sonographic guidance into the recipient twin sac in order to maximize exposure to the placental surface. A 0-degree or 30-degree fetoscope paired with laser fiber in an operative sheath is then advanced through the cannula, enabling visualization of the twin placental cord insertions and chorionic plate. This allows for “mapping” of the placental surface vasculature, in which inter-twin anastomoses are identified and the vascular equator (the rough line along which pathological anastomoses exist) is determined.
Using a laser optimized for absorption by hemoglobin set to 20 to 60 watts, anastomoses are selectively photocoagulated (Figure 28-1). Photocoagulation intends to “dichorionize” the previously shared placental mass, thereby interrupting the TTTS pathophysiology (Figure 28-2). Suitable laser sources include diode, neodymium:yttrium-aluminum-garnet, and potassium-titanyl-phosphate.
Selective photocoagulation refers to limiting photocoagulation to intertwin anastomoses demonstrated upon placental mapping.22 In some centers, sequential selective photocoagulation is practiced, in which anastomoses are ordered for photocoagulation based upon type (ie, arteriovenous, venovenous, or arterioarterial) and directionality (ie, donor-to-recipient before recipient-to-donor); however, benefits of this technique require further study.23 Other centers methodically advance along the vascular equator, photocoagulating from one anastomosis to the next, with a goal to minimize total photocoagulation time. The rationale for this technique is that hemodynamic imbalances may occur during photocoagulation, and so more rapid completion of therapy may minimize complications.
Following photocoagulation, the Solomon technique of equatorial dichorionization may be performed.24 This technique involves photocoagulation along the entirety of the vascular equator from one anastomosis to the next in order to occlude subvisible anastomoses (some under 1 mm in diameter) that might result in the posttherapy complications of twin anemia polycythemia sequence (TAPS) or other recurrent fetofetal transfusion such as reversed TTTS. TAPS is generally a slower, compensated form of fetofetal transfusion that can occur spontaneously or after laser therapy, and is associated with twin morbidity and mortality.25,26 TAPS has been reported in 2% to 13% of TTTS pregnancies after laser.
Prior to completion of the procedure, a therapeutic amnioreduction is generally performed to reduce recipient twin fluid volume to a normal range. As fetoscopy and amnioreduction may each cause uterine contractions, tocolysis may be considered in the perioperative period to maintain or achieve uterine quiescence, with commonly utilized medications including indomethacin and nifedipine. One case-control study indicated that nifedipine administration was associated with a significant increase in recipient twin survival, suggesting a direct fetal cardiovascular benefit.27
Antibiotic prophylaxis is also utilized around the time of all fetoscopic procedures, although the type and route may vary by center. Intravenous antibiotics are often administered prior to therapy, and intraamniotic antibiotics are additionally utilized in some centers prior to the completion of the procedure. Commonly used antibiotic options include nafcillin and cephalosporins. There are no data demonstrating superiority of any given route of administration or antibiotic type. Antibiotics may be continued for a limited period postoperatively, as well.
Complications of laser therapy include but are not limited to: PPROM, previable loss, preterm labor, preterm delivery, abruption, infection, iatrogenic septostomy, fetal injury, and death of one or both twins. There are rare risks of severe maternal morbidities such as bleeding requiring transfusion, internal injury, and exploratory laparotomy. In a subset of cases, treatment is unsuccessful, and repeat laser may be recommended.
The first reported series of TTTS pregnancies treated with laser photocoagulation was reported by De Lia and colleagues in 1990.28 Their initial technique involved a 10 cm laparotomy and small hysterotomy prior to cannulation of the exposed fetal membranes. Percutaneous video fetoscopic approaches were developed over the subsequent years, and early, uncontrolled single-center experiences delivered promising results.29,30
The Eurofetus randomized controlled trial by Senat and colleagues was the landmark study that demonstrated superiority of laser photocoagulation over amnioreduction for the treatment of TTTS.6 In this trial, participating women with MCDA twin pregnancies complicated by TTTS between 15 and 26 weeks of gestation were randomized to either laser therapy or amnioreduction. Primary outcome measures included perinatal survival of at least one twin and survival of at least one twin to at least 6 months of age. Although the authors had planned to recruit 172 women in each group, the study was discontinued at the second planned interim analysis after only 142 pregnancies (72 laser cases, 70 amnioreduction cases) had been randomized due to a significant survival advantage observed in the laser group. Overall, the trial demonstrated a greater probability of survival of at least one twin to 28 days of life (76% vs 56%, p = 0.009) and survival of at least one twin to 6 months of age (76% vs 51%, p = 0.002).
