Fig. 1
Schematic anatomy of the right brachial plexus (Marcus and Clarke 2008) (Courtesy of Quality Medical Publishing)
A number of means of classifying the obstetrical brachial plexus injury exist:
(a)
Whether it lies in the supraclavicular (as in the majority of cases) or infraclavicular plexus (which may necessitate an alternate surgical approach).
(b)
Whether the injury is preganglionic or postganglionic – the latter being more common in the lower nerve roots due to them having less robust connective soft tissue attachments to the neural foramina. A preganglionic root avulsion injury results in a permanent motor and sensory deficit in the corresponding dermatome and is not currently amenable to primary surgical repair as the dorsal root ganglion is avulsed from the spinal cord and the ventral rootlets are separated from the anterior horn cells. This is readily evident intraoperatively if the dorsal root ganglion has retracted extraforaminally, although can appear deceptively normal if it remains within the bony limits of the foramina. Postganglionic tears are termed ruptures and are usually amenable to surgical repair as the dorsal root ganglion is preserved proximally (with the distal nerve undergoing Wallerian degeneration).
(c)
According to the description by Seddon of a nerve injury being either a neurapraxia, axonotmesis, or neurotmesis (Seddon 1943). This classification was further expanded to five degrees of injury by Sunderland (1951). Complete recovery would be expected from a neurapraxia as axonal integrity is not violated. In axonotmesis (i.e., axonal damage with intact Schwann cell basal lamina), a partial spontaneous recovery might be expected. Neurotmesis may either represent a cord “avulsion” injury in the context of a preganglionic injury or complete disruption (“rupture”) of nerve continuity in a postganglionic lesion; in the latter scenario, recovery is not possible without surgical intervention. In practice, the picture is invariably more complex, with multiple degrees of nerve injury occurring within the same patient (Mackinnon and Dellon 1988). Indeed, the classic finding of a neuroma-in-continuity has been described as a “Sunderland VI injury” as it is typically a mixture of the other five Sunderland types.
(d)
Based on earlier work by Gilbert and Tassin, Narakas classified obstetrical brachial plexus palsy into four groups, which reflected the histopathologic Sunderland classification. Stratification is based on the neonatal clinical examination findings at 2–4 weeks after birth, by which time neurapraxic injuries will have begun to recover (Table 1) (Gilbert and Tassin 1984; Narakas 1986, 1987).
Group | Affected nerve roots and clinical findings | Approximate rate of complete spontaneous recovery (%) |
---|---|---|
I | C5, C6 | 90 |
Paralysis of shoulder and biceps | ||
II | C5, C6, C7 | 65 |
Paralysis of shoulder, biceps, and forearm extensors | ||
III | C5, C6, C7, C8, T1 | <50 |
Flaccid limb | ||
IV | C5, C6, C7, C8, T1 | 0 |
Flaccid limb and Horner syndrome |
Clinical Assessment
An early referral to a specialist brachial plexus multidisciplinary clinic is encouraged (Borschel and Clarke 2009). The diagnosis of obstetrical brachial plexus palsy is primarily one of clinical acumen; not infrequently a child may need to return for repeated assessment if compliance was lacking at the initial consultation. The clinician must exclude diagnoses that may potentially mimic obstetrical brachial plexus palsy such as underlying intracranial pathology (including cerebral palsy and brain tumors), a radial nerve palsy, or a fracture or dislocation involving the shoulder girdle or upper extremity (“pseudoparalysis ”) (Alsubhi et al. 2011). Plain radiographs should be requested if there is suspicion of an associated bony injury or joint dislocation. Soft tissue contractures tend not to develop until some months after birth; thus any limitation in the passive range of motion of the upper extremity during neonatal assessment should raise the suspicion of underlying musculoskeletal pathology. For example, injury to the proximal humeral epiphyses should be suspected in a child when both active and passive motion of the arm are equally restricted; a plain radiograph should confirm the diagnosis (Adler and Patterson 1967). A thorough birth history including predisposing risk factors and postnatal complications (including respiratory distress, associated fractures, torticollis, or Horner’s syndrome) is taken as detailed in chapter “Supracondylar Humerus Fracture.”
