Acute bronchiolitis is the most frequent cause of infant hospitalization in the United States. Thus, a significant portion of a pediatric hospitalist’s time is spent caring for infants with bronchiolitis. Both bronchiolitis and laryngotracheobronchitis (croup) are common and lead to significant morbidity. Epiglottitis, although rare since introduction of the Haemophilus influenzae type B (Hib) vaccine, warrants inclusion because of its life-threatening nature.
Bronchiolitis, a common communicable respiratory illness, is characterized by signs and symptoms of both upper and lower respiratory tract infection. Most episodes occur in otherwise healthy children younger than 2 years. Children younger than 3 months are at high risk for severe disease. Other groups at risk for serious illness secondary to bronchiolitis include young infants less than 37 weeks gestation with and without chronic lung disease, children with hemodynamically significant congenital heart disease, and those with severe immune deficiency such as hematopoietic stem cell transplantation, solid organ transplantation, and cellular immune deficiencies (e.g. 22q11.2 chromosome deletions).
Respiratory syncytial virus (RSV), a single-stranded RNA paramyxovirus, accounts for 50% to 70% of cases of bronchiolitis. Less common causes of bronchiolitis include parainfluenza virus types 1, 2, and 3, influenza viruses A and B, and adenovirus. More recently, human metapneumovirus (hMPV) has been recognized as a cause of bronchiolitis.1,2 hMPV has been detected in 20% to 25% of children with bronchiolitis and negative direct fluorescent antibody testing of nasal aspirates for RSV, parainfluenza types 1 to 3, influenza A and B, and adenovirus.1,3 Rhinovirus appears to be increasing as an etiology of bronchiolitis in older infants.4 Rare causes of bronchiolitis include Mycoplasma pneumoniae and enteroviruses.
Overall, bronchiolitis is most prevalent between October and May, although sporadic cases occur throughout the year. Epidemic RSV bronchiolitis displays remarkable seasonality, with a peak occurrence between December and April and virtually no occurrence between June and October. Peak activity of hMPV occurs in March and April, just as RSV activity begins to wane. The seasonal prevalence of specific pathogens is shown in Figure 101-1. In the United States, 1% to 3% of affected infants require hospital care; most hospital admissions for bronchiolitis occur between December and March.
FIGURE 101-1.
The seasonal prevalence of bronchiolitis caused by specific pathogens. The number of cases represents the mean number of cases per year from 2000 to 2004. RSV, respiratory syncytial virus. (Used with permission of Dr. Richard L. Hodinka, University of South Carolina, School of Medicine, Greenville.)

Although the clinical manifestations of bronchiolitis and asthma overlap to some extent, the pathogenesis of the two conditions differs, a fact that has important therapeutic implications. In bronchiolitis, progressive infection of the respiratory mucosa induces desquamation of ciliated respiratory epithelial cells and lymphocytic infiltration of peribronchial epithelial cells. These changes lead to small-airway obstruction as intraluminal cellular debris accumulates and mucosal edema worsens. The concomitant increase in mucus production and, in some children, enhanced airway smooth muscle reactivity compound the small-airway obstruction that characterizes bronchiolitis. The relatively small caliber of their distal airways predisposes young infants to more severe obstructive symptoms.
Symptoms appear 5 to 7 days after exposure, although the incubation period is often shorter for parainfluenza (2–4 days) and influenza (1–7 days) viruses. The initial symptoms include nasal congestion, rhinorrhea, and cough. Poor feeding, posttussive emesis, and irritability may accompany these symptoms. Fever develops in half to three-fourths of patients. These findings persist for several days, and then lower respiratory tract involvement develops precipitously. Dyspnea and audible wheezing are common. In younger infants, especially those younger than 2 months, apnea may develop as the initial manifestation of bronchiolitis.
At the time of evaluation, tachypnea, tachycardia, and mild to moderate hypoxemia are often present. The infant may appear barrel-chested because of air trapping. Labored breathing, with flaring of the alae nasi, grunting, abdominal breathing, and suprasternal, intercostal, subcostal, and supraclavicular retractions, may be observed. On auscultation, harsh rhonchi, expiratory wheezing, and rales are heard diffusely throughout the chest. As the illness progresses, wheezing is often heard without the aid of a stethoscope. In children with severe lower airway obstruction, wheezing may not be readily appreciated because of poor aeration. Although not common in a tachypneic young infant, marked prolongation of the expiratory respiratory phase occurs with more severe lower airway obstruction.