Neurological outcomes in the Eurofetus trial also favored laser therapy, including a decreased rate of cystic periventricular leukomalacia upon neuroimaging (6% vs 14%, p = 0.02). Furthermore, although the average gestational age at randomization was no different between groups (20.6 weeks laser group, 20.9 weeks amnioreduction group), the median gestational age at delivery after laser was 4 weeks greater than after amnioreduction (33.3 vs 29.0 weeks, p = 0.004). This dramatic difference between groups becomes more striking when considered within the context of subsequent data suggesting prematurity as a major risk factor for postlaser neurological morbidity.31,32
Another randomized, multicenter trial of laser therapy versus amnioreduction for the treatment of severe TTTS funded by the National Institute of Child Health and Development was also prematurely terminated after 42 subjects were randomized.33 Study discontinuation was reportedly at the request of the investigators due to poor recruitment. Analysis of the limited cohort indicated that increased recipient fetal mortality in the laser arm was offset by recipient neonatal mortality in the amnioreduction arm, such that there was no statistically significant observed difference in 30-day postnatal survival between groups.34
Multiple single-center experiences published over the last decade document postlaser survival of one or two twins ranging from 74% to 91% of cases, with overall perinatal survival rates of 57% to 85%.23,35-47 These ranges notably exclude reported experiences from the developing world, in which survival rates tended to be lower over the same time period.48,50
Regarding neurological outcomes, long-term follow-up data indicate that while laser likely offers a benefit when compared to expectant management, there are still substantial risks for long-term neurological morbidity. In single-center experiences in which standardized neurological testing was performed, rates of major neurological morbidity among infant laser survivors ranged from roughly 4% to 18% (Table 28-1).51-58 When considering both minor and major disabilities, neurologically intact children ranged from 78% to 89% in series that provided these data.
Study | Pediatric Subjects | Participation Rate# | Type of Neurologic Testing | Pediatric Age(s) at Testing | Major Neurodevelopmental Disability Rate |
---|---|---|---|---|---|
Banek et al, ‘0352 | 89 | 100% (89/89) | Standardized physical and neurological examinations (all children); 75 (84.2%) children underwent GMDS; 14 (15.7%) underwent SONVIT | GDMS median age 21 months; SONVIT median age 34 months | 11% |
Graef et al, ‘0653 | 167 | 98.2% (167/170) | Standardized physical and neurological examinations (all children); 40 (24%) children underwent GMDS; 112 (67.1%) underwent SONVIT | Median age 38 months (range 14-53 months); GDMS median age 21.5 months; SONVIT median age 39 months | 6% |
Lenclen et al, ‘0931 | 88 | Not specifically reported for laser subgroup | General clinical and standardized neurological examination; ASQ | 2 years corrected for prematurity by study design% | 4.6%$ |
Lopriore et al, ‘0955 | 278 | 94.2% (278/295) | Physical and neurological examination; BSID | 2 years corrected for prematurity | 18% |
Salomon et al, ‘1057 | 69 | 94.5% (69/73) | Questionnaires at 1 and 2 years; standardized physical and neurological examinations; parental ASQ; at age 6, WISC-IV and Draw-a-Man test | Testing through age 6 years | 13% |
Gray et al, ‘1156 | 113 | 97.4% (113/116) | Physical and neurological examination; GMDS, BSID | At least 2 years corrected for prematurity | 12.4% |
Vanderbilt et al, ‘1432 | 100 | 48.5% (100/206) | Physical examination; BDI-2 | 2 years corrected for gestational age at birth | 4% |
The aforementioned Solomon technique involving photocoagulation along the entire vascular equator after “standard” laser therapy has recently been validated as potentially useful in an open-label randomized controlled trial.24 In this trial of 274 women randomized to either Solomon technique (n = 139) or standard therapy (n = 135), the Solomon technique was associated with reduced rates of TAPS or recurrent TTTS (4% vs 21%). There were no differences in procedural complications, perinatal mortality, or severe neonatal morbidity between groups. In a secondary study evaluating the presence of residual placental anastomoses, there were significantly fewer placentas with residual anastomoses in the Solomon group (19% vs 34%, p = 0.04). Neurological outcomes at 2 years in children from the Solomon trial have since been determined to be no different between groups, with survival without neurodevelopmental impairment present in 67% of the Solomon group and 68% of the standard therapy group (p = 0.92). Taken together, these data suggest that due to associated reductions in significant short-term complications of laser therapy, it is advisable to perform the Solomon technique when technically feasible. However, given that the technique does not clearly influence the most meaningful outcomes of survival and long-term neurological health, there are insufficient data with which to conclude that this technique must be performed invariably with each laser procedure.