Clinical assessment is best performed with the infant placed on a clean sheet on a padded mat at floor level (or low examination bench) as this facilitates efficient examination in a safe environment. Clothing above the child’s waist should be removed and particular care taken to note the head posture; children with obstetrical brachial plexus palsy tend to look to the contralateral side. The presence of torticollis should be noted; this may result from birth trauma or as a secondary phenomenon due to positioning of the child (invariably, but incorrectly, nursed with the affected extremity uppermost). The lower extremities must also be examined in order to exclude a hemiparesis or neonatal tetraplegia.
The posture of the upper extremity gives invaluable information as to the level of the plexus lesion. Shoulder adduction and internal rotation with the elbow in extension, the forearm pronated, and wrist/fingers held in flexion (the “waiter’s tip” position) is highly suggestive of an Erb-Duchenne upper plexus (C5, C6 ± C7) injury. Recent evidence indicates that the diagnostic specificity of the “waiter’s tip” posture in infants is less than was originally assumed, as the only absolute requirement to produce a “waiter’s tip” posture is an intact T1 nerve root (Fattah et al. 2012). A flail limb, with the absence of any motor activity, implies a total plexus palsy (C5-C8, T1). Less common phenotypes include a Klumpke lower plexus (C8, T1) palsy (a flaccid hand in an otherwise functioning upper extremity), an intermediate (C7, C8, T1) palsy (with an abducted shoulder, flexed elbow, and flaccid hand), and an isolated C7 palsy (with the elbow held in a flexed posture).
Physical signs that would support the diagnosis of an upper plexus injury include winging of the scapula (due to paralysis of the long thoracic nerve of Bell; root value C5-C7), although assessment of the scapulae in neonates is not possible. In addition, paradoxical breathing between the chest and abdomen could be the result of an associated phrenic nerve injury (root value C3-C5; although the phrenic nerve typically contributes to C5) though this is impossible to detect clinically. An ipsilateral Horner syndrome (characterized by ptosis, miosis, anhidrosis, and enophthalmos) can be seen on routine examination and indicates disruption of T1 proximal to the sympathetic rami communicantes (i.e., a preganglionic avulsion injury) (Fig. 2).
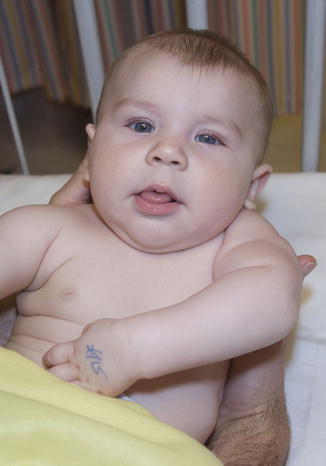
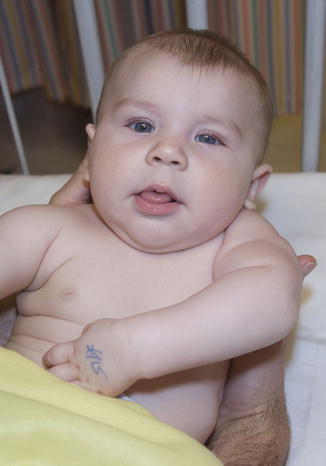
Fig. 2
Four-month-old child with left global brachial birth palsy and Horner’s syndrome (Courtesy of Shriners Hospital for Children, Philadelphia)
A thorough motor examination of the upper extremity is central to confirming the diagnosis, prognosis, and subsequent treatment plan and probably represents the most challenging element of the examination. Traditionally, the Medical Research Council muscle grading scale (Table 2) has been used (Medical Research Council 1943); however, its use in infants is limited on account of their inability to fully cooperate with the examination, and thus the authors favor the validated Active Movement Scale developed at The Hospital for Sick Children (Table 3) (Clarke and Curtis 1995; Curtis et al. 2002). Fifteen cardinal motions of the upper extremity are assessed, including those of the shoulder (abduction, flexion, internal rotation, and external rotation), elbow (flexion and extension), forearm (pronation and supination), and wrist, fingers, and thumb (flexion and extension). The Active Movement Scale measures motion within the range of joint motion, not voluntary strength. It incorporates gravity as a standard; movements are tested both against gravity and with gravity eliminated in order to document early recovery with precision. The Mallet scale (Fig. 3), which was developed to provide a quantifiable assessment of shoulder function in obstetrical brachial plexus palsy, is also widely used although it demands a degree of patient compliance that negates its routine use in children under the age 3 years (Mallet 1972).