Other findings in children with bronchiolitis include conjunctivitis, otitis media (upto 50% of cases), and pharyngitis.5,6 The liver and spleen may be palpable because of air trapping and flattening of the diaphragm. Signs of dehydration may be noted in children with decreased fluid intake due to cough or respiratory distress and in those with significant posttussive vomiting.
In older children and adults, infections caused by RSV and hMPV usually cause symptoms of upper respiratory tract involvement such as rhinorrhea, cough, coryza, and pharyngitis. Any of the agents that cause bronchiolitis in younger children can exacerbate asthma or other chronic lung diseases in older children and adults.
In young infants early in the course of illness, the cough is occasionally staccato in nature, which makes it difficult to differentiate clinically from infection with Bordetella pertussis or Chlamydia trachomatis. Asthma, as well as other conditions that cause wheezing (see Chapter 141), must also be considered in the differential diagnosis.7
The diagnosis of bronchiolitis can generally be made by the history and physical examination findings alone. No tests are routinely required.
In the hospital setting, an etiologic diagnosis may facilitate the implementation of strategies to limit nosocomial transmission of respiratory viruses (e.g. type of precautions, patient cohorting). However, institution of these interventions can often be based on the clinical diagnosis (e.g. bronchiolitis) rather than the specific infectious agent.
Rapid viral diagnostic tests are becoming more widely available and have supplanted viral culture of nasal aspirates as the initial diagnostic test for most clinical situations. Types of antigen detection tests include direct and indirect fluorescent antibody tests and “point-of-care” tests. Fluorescent antibody testing can be completed in 2 to 4 hours with sensitivity and specificity ranging from 80% to 90% and 92% to 99%, respectively. The exception is adenovirus detection, for which the sensitivity is 40% to 60%.8,9 The predictive value of antigen detection is high during peak seasons, but false positive results are more likely to occur when disease incidence is low.
More rapid tests, known as point-of-care tests or solid-phase immunoassays, are currently available for influenza viruses A and B and for RSV. In these rapid tests, binding of viral antigen to an antibody is indicated by a color change on the test cartridge. With appropriate facilities and trained personnel, these tests can be completed in an emergency department or office setting in less than 30 minutes. The sensitivity and specificity of these tests vary by manufacturer. Typically, the sensitivity is 75% to 82% and the specificity is 92% to 98%. During the influenza or RSV season, some laboratories initially perform these tests and reserve fluorescent antibody or molecular testing for specimens negative by point-of-care tests.
Molecular assays have become the new gold standard for respiratory virus detection. When compared with viral culture or antigen detection assays, molecular assays using polymerase chain reaction (PCR) offer improved sensitivity and specificity (approaching 100%). These tests must be interpreted in the context of clinical findings, as their improved sensitivity results in detection of viral RNA that persists in the nasal passage for several weeks after shedding of infectious virus has ceased. PCR can be used to detect adenovirus, hMPV, M. pneumoniae, Bortedella pertussis, and enteroviruses in nasal aspirates. Adenovirus and enteroviruses can also often be detected in urine and blood samples of infected patients with signs of systemic illness by PCR. Sending specimens from multiple sites (e.g. nasal aspirate, conjunctival swab, urine, and blood) for testing will increase the yield of the test, but should be reserved for specific cases where the viral etiology must be known.