Fetoscopic laser therapy has also been applied to the management of monochorionic twin pregnancies complicated by unequal placental sharing (UPS) with selective intrauterine growth restriction (sIUGR), although this practice is of unproven benefit. In a retrospective series involving 18 cases of UPS with sIUGR and critical Doppler studies undergoing laser compared to 31 pregnancies that were expectantly managed, Gratacos and colleagues observed significant technical difficulties in completing the procedure.59 In 11.1% (2/18) of cases, the procedure could not be performed, and in an additional 11.1% a second procedure was required due to incomplete photocoagulation of anastomoses. When compared to the expectantly managed group, the laser group had significantly decreased sIUGR twin survival (33.3% vs 80.6%, p = 0.001) and overall perinatal twin survival (63.9% vs 85.5%, p = 0.02). However, although not significantly different between groups, periventricular leukomalacia was 14.3% in the expectant management group versus 5.9% in the laser group, perhaps suggesting a neuroprotective benefit to photocoagulation that this study lacked power to appreciate.
Other applications of fetoscopy include fetoscopic tracheal occlusion (FETO) for severe congenital diaphragmatic hernia (CDH) presentations, as well as fetoscopy for amniotic band release. FETO involves occlusion of the fetal trachea with a fetoscopically placed balloon in order to prevent the efflux of lung fluids, which is believed to stimulate lung growth.60,61 In a metaanalysis analyzing survival after FETO for severe CDH cases with lung-to-head ratio of 1.0 or less and liver herniation, FETO favored survival outcome when compared to untreated controls (odds ratio 13.3; 95% confidence interval 5.4-32.9).61 Among isolated CDH, survival ranged from 35% to 52% across five included publications, as compared to 0% to 9.3% among controls.
One of the included studies within the meta-analysis was a small randomized, controlled trial by Ruano and colleagues, in which 41 subjects were randomized (20 FETO, 21 standard postnatal care).62 Although the FETO group delivered at an earlier average gestational age (35.6 vs 37.4 weeks, p <0.01), there was a higher survival rate after FETO (50% vs 5%, p <0.01). However, lower-than-anticipated survival rates in both groups and small study size limit the strength of conclusions that can be formed upon this trial. An international randomized trial is now underway attempting to evaluate the role of FETO in the management of severe fetal CDH.
Regarding fetoscopic amniotic band release, a recent single-center case series and review of the literature identified just 14 published cases deemed suitable for analysis. While preserved limb function of the affected limb was reported in 50% of cases, therapy was associated with a 57% rate of PPROM. Further study is required to better understand this practice.
CORD OCCLUSION FOR SELECTIVE FETICIDE IN COMPLICATED MONOCHORIONIC MULTIPLE GESTATIONS
Due to their shared placental mass, monochorionic multiple gestations are at risk for severe complications such as twin reversed arterial perfusion (TRAP) (Figure 28-3), discordant anomalies, UPS with sIUGR, and TTTS. Even when one twin within a monochorionic pair is disproportionately impacted by such pathology, the issue threatens the health of both fetuses: Complicated monochorionic multiples are at increased risk for prematurity, and furthermore if one twin (presumably the more severely affected fetus) experiences an in utero demise then this presents risk to its cotwin of death (12%) or survival with profound neurological injury (18%) as a result of transient hemodynamic instability within their shared placental mass.63 Therefore, as a voluntary and utilitarian decision, a patient may request selective feticide within a complicated monochorionic twin gestation in order to terminate the more severely affected fetus and maximize perinatal outcomes for the nontargeted fetus.
Due to vascular anastomoses linking twin circulations within nearly all monochorionic placentas, standard methods to achieve selective feticide within dichorionic twins are considered inappropriate. Intracardiac potassium chloride (KCl) instillation to cause a monochorionic twin feticide could theoretically transfer a bolus of this cardioplegic agent to the nontargeted co-twin via placental anastomoses, resulting in injury or death.64 Furthermore, selective feticide of a monochorionic twin by conventional methods would do nothing to limit the aforementioned risks of co-twin death or severe injury resulting from transient hemodynamic instability within a shared placental circulation.
