Materials and Methods
Reagents and cell lines
The K-ras/PTEN cell line were established by us from ovarian tumors that were generated with the use of a genetic mouse model. The SKOV3ip1 and HeyA8 cell lines were provided by Dr Gordon Mills (MD Anderson Cancer Center, Houston, TX). The IOSE 397 cell line was shared by Dr Nelly Auersperg (University of British Columbia, Canada). The IGROV1 and Ovcar-5 cell lines were purchased from American Type Culture Collection (Manassas, VA). The Kuramochi cell line was purchased from the Japanese Collection of Research Bioresources Cell Bank (Osaka, Japan). Cell lines were validated by short tandem repeat DNA fingerprinting with the use of the AMPF’STR Identifier kit (Applied Biosystems, Foster City, CA) and compared with American Type Culture Collection and University of Texas MD Anderson Cancer Center (Houston, TX) fingerprints. Metformin was obtained from Sigma-Aldrich (St. Louis, MO). The Cdk4, cyclin D1, AMPK, EGFR, ErbB4, PDGFRα, FABP4, and fatty acid synthase (FASN) antibodies were from Cell Signaling Technologies (Beverly, MA); the cyclin D1 antibody used for immunohistochemistry was from Novus Biologicals (Littleton, CO). The LKB1 and ACC antibodies were from Millipore (Billerica, MA); PARP 1/2 was from Santa Cruz Biotechnology (Santa Cruz, CA); FASN was from ATLAS (Stockholm, Sweden); phosphorylated RON was from R&D Systems (Minneapolis, MN), and RON was from Epitomics (Burlingame, CA).
MTT assay
Cells were plated in quadruplicate into 96-well plates and treated with vehicle control, paclitaxel, and/or metformin for the designated amount of time; cellular proliferation was measured with the use of 3-(4,5-dimethylthiazol-2-yl)-2,5-diphenyltetrazolium bromide (MTT) assays as previously described. The effect of treatment was calculated as a percentage of control cell growth that was obtained from vehicle-treated cells that were grown in the same plate. Each experiment was conducted in triplicate.
Apoptosis and cell cycle analysis
Cells were serum-starved for 24 hours, treated for 24 hours, fixed, and resuspended in propidium iodide/RNase–staining buffer or Annexin V and propidium iodide staining buffer and analyzed with a FACS Calibur (Becton Dickson, San Jose, CA). The percentage of cells in the G 2 /M, S-phase, and sub-G 0 -G 1 population (apoptotic cells) was determined with FlowJo software (Flowjo, LLC, Ashland, OR). Staurosporine treatment served as a positive control. Each experiment was conducted in triplicate.
Western blot analysis
Cells were serum-starved for 24 hours and treated with metformin or vehicle control for the indicated amount of time. Western blots were performed as previously described. Briefly, the cells were lysed in radioimmunoprecipitation assay buffer; cell extract (30 μg) was separated by sodium dodecylsulfate-polyacrylamide gel electrophoresis and transferred to a nitrocellulose membrane. The membrane was incubated with respective primary antibodies and then with secondary horseradish peroxidase-conjugated immunoglobulin G and visualized with enhanced chemoluminescence reagents.
Quantitative reverse transcription–polymerase chain reaction
RNA was extracted from OvCa cells with the use of TRIzol (Invitrogen, Carlsbad, CA) and was transcribed into complementary DNA with a high-capacity complementary DNA kit (Applied Biosystems). Quantitative real-time reverse transcriptase–polymerase chain reaction was performed as described with the Applied Biosystems 7500 Real Time PCR system (Applied Biosystems) and the following probes (Applied Biosystems): carnitine palmitoyltransferase 1a (CPT1a, Hs00912681_m1), fatty acid binding protein 4 (FABP4, Hs00609791_m1), and glyceraldehyde 3-phosphate dehydrogenase (GAPDH, Hs00266705_gl). Relative levels of messenger RNA (mRNA) expression were calculated by the 2 -ΔΔCT method.
Receptor tyrosine kinase array
The expression level of activated (phosphorylated) receptor tyrosine kinases (RTK) were analyzed with the Proteome Profiler Human Phospho-RTK Array Kit (R&D Systems,) according to the manufacturer’s protocol. Briefly, OvCa cells were serum-starved for 24 hours and treated with metformin or vehicle control for 48 hours. The cells were then lysed in NP-40 lysis buffer, RTK array membranes were incubated with cell lysates (500 μg), and then incubated with horseradish peroxidase conjugated anti-phosphotyrosine and visualized with enhanced chemoluminescence reagents. The level of phosphorylated RTK was quantified densitometrically with Image J software (National Institutes of Health, Bethesda, MD) and normalized to the internal phosphotyrosine-positive control spots.