Chest radiograph findings in bronchiolitis include hyperinflation with flattening of the diaphragm, peribronchial thickening, and patchy infiltrates. Nearly all children with a typical clinical presentation for bronchiolitis have either a normal chest radiograph or these findings consistent with the disease.10 Observed infiltrates are usually due to atelectasis; they occur as a consequence of airway narrowing and mucous plugging. Because radiographic differentiation of atelectasis, pneumonitis, and bacterial pneumonia is challenging, antibiotics are frequently administered after radiography despite typical findings of bronchiolitis on the radiograph.10 Furthermore, studies demonstrate increased antibiotic use after radiography in patients with bronchiolitis without any difference in time to recovery.11 Therefore, current evidence does not support the routine use of chest radiography for infants with clinically suspected bronchiolitis. Chest radiography may be useful in children with severe illness, when there is concern for bacterial pneumonia or other complications (e.g. pneumothorax), when the diagnosis is uncertain, or for children whose disease course is prolonged.12 Concomitant bacterial pneumonia should be suspected in infants with stable (as opposed to migratory) radiographic infiltrates, persistent fever (longer than 2–3 days), and lack of clinical improvement with supportive care only. Some studies suggest that transcutaneous oxygen saturations below 92% may be associated with bacterial pneumonia.10,13 The presence of a pleural effusion also suggests bacterial superinfection.
Infants with poor oral intake may require evaluation for electrolyte abnormalities. Arterial blood gas studies may be useful when there is concern for or evidence of respiratory failure.
The issue of whether children with bronchiolitis merit additional testing to exclude serious bacterial infection (SBI) remains controversial.14 The risk for SBI differs by age group. Infants and children older than 2 months without evidence of lobar pneumonia who are evaluated during the bronchiolitis season and have fever with clear symptoms and signs of bronchiolitis do not routinely require additional testing; the risk for concomitant SBI is low. However, high fever in infants raises suspicion for urinary tract infection (UTI). Febrile uncircumcised boys appear to be at particularly high risk. Risk factors for UTI in infant boys include temperature ≥39°C, fever lasting more than 24 hours, absence of another infectious source, and non-black race. Infant girls are at risk for UTI with temperature ≥39°C, fever lasting 2 days or more, absence of another infectious source, white race, and age less than 12 months.15 A screening urinalysis and urine culture should be considered in children with risk factors present (Chapter 104).16 Clinicians should consider further evaluation, including cultures of blood, urine, and possibly cerebrospinal fluid, in any child with an ill appearance or an atypical clinical course.
Febrile children younger than 2 months with bronchiolitis may be at lower risk for SBI than those without bronchiolitis. However, the risk is not zero. The rate of UTI in particular remains appreciable. Most current studies assessing the risk for SBI in children with bronchiolitis contain important methodologic flaws that limit their generalizability. Levine and colleagues conducted the first prospective multicenter trial in which the risk for SBI was compared in young febrile infants who tested positive for RSV and those who tested negative for RSV. Rates of SBI in the RSV-positive (n = 269) versus the RSV-negative (n = 979) group were as follows: urinary tract infection, 5.4% (95% confidence interval [CI]: 3.0%–8.8%) versus 10.1% (95% CI: 8.3%–12.2%); bacteremia, 1.1% (95% CI: 0.2%–3.2%) versus 2.3% (95% CI: 1.4%–3.4%); and meningitis, 0% (95% CI: 0%–1.2%) versus 0.9% (95% CI: 0.4%–1.7%).16 A systematic review of infants younger than 90 days hospitalized with bronchiolitis found the rate of UTI in this cohort of 3.3% (95% CI: 1.9%–5.7%). No case of bacteremia was found in 8 of 11 studies evaluated, and no case meningitis was reported in any study.17
Generally, bronchiolitis is a self-limited disease, characterized by frequent waxing and waning clinical appearance. Apnea associated with bronchiolitis usually resolves within 48 to 72 hours, although some infants may need longer periods of hospitalization and monitoring. The typical acute bronchiolitis illness lasts 3 to 7 days. Infants hospitalized relatively early in their illness may demonstrate clinical worsening for several days before improving. Improvement is gradual and heralded by decreases in the respiratory rate, retractions, and duration of the expiratory phase. Wheezing generally persists for more than a week after hospital discharge. Oxygen saturation as measured percutaneously by pulse oximeter may hover between 92% and 96% for several weeks. Other symptoms, including cough and noisy breathing, take longer to resolve.18 Anticipatory guidance on the course of illness is essential to help parents understand the expected disease course of bronchiolitis and to give them practical advice on when to seek medical care (Table 101-1).