As a result of these concerns, methods intended to achieve selective feticide within monochorionic multiple gestations involve targeted twin cord occlusion strategies. Reported techniques include chemical occlusion (using sclerosing agents such as alcohol or encubilate gel), mechanical coil placement, mechanical cord occlusion via suture ligation, and various minimally-invasive fetoscopic and ultrasound-guided energy-based methods, including bipolar coagulation, radiofrequency ablation (RFA), and laser therapy.65 High-intensity focused ultrasound has most recently been described in case reports as an intriguing, ostensibly noninvasive modality for fetal cord occlusion.66,67
Sclerosing agents and mechanical coils have been abandoned as cord occlusion strategies due to technical difficulties and low rates of technical success.65,68,69 Mechanical cord occlusion through suture ligation, whether performed fetoscopically or using a single port under ultrasound guidance, is not a popular technique due to technical complexity and substantial risk for iatrogenic complications such as PPROM.70-72 A more recent experience with umbilical cord ligation, with and without transection, for the management of TRAP sequence suggested somewhat improved outcomes (63% survival rate for nontargeted fetus among 32 cases)73; however, even this may not be comparable to other contemporary cord occlusion methods.
Fetoscopic laser therapy to achieve cord occlusion was first described by Ville and colleagues for selective reductions within a series of TRAP presentations.74 Although the initial technique involved photocoagulation of umbilical cord vessels at either the abdominal wall or placental cord insertion site of the targeted fetus, photocoagulation of placental anastomoses has also been described as a suitable alternative in the particular scenario of TRAP sequence.75 In the experience of Hecher and colleagues, this technique was associated with an 80% survival rate at a median gestational age of 37.4 weeks (range 23.7-41.4 weeks) for the “pump” fetus. However, fetoscopy is more invasive than other current methods for cord occlusion, potentially predisposing it to higher rates of PPROM and other complications, and it may be difficult to complete due to technical challenges such as limited access or poor fetoscopic visibility. Furthermore, increasing cord vessel diameters with advancing gestational age may preclude effective photocoagulation, such that many limit this therapy to pregnancies under 20 weeks of gestation.
Perhaps the most commonly employed contemporary techniques for monochorionic twin selective cord occlusion involve bipolar coagulation and RFA. Bipolar uses direct application of bipolar forceps to the target umbilical cord to achieve cord occlusion. The forceps are guided into the uterus through a single percutaneously placed port and the procedure is completed under continuous sonographic guidance. Overall reported experience with bipolar cord occlusion is favorable. Deprest and colleagues described a series of 10 complicated monochorionic twin gestations undergoing bipolar cord occlusion.76 Two cases experience PPROM (resulting in termination) and the remaining eight resulted in live birth of the nontargeted twin, with seven delivering at or beyond 36 weeks’ gestational age. In a series of 17 complicated monochorionic multiple gestations between 18 and 27 weeks of gestation, Nicolini and colleagues observed a 76% survival rate using bipolar, with a majority born at or beyond 34 weeks of gestation.77
RFA utilizes high-frequency radiowaves to cause focal thermal tissue injury. Prior to its application to fetal therapy, it had been used for the treatment of unresectable hepatic tumors and cardiac arrhythmias.78 When used obstetrically, an RFA probe is percutaneously guided under continuous sonographic guidance to an intrafetal cord insertion site (Figure 28-4). High-frequency sinusoidal current (400-500 kHz) is then used to induce focal thermal injury and resultant cord occlusion.79
Although various generators exist for RFA therapy, at Columbia University Medical Center we use the Boston Scientific RF 3000 Generator (Boston Scientific, Natick, MA) and LeVeen needle electrodes (Boston Scientific). The LeVeen probes possess deployable tines in a “palm-tree” configuration allowing for tissue stabilization and a more predictable ablation diameter. Although the initial LeVeen probe was 14-gauge, the most current modification (the SuperSlim LeVeen probe) is 17-gauge, roughly similar to the caliber of needles used in other ultrasound-guided obstetrical procedures. RFA grounding pads also must be applied to the patient’s thighs prior to RFA, as this substantially reduces the risk of thermal injuries reported with early RFA experience.80 Prior to therapy, the patient usually receives antibiotic prophylaxis. While the procedure can be performed under a range of anesthesia types, at our institution we perform the procedure using local lidocaine block after preprocedure Tylenol #3 administration. Uterine irritability is common after therapy, and there is a low threshold for a limited periprocedural course of indomethacin tocolysis.
The most robust RFA outcomes data involve its use for TRAP presentations54,80-82 (Table 28-2). Across four published RFA series, survival of the nontargeted “pump” twin ranged from 80% to 100%, with mean gestational ages ranging from 33 to 37 weeks. The largest experience within this group was the North American Fetal Therapy Network (NAFTNet) retrospective review of 98 patients from 12 centers undergoing RFA for pregnancies complicated by TRAP sequence. Overall, 80% “pump” survival was reported at a median gestational age at delivery of 37 weeks. There was no difference when outcomes were stratified by RFA device manufacturer.