Immunostaining
Mouse tumors were formalin-fixed, paraffin-embedded, sectioned, and mounted on slides. Immunohistochemistry staining of slides for terminal deoxynucleotidyl transferase dUTP nick end labeling (TUNEL) and Cyclin D1 was performed as previously described. TUNEL staining was performed using a TUNEL apoptosis detection kit (Millipore) according to the manufacturer’s protocol. Quantification of immunostaining was performed with the Automated Cellular Imaging System (Clarient, San Juan Capistrano, CA) by setting color-specific thresholds to determine brown (positive) and blue (negative) nuclei within 12 representative regions per slide and by calculating the ratio of positively stained nuclei to all nuclei, expressed as a region score.
Glycolysis
Glycolysis and glycolytic capacity were measured with the Seahorse Extracellular Flux (XF-96) analyzer (Seahorse Bioscience, North Billerica, MA). Cells were cultured in Seahorse XF-96 plates at a density of 30,000 cells per well and grown in complete growth media for 3 days. On the day of the experiment, cells were changed to unbuffered DMEM in the absence of glucose. Sequential injections were performed with D-glucose (2 g/L), oligomycin (1 μmol/L), and 2-deoxyglucose (100 mmol/L). The extracellular acidification rates (ECAR) after the injection of D-glucose was a measure of glycolysis, and the ECAR after the injection of oligomycin represented maximal glycolytic capacity. Nonglycolytic activity was quantified by the measure of ECAR after the injection of 2-deoxyglucose.
Animals
The xenograft model was described previously. Mice were treated for 2 weeks with metformin, 250 mg/kg/d, or control injected intraperitoneally, then SKOV3ip1 cells (1 × 10 6 ) were injected intraperitoneally. Treatment was continued until mice were sacrificed 4 weeks later. The LSL- K-ras G12D/+ PTEN loxP/loxP genetic mouse model was obtained from the Massachusetts Institute of Technology (Boston, MA) and used as previously described. OvCa was initiated by intrabursal injection of AdCre virus (2.5 × 10 7 plaque-forming units) in the right ovary; the left ovary was not injected and served as an internal control. Two weeks after the initiation of OvCa, treatment began with control, paclitaxel 3 mg/kg/wk injected intraperitoneally, or metformin added to water for an estimated final dose of 100 mg/kg/d per mouse. Mice were sacrificed 9 weeks after the injection of the virus; tumors were excised, weighed, and measured; and the number of metastatic nodules and volume of ascites were recorded. All animal procedures were approved by the Institutional Animal Care and Use Committee of the University of Chicago. All animal studies were performed in adherence with the Animal Research: Reporting of In Vivo Experiments guidelines for reporting animal research. This study did not involve human subjects and did not require institutional review board approval.
According to published pharmacokinetic analysis of metformin-treated mice, the doses of oral and intraperitoneal metformin administered here would result in serum levels of 0.16 and 4 μg/mL, respectively. In humans who receive 1 g of metformin twice daily, the metformin plasma concentrations range from 0.05-4 μg/mL.
Statistical analysis
Data were analyzed by an unpaired, 2-tailed Student t test of significance, assuming equal variance of the test and the control populations. For nonparametric data, a Mann-Whitney U test was completed to compare the medians for all groups. Data are presented as mean ± standard deviations. A probability value of < .05 was considered significant. All data analysis was performed with STATA software (version 13; StataCorp, College Station, TX).
Results
Metformin decreases proliferation of OvCa cells through induction of cell cycle arrest
To evaluate the effect of metformin in OvCa, 5 different OvCa cell lines that are commonly used in preclinical studies and immortalized human ovarian surface epithelial (IOSE) cells were treated with metformin, and the proliferation rate was measured. Metformin treatment resulted in a significant dose-dependent decrease in proliferation in 4 of 6 cell lines; the exceptions were the Kuramochi and IOSE lines, both of which were much less sensitive to metformin treatment ( Figure 1 , A). Metformin may inhibit carcinogenesis, in part, through activation of AMPK. To evaluate the effect of metformin on AMPK signaling in our model systems, we first demonstrated that LKB1, a tumor suppressor that regulates AMPK function, was expressed in cell lines ( Figure 1 , B). Metformin treatment produced a dose-dependent increase in the levels of phosphorylated AMPK at Thr172 in all cell lines, except the Kuramochi and IOSE lines ( Figure 1 , C).