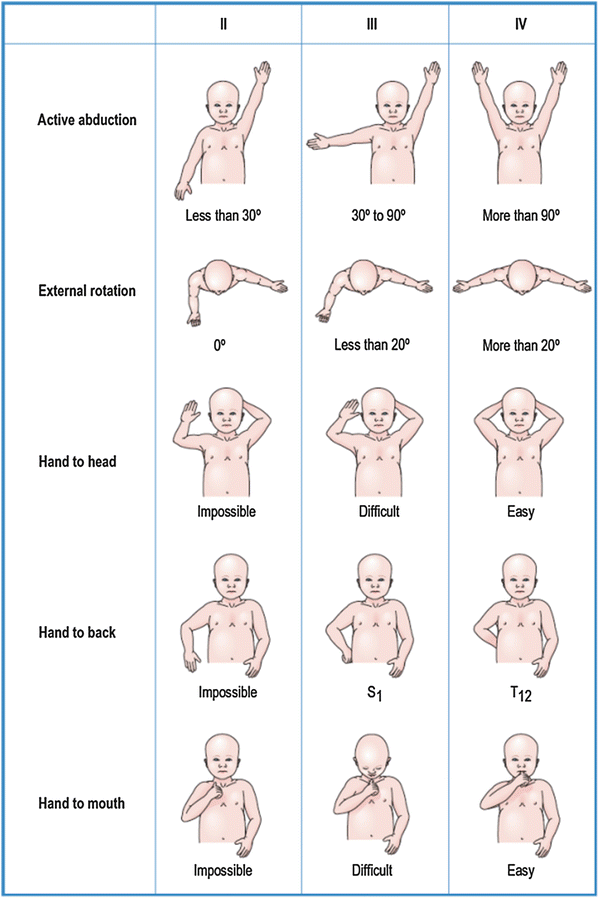
Observation | Muscle grade |
---|---|
No contraction | 0 |
Flicker or trace of contraction | 1 |
Active movement, with gravity eliminated | 2 |
Active movement, against gravity | 3 |
Active movement, against gravity and resistance | 4 |
Normal power | 5 |
Observation | Muscle grade |
---|---|
Gravity eliminated | |
No contraction | 0 |
Contraction, no motion | 1 |
Motion ≤½ range | 2 |
Motion >½ range | 3 |
Full motion | 4 |
Against gravity | |
Motion ≤½ range | 5 |
Motion >½ range | 6 |
Full motion | 7 |
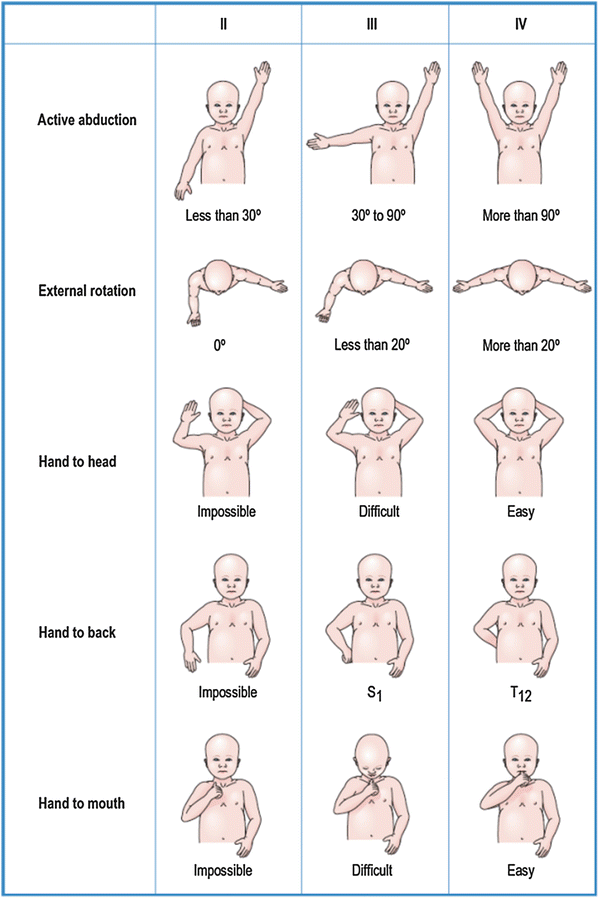
Fig. 3
The Mallet method of assessing upper extremity function (Chung et al. 2012) (Courtesy of Elsevier Saunders)
Indications for Surgical Exploration
It is generally accepted that in those children who meet the criteria for surgical intervention, treatment should not be delayed, as this is likely to prejudice the maximal benefit that could otherwise be obtained. The challenge facing brachial plexus surgeons has been to evolve the ability to statistically prognosticate which children are most likely to benefit from surgical intervention versus those who are best managed expectantly. Clearly, surgical intervention should only be warranted if the outcome after intervention is likely to result in enhanced long-term function compared to nonoperative management; such a judgment requires a sound understanding of the natural history of obstetrical brachial plexus palsy. Identification is straightforward in the scenario of an infant with a flail limb and an associated Horner syndrome; equally, surgery is contraindicated in a child with mild paralysis who recovers substantial motor function within the first month as near complete functional recovery would be anticipated (Al-Qattan et al. 2000). Clinical evidence of an isolated T1 avulsion (i.e., a limp hand resting in the intrinsic minus position) is a reliable indicator