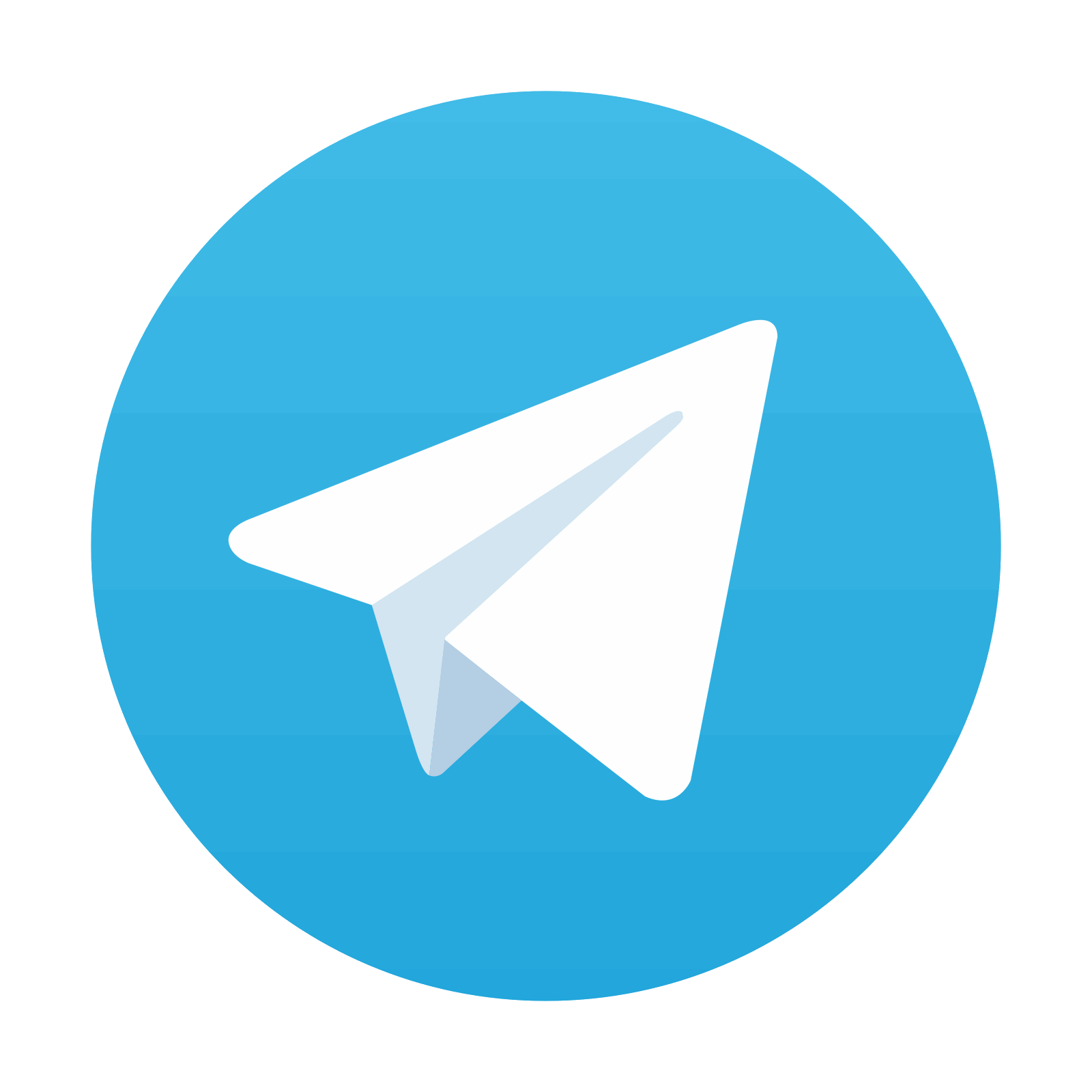
Stay updated, free articles. Join our Telegram channel

Full access? Get Clinical Tree
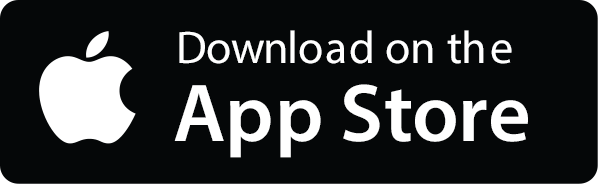
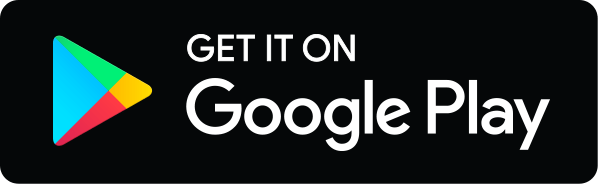