Children have reduced metabolic reserves and are particularly susceptible to the adverse effects associated with the catabolic response to critical illness.
The metabolic response to injury is proportional to the degree of stress.
Both protein degradation and protein synthesis increase during illness; however, quantitatively the former predominates. Hence, net negative protein balance ensues.
Adequate supplementation of protein is the most important nutritional intervention in children during illness.
In illness the breakdown of existing skeletal muscle stores and consequent release of amino acids is driven by increased requirements. These needs include amino acids to synthesize new proteins and the conversion of these amino acids to form glucose through the process of gluconeogenesis. During the metabolic stress state, this catabolic process is refractory to inhibition by the supplementation of dietary glucose.
Energy expenditure can be measured either directly or indirectly. Direct calorimetry is very precise but not practical as a method to use in sick children. Indirect calorimetry requires a leak-free system, which can be difficult to achieve in children with uncuffed endotracheal tubes or not breathing calmly. Other indirect methods include deuterated water and 13C-labeled bicarbonate, which have been shown to correlate with indirect calorimetry.
In neonates and children, the increase in resting energy expenditure associated with surgery is very brief and is measured in hours.
Free fatty acids are the primary source of energy in stressed patients. In neonates, with limited stores, this makes them more susceptible to develop essential fatty acid deficiency. The quantity of lipid necessary to obviate fatty acid deficiency is relatively limited. Newer experimental data support the use of lipid-limited omega-3 formulas, in highly selected patients with cholestasis and a long-term requirement for parenteral nutrition.
During illness neural pathways, hormones, and inflammatory mediators mediate the prolonged metabolic response. The ability to effectively modulate these responses could result in an amelioration of the net negative protein balance that exists during stress states. Neural pathway modulation is achieved with adequate sedation and analgesia and has been shown to decrease the hypercatabolic state in ill neonates. Hormonal and inflammatory mediator modulation remains experimental at this time.
Surgery, trauma, and sepsis are accompanied by a constellation of metabolic aberrations that tend to be profound and predictable. Sir David P. Cuthbertson described the fundamental aspects of this metabolic response to injury in the adult over 50 years ago. By observing patients with long-bone fractures and utilizing complementary animal models, he determined that the excessive output of nitrogen following the stress of injury was a result of the catabolism of whole-body protein rather than merely the loss of muscle mass surrounding the site of injury. Diet, particularly protein intake, affected the degree of catabolism. Regardless of the etiology of the injury, the metabolic response seemed to be stereotypic and was characterized by a short “ebb” phase marked by relative hypometabolism followed by a subsequent prolonged rise in metabolic activity termed the “flow” phase. The mechanisms for these changes, however, remained largely obscure until Francis D. Moore carefully elucidated the hormonal and substrate response to surgery. In the early 1980s, a class of short peptide mediators called cytokines were isolated from human white blood cells, and it became evident that neural and endocrine changes accompanying injury along with the release of these peptides were responsible for the unified metabolic response to injury. Recent research has demonstrated that the metabolic sequelae of illness and operation in the neonate and child qualitatively resemble those in the adult, although profound quantitative differences exist. Overall, it is necessary to understand these metabolic alterations in order to design improved therapies to augment the short-term benefits of the pediatric metabolic stress response while obviating its long-term harmful effects.
Children and adults rely on the metabolism of carbohydrates, lipids, and proteins to meet the catabolic demands of injury. Table 6-1 outlines the macronutrient metabolic reserves of reference neonates, children, and adults in terms of percentage of total body weight. Carbohydrate stores remain constant and do not afford significant reserves at any time during maturation. Lipid stores are low at birth and increase somewhat through development, with premature infants having even greater proportionately reduced reserves. The most striking difference in body composition between a healthy adult and a child or neonate is the quantity of protein available in times of injury. Adults have triple the protein stores that neonates possess, and even the elderly have proportionately twice the quantity of protein present in the neonate.
Not only do neonates and children have reduced stores, they have much higher baseline requirements. The resting energy expenditure for adults is one third that reported for growing premature neonates when standardized for weight. This inverse relationship of higher energy expenditure with decreased body weight also holds between mammalian species and is partially related to body surface area. Another obvious etiology for the increased demand is the need for growth itself. The quantity of energy and protein required to obtain optimal growth rates in term neonates and children compared to the requirement for weight and protein balance in adults is listed in Table 6-2. The protein requirements of the full-term neonate are about 3 times those of the adult, with the 10-year-old child more closely resembling the adult. Congruent with this trend, stable premature neonates minimally require 2.8 g/kg/day of protein in order to maintain growth rates approximating that in utero, which is 3.5 times the requirement for protein balance in the adult.