for operative intervention within the first 3 months. Despite accepting that the majority of children with obstetrical brachial plexus palsy will not require surgical exploration, there is currently no universal consensus as to how to ascertain which children will – with reasonable certainty – require surgery.
Tassin noted that complete recovery of shoulder function at the age of 5 years (Grade V in the Mallet classification system) was only seen in those children with contraction of biceps and deltoid muscles in the first month and normal contraction of both muscles by the age of 3 months (Tassin 1983). Gilbert and coworkers found testing the deltoid problematic in neonates (due to difficulty in isolating its action from pectoralis major activity) and therefore generated three modified criteria for surgical intervention: a flail arm with Horner syndrome, a complete C5–C6 palsy without muscle contractility by 3 months and a negative EMG (thus suggestive of a root avulsion), and a C5–C6 palsy with no biceps activity at 3 months (Gilbert et al. 1988). Failure of spontaneous return of elbow flexion at 3 months of age is now the most widely accepted criteria for surgical intervention in infants with an upper plexus lesion (Gilbert and Whitaker 1991; Gilbert 1995; Eng et al. 1996; Gilbert et al. 2006).
Waters confirmed the findings of Tassin and Gilbert that infants in whom biceps function recovered by 3 months of age progressed to achieve normal neurologic function, while those whose biceps recovery occurred late (i.e., from 4 to 5 months of age) had a worse long-term recovery according to the Mallet classification (Waters 1999). Those infants who had microsurgical brachial plexus reconstruction for absent biceps recovery by 6 months of life had superior function to those who experienced late spontaneous biceps recovery, but their function was not as good as those in whom elbow flexion recovered early.