Clinical Course: Bronchiolitis typically starts with upper respiratory tract symptoms, progressing to lower respiratory tract symptoms after 2 to 3 days. Disease symptoms peak during days 4 to 6. Resolution of symptoms may take 1 to 2 weeks, with approximately 10% of patients having persistent symptoms at 4 weeks. |
Transient Nature: Symptoms in bronchiolitis can change frequently. Children with bronchiolitis may look to have only mild symptoms at one moment, which can be promptly followed by significant distress. When the child appears in moderate-to-severe distress, attempt to calm the child, provide supportive treatment measures, and if significant distress persists, contact the child’s physician. |
Treatment at Home:
|
Indications to return to medical care: apnea (pause in breathing for more than 15 seconds), cyanosis, not able to maintain hydration or poor feeding, fever, increased respiratory rate (more than 60 breaths per minute that persists for more than 15–30 minutes), increased work of breathing (grunting, flaring, retractions) |
Prevention:
|
Mortality is less than 0.1% overall but approaches 5% in those with high-risk conditions, including young age (<2 months), chronic lung disease, cyanotic heart disease, and immune deficiency.
There is great debate in the medical literature concerning the risk of recurrent wheezing and the development of asthma in children with bronchiolitis. The development of asthma after bronchiolitis is multifactorial and likely depends on viral etiology, host susceptibility, illness severity, and timing and frequency of bronchiolitis episodes. Recent studies suggest that rhinovirus plays an important role in the development of recurrent wheeze in asthma, likely more than RSV; however, this role is not yet fully understood.19-21 Current evidence suggests that children with recurrent viral illness and atopic features are at highest risk of developing asthma later in life.22
Pulse oximetry is often used as a surveillance tool for respiratory failure, but it does not detect hypoventilation. Hypoventilation leading to significant hypercapnia results in only modest decreases in oxygen saturation. Recalling the hemoglobin–oxygen dissociation curve, oxygen saturation does not diminish significantly until the partial pressure of oxygen (PO2) falls to the range of 60 mm Hg (i.e. the steep part of the curve) (Figure 101-2). In addition, small amounts of supplemental oxygen will restore PO2, thereby normalizing oxygen saturation, which would allow unrecognized inadequate ventilation to progress without further derangements in pulse oximeter readings. Respiratory acidosis ensues with increasing hypercapnia. Bedside evaluation of an infant with respiratory failure would reveal somnolence and tachypnea with shallow respirations, which can progress to bradypnea or apnea. Expected derangements in arterial blood gas results would progress from hypocapnia associated with tachypnea and hyperventilation to the normal-range partial pressure of carbon dioxide (PCO2). This “normal” PCO2 would be inappropriate in the setting of hyperventilation and should be considered a harbinger of respiratory insufficiency. Progression to respiratory failure would be confirmed by worsening hypercapnia and respiratory acidosis (i.e. falling blood pH on an arterial blood gas specimen).
The primary care physician manages most infants and children with bronchiolitis in the outpatient setting. Certain high-risk children and children with severe symptoms require closer monitoring and often hospitalization. Factors associated with a more severe disease course where hospitalization should be considered include age less than 3 months, prematurity (gestational age <34 weeks), significant retractions, tachypnea, tachycardia, and hypoxia (oxygen saturation ≤94%).12,23-26
The mainstay of treatment in infants and children with bronchiolitis is supportive. Infants and children admitted with bronchiolitis and hypoxia require supplemental oxygen. The appropriate lower limits for oxygen saturation in children with bronchiolitis are not known, but various guidelines suggest values ranging from 90% to 94%. However, no guideline specifies the acceptable duration of desaturation below these values. Furthermore, normal transient desaturation and measurement error because of poor pulse pressure and motion artifact occur frequently. Considerable uncertainty remains over when to begin, increase, or discontinue supplemental oxygen therapy. As a consequence, abnormalities in pulse oximetry readings alone often unnecessarily prolong hospitalization in children with bronchiolitis as a result of the perceived requirement for supplemental oxygen. Healthy infants with bronchiolitis who have oxygen saturations at or above 90% while breathing room air benefit little from increasing PaO2 with supplemental oxygen (Figure 101-2), particularly in the absence of significant respiratory distress and feeding difficulties. Thus the American Academy of Pediatrics bronchiolitis guidelines recommend that supplemental oxygen should be initiated in previously healthy infants only if oxygen saturation values fall persistently below 90%, and should be discontinued if oxygen saturation is greater than 90% while feeding well with minimal respiratory distress.27 Fever, acidosis, and hemoglobinopathies shift the oxyhemoglobin dissociation curve to the right; a higher oxygen saturation should be maintained in patients with these factors. Schroeder and coworkers propose the following strategies to limit overreliance on pulse oximetry:
Discontinue the use of pulse oximetry or transition to intermittent pulse oximetry during the convalescent stage of illness.