Thus, the child, and particularly the neonate, is potentially much more susceptible to the deleterious effects of protracted catabolic stress imposed by injury because of the presence of reduced stores and markedly increased baseline metabolic demands.
The pediatric response to illness and operation has predictable effects on protein, energy, carbohydrate, and lipid metabolism. Increased intracellular utilization and enhanced interorgan transport are its hallmarks. Alterations in vitamin and trace mineral nutrition appear less pronounced, although much additional research is needed to accurately assess requirements. An understanding of these changes in nutrient metabolism allows for the provision of metabolic support to facilitate growth and recovery in even the most severely ill child (Table 6-1).
Amino acids are the key building blocks required for growth and tissue repair. The majority of amino acids (98%) reside in proteins, with the remainder being in the free amino acid pool. Proteins themselves are not static, as they are continually degraded and synthesized in a process termed protein turnover. The reutilization of amino acids released from protein breakdown is extensive, as is evidenced by the fact that protein turnover is more than 2 times protein intake. Newborns have a protein turnover of 6.7 g/kg/day, whereas adults have a protein turnover of approximately 3.5 g/kg/day. A salient advantage of having high protein turnover is that a continual flow of amino acids is available for new protein synthesis; hence, maximal adaptability is present in times of injury. This process does, however, consume energy and is partially responsible for the elevated resting energy expenditure evident in traumatized patients.
In significantly stressed patients such as those with severe burn injury or with pulmonary insufficiency requiring extracorporeal membrane oxygenation, protein turnover is twice that in normal subjects (Table 6-2). Generally, there is a redistribution of amino acids away from skeletal muscle to the wound, tissues involved in the inflammatory response, and the liver. Acutely needed enzymes, serum proteins, and glucose are thus synthesized. There is also a marked rise in the circulation of hepatically derived acute-phase proteins (ie, fibrinogen, haptoglobin, α1-antitrypsin, transferrin α1-acid glycoprotein, and C-reactive protein) and a concomitant decrease in hepatically derived nutrient transport proteins such as albumin and retinol-binding protein. Although an increase in both whole-body protein synthesis and whole-body protein degradation exists in illness, it is the latter that predominates. Thus, patients after illness manifest net negative protein balance, which clinically may be noted by weight loss, negative nitrogen balance, and skeletal muscle wasting.
One of the driving forces for the catabolism of muscle is the unbridled production of glucose from amino acids (gluconeogenesis). Glucose is the preferred substrate for the brain, red blood cells, and the renal medulla as well as being the most versatile fuel for energy production elsewhere in the body. Interestingly, the provision of dietary glucose alone is relatively ineffective in quelling endogenous glucose production in stressed states. Gluconeogenesis is evident in illness, from adults to premature neonates, and actually appears, on a per-kilogram basis, to be accentuated in low-body-weight patients (presumably because the brain, a glucose-requiring organ, is proportionately larger in this cohort).
In fasting subjects, alanine and glutamine are readily synthesized by muscle and account for over 60% of the total amino acids released from this tissue, and in ill and injured patients, alanine and glutamine transport from muscle is further accentuated. Alanine, by deamination to pyruvate, contributes 3 carbon units to the liver for glucose production, and glutamine serves a dual role as an intestinal energy source and gluconeogenic precursor. Glutamine given intravenously (or enterally) promotes mammalian intestinal growth and development; however, it is not a classically essential nutrient in that it is readily synthesized in adults and neonates. Whether glutamine supplementation may be of benefit in maintaining intestinal integrity in stressed states remains unclear. One study has suggested a decreased incidence of systemic sepsis, although not necrotizing enterocolitis, in enterally fed very low-birth-weight infants when glutamine supplementation was given. The study unfortunately lacked an isonitrogenous control group. About one-half of the glutamine liberated from the muscle is converted to alanine in the intestine and then transported, via the portal vein, to the liver. Hepatic glucose is then produced and released to the bloodstream to become an available energy source for the brain, wound, and even muscle. This interchange between muscle and liver is termed the glucose–alanine cycle. Two further important substrate cycles are present within the liver. Because hepatic gluconeogenesis utilizes the carbon skeletons produced by the deamination of amino acids, the ammonia moiety released must be detoxified by the liver through the urea cycle, which generates urea as a soluble end product. The urea is then able to be safely excreted from the body in the urine. Glycolysis is often activated as a mode of energy production because of the anaerobic environment of some injured tissues. Although this process is less than 10% as efficient as the aerobic oxidation of glucose, its 3-carbon product lactate may be reutilized by the liver by reduction to pyruvate and subsequent resynthesis of glucose. This process is termed the Cori cycle. Figure 6-1 summarizes the effects on endogenous substrate utilization imposed by illness or surgery.