However, Michelow et al. determined that overreliance on a single functional parameter alone at the age of 3 months would incorrectly predict a poor recovery in 12.8 % of patients, additionally implying that some children were being reconstructed unnecessarily. This false-positive rate could be reduced to 5.2 % if elbow flexion and elbow, wrist, thumb, and finger extension were incorporated into a combined test score. The utility of adopting multiple muscle assessments as an indicator for surgical intervention is becoming increasingly accepted and should lead to a reduction in unnecessary surgery, although a more conservative threshold may result in some children who would benefit from surgery ultimately being managed nonoperatively (Laurent et al. 1993).
The importance of repeat assessment has been promoted by Clarke and Curtis as a small proportion of infants demonstrating evidence of return of elbow function at 3 months may then fail to progress considerably with an ultimately suboptimal recovery, thus endorsing the concept of reassessment at 3-monthly intervals (Clarke and Curtis 1995). The algorithm used for the assessment and management of infants presenting with obstetrical brachial plexus palsy at The Hospital for Sick Children is detailed in Fig. 4. An initial assessment is undertaken at 3 months using the Active Movement Scale (Table 3); a converted test score (the sum of the five scores for elbow flexion and extension of the elbow, wrist, fingers and thumb after conversion according to the values in Table 4) less than 3.5 is strongly predictive of a poor functional outcome and surgical reconstruction is therefore recommended. Those children with a test score of 3.5 or greater, with an intact T1 and no evidence of a Horner syndrome, are observed and undergo daily physical therapy to maintain passive range of motion; they are subsequently reassessed a 3-month interval. In the scenario of a child who initially demonstrates biceps function at the 3-month assessment, but then fails to meaningfully progress by the 6-month assessment (i.e., similar test scores are obtained), then it is likely that this heralds a poor functional outcome and surgical intervention should be considered.
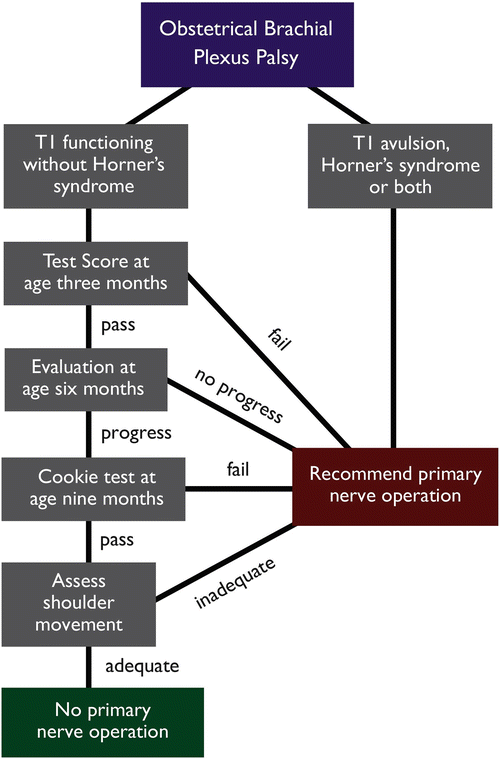
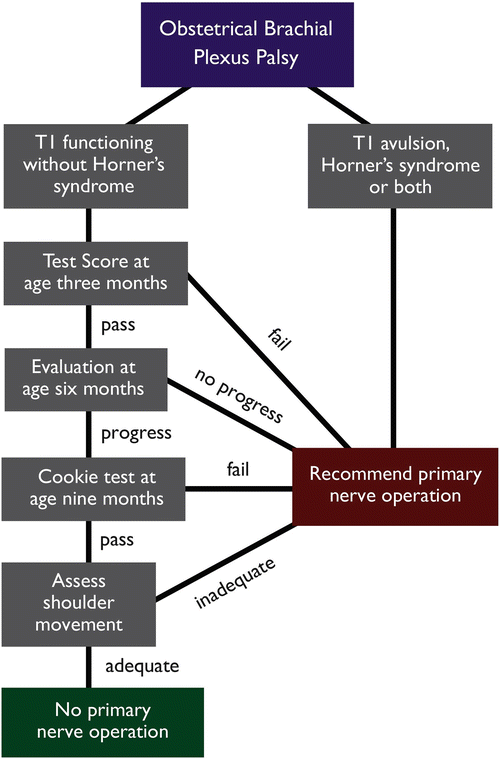
Fig. 4
Flow diagram for the assessment and management of obstetrical brachial plexus palsy at The Hospital for Sick Children (Courtesy of Dr. H. M. Clarke, The Hospital for Sick Children)
Table 4
The Hospital for Sick Children Active Movement Scale conversion for use in calculating the interval test score (Clarke and Curtis 1995)
Muscle grade (from Active Movement Scale) | Converted score |
---|---|
0 | 0 |
1 | 0.3 |
2 | 0.3 |
3 | 0.6 |
4 | 0.6 |
5 | 0.6 |
6 | 1.3 |
7 | 2.0 |