Initiate or increase supplemental oxygen therapy only in children with increasing respiratory distress or when other maneuvers such as stimulation, suctioning, or repositioning fail to improve oxygenation.
Establish a hospital-wide consensus on an acceptable level and duration of desaturation.
Increase physician involvement in decisions to initiate and increase oxygen therapy; such decisions are often made independently by nurses or respiratory therapists.28
Other potential respiratory support interventions include heliox therapy, nasal continuous positive airway pressure (CPAP), high-flow oxygen via nasal cannula, and endotracheal intubation with mechanical ventilation. Administration of heliox (70% helium, 30% oxygen) through a non-rebreather (reservoir) facemask may improve respiratory effort by preserving laminar airflow at higher flow rates. However, heliox has not been shown to reduce either the rate of endotracheal intubation or length of ICU stay.29 In small studies, CPAP has been shown to reduce pCO2 and improve clinical respiratory scores.30,31 High-flow nasal cannula (HFNC) has emerged as a respiratory treatment in bronchiolitis due to its ability to wash out nasopharyngeal dead space, allowing for improved oxygenation and ventilation, as well as its ability to provide inspiratory flow to overcome nasopharyngeal resistance, provide positive distending pressure for lung recruitment, improve pulmonary compliance, and reduce metabolic demand.32 Several studies demonstrate that use of HFNC in bronchiolitis decreases intubation, decreases respiratory rate, and decreases ICU length of stay.33-36 Studies suggest that early use of HFNC do not alter the disease course; however, it is unclear if HFNC has a role as a rescue therapy to minimize risk of the need for intensive therapies.37 The most beneficial and cost-effective methods of using HFNC has yet to be determined. Mechanical ventilation should be considered for infants with signs of respiratory failure, shock, or persistent apnea.
Maintaining hydration with intravenous fluids may be necessary, but nasogastric feeding is an alternative. One open randomized trial found that both intravenous and nasograstric fluids were appropriate hydration methods for infants with bronchiolitis, but nasogastric insertion may require fewer attempts and have higher insertion success rates than intravenous access.38 Infusing nasogastric feeding slowly tends to minimize gastric distention and vomiting. Daily weights and careful documentation of intake and output are helpful in assessing and tracking fluid status.
Several meta-analyses have systematically reviewed the efficacy of β-agonists in the treatment of bronchiolitis.39,40 A total of 13 trials consisting of 956 patients were included in the systematic review by King et al.40 Most of the studies compared nebulized albuterol with nebulized saline, unspecified placebo, or no treatment, while four studies also compared nebulized albuterol with nebulized ipratropium bromide. Nine of the 13 trials showed no difference in various clinical measures (e.g. oxygen saturation, respiratory rate) between children treated with and without β-agonist therapy, three trials demonstrated short-term improvement in various clinical measures, and one trial demonstrated a worsening of these parameters. Seven of the 13 trials examined a primary outcome measure related to the need for or length of hospitalization; none reported significant differences between the groups. Hartling and colleagues examined the use of bronchodilators in 48 trials that included a total of 4897 patients. Meta-analysis revealed that β-agonist use was not associated with lower hospitalization rates at 1 and 7 days after presentation to the emergency department or with a shorter hospital length of stay.41 Gadomski and Brower examined 28 trials (1912 infants) and found that β-agonists do not improve oxygen saturation, do not reduce hospitalizations, do not shorten the duration of hospitalization, and do not reduce time to resolution of illness.42 In seven trials that examined effects of β-agonists on respiratory status (365 infants), the clinical score of 64% of those treated with bronchodilators improved compared to 27% of placebo (odds ratio for no improvement: 0.18; 95% CI: 0.06–0.5). The average improvement in clinical score, though statistically significant, was of small magnitude, thus limiting its clinical significance (standardized mean difference: –0.37; 95% CI: –0.62 to –0.13).42 In summary, although some trials of β-agonist therapy demonstrated mild improvement in some clinical features of bronchiolitis, β-agonist use must be weighed carefully against potential adverse effects and cost of these therapies. In addition, β-agonists appear to have no effect on other clinically important factors, such as oxygen saturation, need for hospitalization, duration of illness, and hospital length of stay.