To understand the mechanism by which metformin inhibited proliferation of OvCa cells, we tested whether metformin induced apoptosis. First, cleaved and total PARP 1/2 was evaluated with the use of Western blots, and no change was noted with metformin treatment ( Figure 2 , A). Consistent with this finding, flow cytometry for Annexin V/PI revealed no evidence of increased apoptosis in metformin-treated cells ( Figure 2 , B). Therefore, to find an alternative explanation for metformin’s inhibition of proliferation, we evaluated the drug’s effect on cell cycle. Analysis with flow cytometry demonstrated arrest of the cell cycle in the G 0 /G 1 phase with metformin treatment in 2 cell lines ( Figure 3 , A). In the HeyA8 cell line, the cell cycle arrest induced by metformin was dose- and time-dependent (data not shown). To understand how metformin induced cell cycle arrest in OvCa cells, Cdk4 and Cyclin D1 were evaluated. It was noted that metformin treatment resulted in a time-dependent decrease in the expression of Cdk4 and Cyclin D1 ( Figure 3 , B).
Pleotropic effects of metformin in OvCa
When used as a treatment for diabetes mellitus, metformin acts on the canonical AMPK pathway, altering cellular glucose and lipid metabolism. When activated, AMPK inactivates (phosphorylates) a key regulatory enzyme in lipid metabolism, acetyl CoA carboxylase (ACC), that results in decreased malonyl-CoA production. When malonyl-CoA levels are depleted, fatty acid synthesis is decreased (regulated by FASN), and fatty acid oxidation is increased (regulated by CPT-1). We tested whether metformin would induce similar metabolic changes in OvCa.
When we evaluated glucose and lipid metabolism, it was noted that metformin increased glycolytic capacity in the OvCa cells ( Figure 4 , A), increased levels of phosphorylated (inactivated) acetyl CoA carboxylase, and decreased FASN ( Figure 4 , B). In addition, metformin increased mRNA levels of CPT-1, which suggested increased fatty acid oxidation ( Figure 4 , C). Previously, our laboratory has shown that adipocytes contribute to ovarian tumorigenesis by transferring free fatty acids to adjacent cancer cells and that this tumor-promoting effect of adipocytes can be interrupted by pharmacologically inhibiting a protein that is involved in fatty acid transport, FABP4. Therefore, we asked whether FABP4 could be a unique target of metformin in OvCa. Interestingly, we found that metformin decreased both the mRNA and protein levels of FABP4 in OvCa cells ( Figure 4 , B and C).
Although metformin-induced alterations in glucose and lipid metabolism are recognized mechanisms of action of the drug in diabetes mellitus, glucose and lipid metabolism are not well-established targets for OvCa therapeutics. Therefore, we also asked whether metformin, independent of its traditional metabolic effects, could also alter validated cancer signaling pathways, specifically RTKs. To test this, phosphorylated RTK arrays were completed in 2 different OvCa cell lines. The arrays indicated that metformin treatment decreases activity of RON, ErbB4, and PDGFRα in SKOV3ip1 cells and reduces the activity of EGFR in HeyA8 cells ( Figure 5 , A). The RTK array findings for RON, ErbB4, and EGFR were confirmed with the use of Western blots; the effect of metformin on PDGFR activity was difficult to interpret because metformin treatment reduced total and phosphorylated PDGFR ( Figure 5 , B).
Metformin inhibits OvCa cell growth and metastasis
To investigate whether the promising in vitro findings would translate into a protective effect of metformin in animal models of OvCa, we used 2 approaches: a preventive and treatment study design. In the preventive study, SKOV3ip1 xenograft mice were pretreated with metformin or placebo for 2 weeks before the initiation of OvCa, with continuation of metformin treatment until completion of the study ( Figure 6 , A). In this experiment, at the time of sacrifice, mice that had been treated with metformin had significantly fewer tumor implants (mean number of tumors: placebo, 116; metformin, 47; P < .005; Figure 6 , B).