At the 9-month assessment, the child undertakes the “cookie test ” in an attempt to identify those in whom a good early recovery (i.e., a test score of 3.5 or greater) failed to predict adequate elbow flexion by the end of the first year (Clarke and Curtis 1995; Curtis et al. 2002). If the child fails to achieve greater than half the range of elbow flexion against gravity (i.e., an Active Movement Score of at least 6), then exploration of the brachial plexus is advocated (Fig. 5). In practice, the test is performed with the child either seated or standing with the elbow held adducted against the trunk by the examiner to negate the trumpet sign; a small cookie is placed into the hand of the child, and they are encouraged to eat the cookie by actively flexing their elbow against gravity. The test is considered void if there is excessive neck flexion (greater than 45°). Application of the test score should never supplant sound clinical judgment; it is intended as a guide to early treatment.
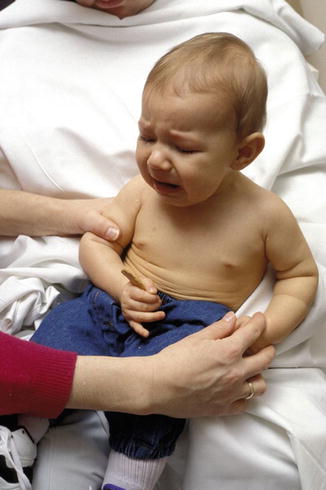
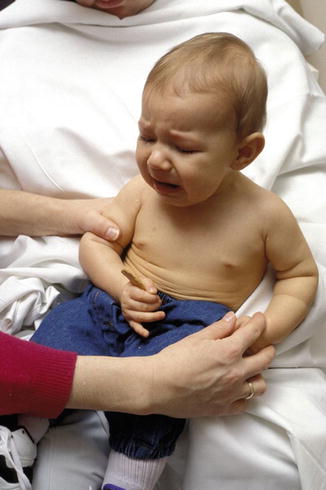
Fig. 5
Failed “cookie test” at 9 months of age: the child fails to achieve greater than half the range of elbow flexion against gravity (Courtesy of Dr. H. M. Clarke, The Hospital for Sick Children)
Preoperative Investigations
In those patients who require surgical exploration, a preoperative diaphragmatic ultrasound is performed to document phrenic nerve function (Borschel and Clarke 2009). A chest radiograph is recommended if considering extra-plexal neurotization from the intercostal nerves (Slooff et al. 2001). Additionally, a computed tomographic myelography is undertaken to determine the probability of a root avulsion injury: the presence of a pseudomeningocele without demonstrable ventral rootlets indicates (with a specificity of 0.98) that the nerve root is probably avulsed (Chow et al. 2000). Pseudomeningoceles indicate an underlying dural injury, and in isolation, they are not absolute proof of a root avulsion injury – discontinuity of the rootlets should be identified in both axial and coronal planes to confirm the diagnosis of a preganglionic injury. The necessity for general anesthesia, intrathecal instillation of contrast medium, and exposure to ionizing radiation has driven the application of magnetic resonance imaging (MRI) in the radiologic assessment of the infant brachial plexus as it is noninvasive, nonionizing, and can be performed under sedation alone. However, MRI currently lacks the higher spatial resolution of CT myelography in identifying individual nerve roots (Caranci et al. 2013), and the quality of the images appears to vary widely across centers. Many specialist units do not routinely image the brachial plexus save for specific indications such as a suspected upper root avulsion injury following a breech delivery (Gilbert 1995; Al-Qattan 2004; Shenaq et al. 2004).