In addition to the bronchodilating effects of β-agonists, nebulized racemic epinephrine adds α-agonist properties that are thought to reduce airway edema and mucus production by inducing vasoconstriction of the bronchial vasculature. Hartling and colleagues performed a meta-analysis of 19 trials involving 2256 infants examining effects of racemic epinephrine in bronchiolitis. Compared to placebo, epinephrine showed a significant reduction in hospitalization at one day after presentation in outpatients (risk ratio [RR]: 0.67; 95% CI: 0.5–0.89), but no difference at 7 days. There was no difference in admission rates when epinephrine was compared to β-agonists. For inpatients, there was no difference in hospital length of stay when comparing epinephrine to placebo, although the mean length of stay was 0.28 (95% CI: 0.46–0.09) days less in those receiving epinephrine compared to β-agonists.43 This analysis also found a statistically significant, though clinically modest, improvement in clinical score at 1 hour after treatment when comparing epinephrine to placebo in outpatients (standardized mean difference: –0.40; 95% CI: –0.58 to –0.23) with no differences in oxygen saturation or respiratory rate. When compared to β-agonists, epinephrine appears to have modest benefits in short-term clinical outcomes in the first 24 hours of care, such as clinical score and respiratory rate.43
Although the benefit of administering bronchodilators has not been established, they are often used in clinical practice, as clinical experience suggests that in selected infants bronchodilators improve the clinical condition. There is currently no evidence-based means of determining which infants may benefit from bronchodilators. Bronchodilators may be considered in patients with severe respiratory distress, hypoxia, or impending respiratory failure. Attempting a trial of nebulized bronchodilator and evaluating clinical response may be considered in patients with moderate to severe bronchiolitis. It is critical to evaluate response to therapy with objective scores before and after the bronchodilator trial. If no significant improvement occurs within 60 minutes of administration, treatment should be discontinued. Although studies suggest that racemic epinephrine may be superior to β-agonists in the emergency department and for hospitalized patients, it is not typically prescribed in the outpatient setting due to potential adverse effects.
The role of corticosteroids in the treatment of bronchiolitis is also controversial. Studies have typically used 3- to 5-day courses of either dexamethasone (0.6 mg/kg to a maximum of 15 mg orally once daily) or prednisolone (1–2 mg/kg/day to a maximum of 60 mg orally divided twice daily). One of the largest clinical trials examining corticosteroids in bronchiolitis enrolled 600 infants 2 to 12 months of age with moderate to severe bronchiolitis across 20 pediatric emergency departments in the United States. This study demonstrated that a single 1 mg/kg dose of oral dexamethasone did not change hospitalization rate, respiratory state at 4 hours after treatment or longer-term outcomes compared to placebo.44 A meta-analysis of six placebo-controlled studies (347 total patients) of corticosteroid therapy demonstrated a small but statistically significant reduction in the length of hospital stay; the mean duration of hospitalization was 0.43 day (95% CI: 0.05–0.81) shorter in the corticosteroid group than in the placebo group.45 When the studies that included patients with a history of wheezing were omitted from the analysis, this difference was no longer significant, a finding that supports the notion that certain subgroups with bronchiolitis may benefit from corticosteroid treatment.45 A subsequent meta-analysis of 17 trials including 2596 patients found that corticosteroids did not reduce hospitalization rates or inpatient length of stay.46 Unadjusted results from a single multicenter examining nebulized epinephrine and dexamethasone (1 mg/kg initially, followed by 5 days of 0.6 mg/kg/day) found that only those infants who received both treatments (as opposed to epinephrine and placebo, dexamethasone and placebo, or two placebos) had reduced hospital admissions at 7 days after treatment (RR: 0.65; 95% CI: 0.45–0.95). After adjustment this effect is not significant, making these results exploratory and in need of further investigation.47
Overall, the risks and benefits of corticosteroid administration must be weighed. Because bronchiolitis is generally self-limited and corticosteroid use prolongs viral shedding in RSV bronchiolitis, many clinicians do not favor their routine use in the treatment of children with bronchiolitis. However, subgroups that may benefit from corticosteroid administration include those with recurrent wheezing in the context of a strong family history of atopy and asthma (provided that evaluation for other causes of recurrent wheezing has been undertaken) and those with severe illness or respiratory failure. In summary, current evidence suggests that corticosteroids should not be routinely administered to infants with bronchiolitis. Future studies may demonstrate benefit in combination with certain medications or in certain populations.