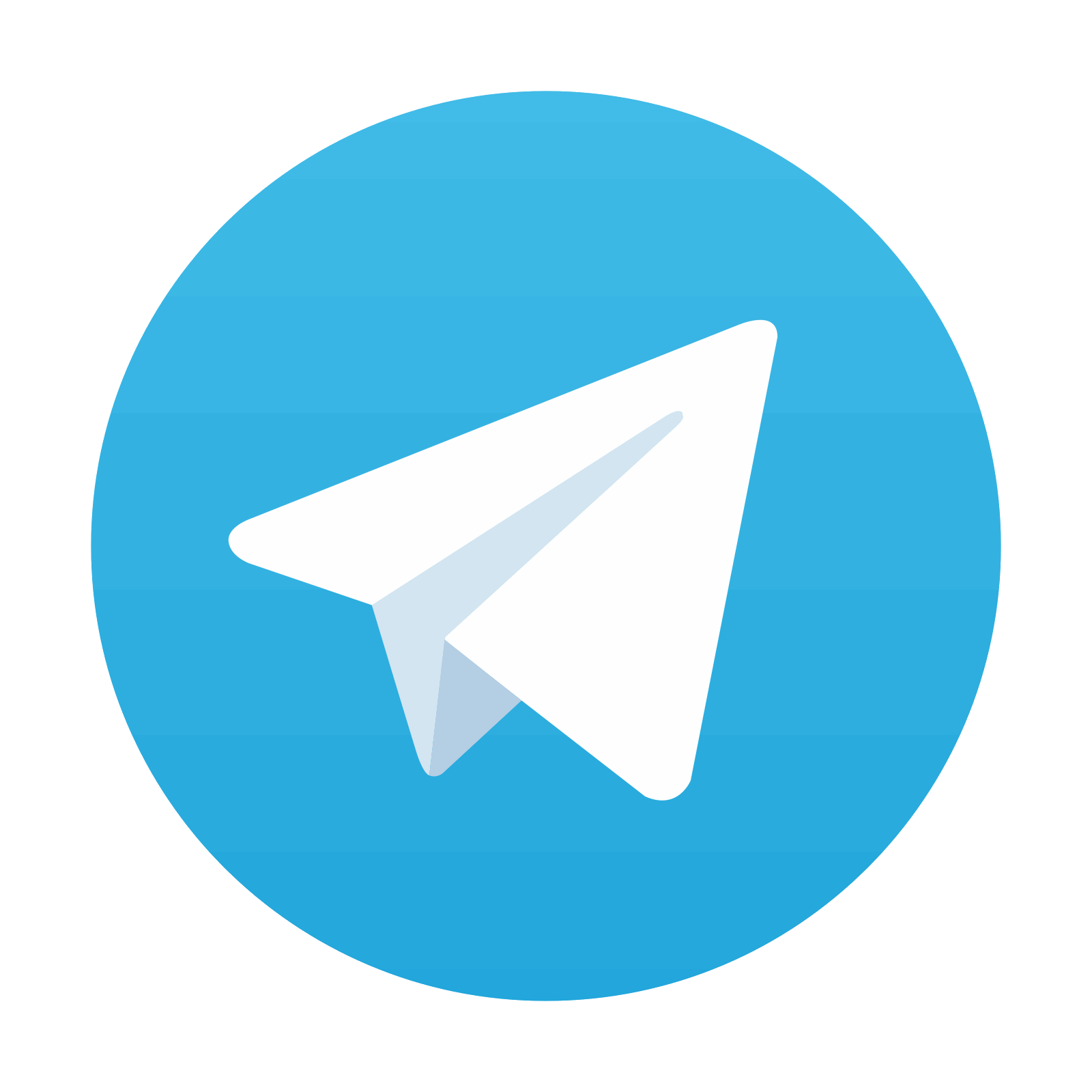
Stay updated, free articles. Join our Telegram channel

Full access? Get Clinical Tree
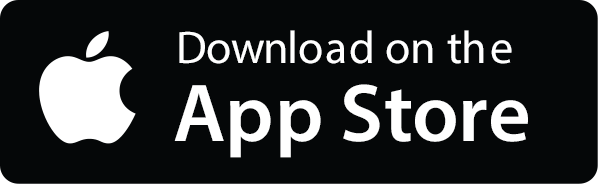
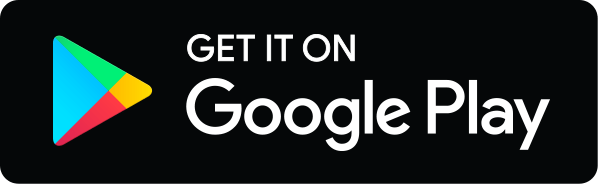