Electrodiagnostic studies have been used to provide information on the location, severity, and extent of the obstetrical brachial plexus palsy. Although more challenging to perform in infants, nerve conduction studies (NCS) may help to differentiate between preganglionic and postganglionic plexus injuries. Motor and sensory nerve responses (conduction velocities and distal latencies) vary significantly with age and in neonates are approximately 50 % the magnitude of those seen in adults (Miller and Kuntz 1986). Electromyography (EMG) is not routinely used in the assessment of obstetrical brachial plexus palsy; Gilbert et al. found that EMG findings correlated poorly with prognosis, although evidence of fibrillation on the background of absent voluntary motor unit activity was suggestive of a root avulsion injury (Gilbert et al. 1988). Overall, there is reasonable agreement that electrodiagnostic studies are overly optimistic in the setting of obstetrical brachial plexus palsy and are therefore not used at The Hospital for Sick Children, Toronto (Vredeveld et al. 1999).
Surgical Exploration and Reconstruction
Intraoperative Setup
The working relationship between the surgeon, anesthesiologist, and theatre scrub staff is essential; the procedure is lengthy and complex, and thus communication within the team is vital with the perioperative safety of the child being of paramount importance. Factors that deserve specific attention include the duration of the procedure, which is typically between 6 and 12 h, with the attendant risks of patient hypothermia, pressure area necrosis, and fluid imbalance; the availability of only one extremity for intravenous access and noninvasive monitoring purposes (as both lower extremities are usually prepped for sural nerve harvest); and the need to avoid muscle paralysis if intraoperative nerve stimulation is to be employed. The neuropathology laboratory is forewarned that fresh specimens will be obtained for frozen section and will require urgent processing. The key considerations in the preoperative planning are outlined in Table 5.
Table 5
Preoperative planning checklist for brachial plexus exploration and reconstruction with bilateral sural nerve harvest
Preoperative planning checklist | |
---|---|
Operating table | Table reversed in order that the patient not resting on break in the table |
Airway | Nasal intubation secured with silk suture |
Transparent drape | |
Patient position | |
a. Bilateral sural nerve harvest | a. Prone with abdominal support |
b. Brachial plexus exploration | b. Supine with shoulder roll in situ |
Tourniquet | Sterile; placed on bilateral proximal thighs |
Sterile Esmarch bandages | |
Pressure care | Egg crate mattress |
All bony prominences padded | |
Temperature homeostasis | Rectal temperature probe |
Access to forced-air warming if required | |
Fluid management | Urinary catheter in situ |
Total fluid intake ≤4 ml/kg/h | |
Specific equipment | |
a. Sural nerve harvest | a. 0° 4 mm endoscope |
b. Assessment of brachial plexus | b. Nerve stimulator |
c. Microsurgery | c. Operating microscope and instruments |
d. Nerve grafts | d. Fibrin sealant |
Miscellaneous | a. Ligaclip applicators |
b. Book frozen section with a neuropathologist | |
c. 2-inch stockinette and safety pins for Velpeau splint |
Following nasal intubation with an uncuffed endotracheal tube, the airway is secured by suturing the tube to the membranous nasal septum with a 3-0 silk suture to minimize the risk of inadvertent extubation. The child is catheterized with a size 8 Foley for the convenience of the team; it is only rarely advised to treat low urine output with a fluid bolus. Prophylactic intravenous cefazolin is administered, with the dose repeated every 4 h for the duration of the procedure. A rectal temperature probe is used to monitor core temperature throughout the operation.