Nebulized hypertonic saline is believed to increase mucociliary clearance of pulmonary secretions through its ability to lower the viscosity of mucus, stimulate cilial beat, and reduce airway edema by absorbing water from the mucosa. Although initial trials found that 3% saline reduced hospital length of stay by more than 1 day compared with 0.9% saline, these studies occurred in hospitals with long mean lengths of stay.48 More recent trials with lengths of stay <72 hours, more consistent with most U.S. hospitals, demonstrate no difference in length of stay between 3% and 0.9% saline.49-51 Recent systematic reviews have shown either an attenuated effect of 3% saline compared with prior reviews or no effect on length of stay.52-54 AAP bronchiolitis guidelines therefore do not recommend hypertonic saline use in the ED or in hospitals with average length of stay of >72 hours for infants with bronchiolitis. Although inpatient dosing protocols vary between studies, the most frequently studied regimen is 1.5 mg of racemic epinephrine mixed with 4 mL of 3% saline or 1 to 2.5 mg of albuterol mixed with 4 mL of 3% saline given between every 2 and every 8 hours until discharge.55-59 Four randomized trials found no significant improvement in respiratory distress after isolated administration of hypertonic saline in the emergency department setting.60-63 There have been concerns about bronchospasm related to hypertonic saline administration. Although some data exists suggesting that hypertonic saline is safe as a standalone treatment, most randomized trials have used this treatment with a concomitant bronchodilator.64 In summary, it appears that nebulized hypertonic saline may be beneficial if given repeatedly over time during a hospitalization in hospitals with an average length of stay of >72 hours, but does not seem to have benefit in isolated doses in the emergency department setting.
Mechanical airway clearance involves improving symptoms of bronchiolitis through mobilizing and eliminating respiratory secretions. Chest physiotherapy through chest percussion and postural drainage has been proposed as a physical mechanism to help clear bronchial secretions. A meta-analysis of nine trials involving 891 patients found no differences between chest physiotherapy and no intervention in disease severity, respiratory parameters, oxygen requirements, or length of stay.65 Suctioning may provide temporary relief and improve the ability to sleep and feed. Deep suctioning has been shown to be associated with increased hospital length of stay, likely due to airway trauma and resultant edema.66 There is no evidence to support deep suctioning of the lower pharynx, larynx, or trachea, and such techniques may be potentially harmful.