Bilateral Sural Nerve Harvest
In most situations the surgeon will be confident that sural nerve grafting is necessary and the procedure begins with the child in the prone position in preparation for bilateral sural nerve harvest. An abdominal bolster is used to facilitate patient positioning with meticulous care taken to pad all pressure areas appropriately. Endoscopic-assisted sural nerve harvesting is used to minimize donor site scarring (Capek et al. 1996). The harvests are performed under tourniquet control using three 2-cm incisions for access at the level of the lateral malleolus, the distal belly of the gastrocnemius muscle (anticipating the point at which the nerve becomes subfascial in the mid-calf), and the popliteal fossa. Endoscopic visualization can facilitate identification of the entire sural nerve from the most proximal origin at the tibial nerve to the distal arborizations at the lateral aspect of the foot, together with the contributing lateral (peroneal) branch, which is also harvested if present. Approximately 15 cm of sural nerve can be harvested from each leg in a 10 kg infant. The nerves are marked with ink in order to aid orientation and stored in a damp sterile container in a refrigerator until later required in the reconstruction. Sural nerve harvesting in children leaves a measurable sensory deficit; however, this does not appear to have significant clinical implications (Lapid et al. 2007).
Approach to the Brachial Plexus
On completion of the sural nerve harvest, the child is turned supine and placed at the extreme head of the table, close to the lateral edge, in order to facilitate access for both the surgeon and assistant (Fig. 6). A small padded roll is placed under the scapulae in order to provide a modest degree of neck extension; the head is turned fully away from the affected side. The upper extremity is free draped in order to facilitate observation of the entire limb during intraoperative nerve stimulation. Clear draping of the patient’s head enables the anesthesiologist to continually visualize the endotracheal tube.
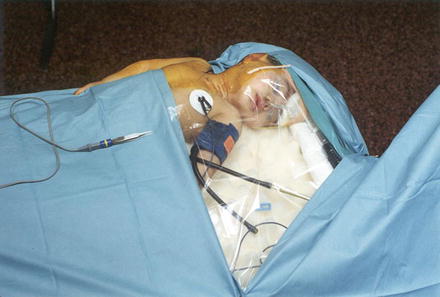
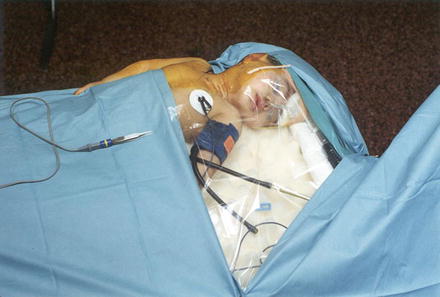
Fig. 6
Intraoperative positioning of the infant for a right brachial plexus exploration: note the use of a clear drape allows visualization of the endotracheal tube (Courtesy of Dr. H. M. Clarke, The Hospital for Sick Children)
The vast majority of infant plexus explorations are performed via a supraclavicular approach in the posterior triangle of the neck. A variety of different incisions are used in order to access the brachial plexus, with many surgeons favoring a single transverse incision just above the clavicle (Borrero 2001). Excellent exposure to the contents of the posterior triangle of the neck is afforded by a superiorly based V-shaped incision with the medial limb running along the posterior border of the sternocleidomastoid muscle and then gently curving at its apex to create the lateral limb which runs parallel to the superior border of the clavicle (with the potential to extend into the deltopectoral groove should this be necessary to access the more distal plexus). A step-by-step description of this surgical approach is listed in Table 6. The tip and edges of the skin flap are tattooed with surgical ink to facilitate accurate wound alignment at the time of wound closure. Subsequent dissection is performed under loupe magnification in a subplatysmal plane with the skin flap being anchored superolaterally beyond the surgical field by means of a hemostat. The clavicular head of the sternocleidomastoid muscle is divided near its origin to facilitate exposure; the overlying external jugular vein is frequently divided if it obstructs the surgical field.
Table 6
A step-by-step approach to exploration of the brachial plexus
Surgical steps |
---|
Tattoo preoperative markings to facilitate subsequent wound closure |
Elevate skin flap in subplatysmal plane and reflect superolaterally
![]() Stay updated, free articles. Join our Telegram channel![]() Full access? Get Clinical Tree![]() ![]() ![]() |