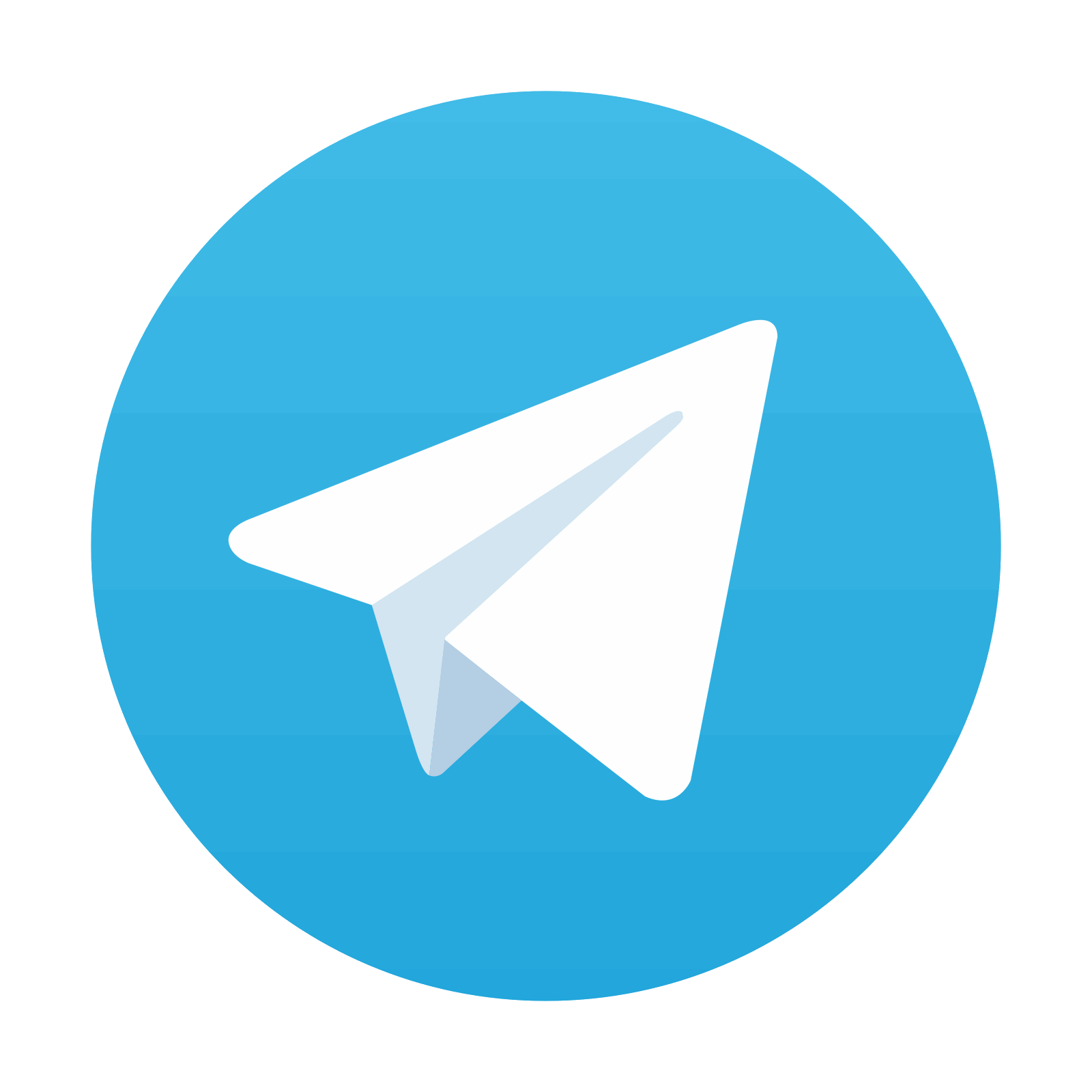
Stay updated, free articles. Join our Telegram channel

Full access? Get Clinical Tree
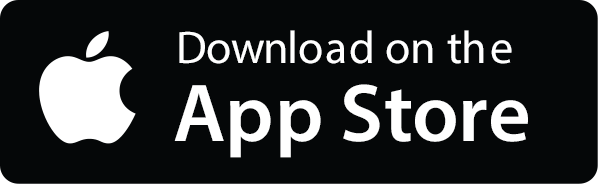
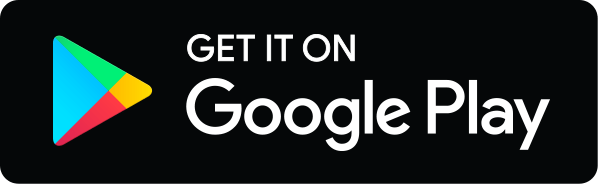