Figure 6-1
Diagrammatic representation of the metabolic response to illness or operation. The endogenous utilization of substrates after injury is characterized by the release of alanine and glutamine from protein breakdown in skeletal muscle and the gastrointestinal tract for subsequent conversion to glucose and acute-phase proteins in the liver. The newly generated glucose can be utilized by those tissues of the body that are glucose-obligate, such as the wound and central nervous system. The by-products of anaerobic metabolism (ie, lactic acid) are then transferred to the liver for reconversion into glucose via the Cori cycle.

Although the catabolism of skeletal muscle to generate glucose is an excellent short-term adaptation in children, it is limited in duration because of the reduced stores available. Without elimination of the inciting stress for catabolism, the progressive loss of diaphragmatic and intercostal muscle leads to respiratory compromise while the loss of cardiac muscle may lead to fatal arrhythmia after approximately one third of the lean body mass dissipates. Fortunately, amino acid nutritional supplementation does improve protein balance, and the mechanism for this change in ill premature neonates appears to be an increase in protein synthesis while protein degradation rates remain constant. The quantity of protein administration required to optimally enhance protein accretion is higher in unwell than in stable children. Infants demonstrate 25% higher protein degradation after surgery, 100% increase in urinary nitrogen excretion with bacterial sepsis, and 100% increase in protein breakdown if they are ill enough to require extracorporeal membrane oxygenation. Children under treatment for cancer also demonstrate increased net protein breakdown.
The quality, or precise amino acid composition, of formulas that best increase whole-body protein balance has yet to be determined, although stable isotope techniques now exist to address this issue. Neonates have immature biosynthetic pathways that alter their capacity for the synthesis of specific amino acids. A conditional essentiality for the amino acid histidine is present until about 6 months of age, and current data suggest that the biosynthetic pathways for cysteine, taurine, and proline may be of limited capacity in the premature neonate. There is little doubt that specific disease states may further accentuate certain amino acid requirements as exemplified by the increased proline demands noted in severe burn injury.
The provision of adequate dietary protein required to maximize protein synthesis, facilitate wound healing and the inflammatory response, as well as preserve skeletal muscle protein mass is the single most important nutritional intervention in ill or postoperative children. The quantity of protein (or amino acid solution) administered should be 3 to 4 g/kg/day for low-birth-weight infants, 2 to 3 g/kg/day for term neonates, and 1.5 g/kg/day for older children. Certain severely stressed states may require additional protein supplementation; hence, growth rates must be monitored closely. Nitrogen balance determination is also clinically useful but has an inherent bias toward apparent protein accrual when none actually exists. Excessive protein administration should be avoided, as toxicity to protein allotments of 6 g/kg/day in neonates has been reported and includes azotemia, pyrexia, a higher incidence of strabismus, and somewhat lower IQ.
Increases in energy requirement during acute illness and following surgery closely parallel the elevated protein needs. Protein retention is augmented by an adequate energy allotment, and this is particularly evident when protein intake is marginal. A careful appraisal of energy requirements in stressed states is required, as both underestimates and overestimates of energy needs are associated with potentially deleterious consequences.
The energy requisites of ill children and neonates are dependent on their resting metabolic rate, degree of illness, physical activity, and need for growth. Formally, resting energy expenditure encompasses the basal metabolic rate plus diet-induced thermogenesis (the heat generated by the consumption of food); however, the latter is quantitatively small even in neonates. Resting energy requirements may be determined directly or indirectly. The direct method measures the heat released by a subject at rest and is based on the principle that all energy is eventually converted to heat. In practice, a subject is placed in a thermally isolated chamber, and the heat dissipated is accurately measured for a prolonged period. Although extremely precise, this technology is not easily applicable to pediatric patients. The indirect methods all rely on the estimation of energy production based on the quantities of O2 consumed and/or CO2 produced by the whole body during a specific time interval. Indirect calorimetry, the most venerable technique, relies on the measurement of VO2 (the volume of oxygen consumed) and VCO2 (the volume of CO2 produced) and usually a correction factor based on urinary nitrogen excretion to calculate the energy production rate. In practice, this involves the creation of a leak-free system, the use of a microcomputer-controlled gas exchange measurement device, termed a metabolic cart, and a 24-hour urinary nitrogen collection. Intubated patients with uncuffed endotracheal tubes and those who are not breathing calmly or regularly pose difficulties. Two newer stable isotope techniques with the advantage of not requiring a leak-free system, doubly labeled water (2H218O), and 13C-labeled bicarbonate, have also been used successfully in pediatric surgical patients to assess total energy expenditure over a period of several days and several hours, respectively. For clinical purposes, all of the indirect techniques provide valuable information in pediatric patients, and their results are highly correlated.
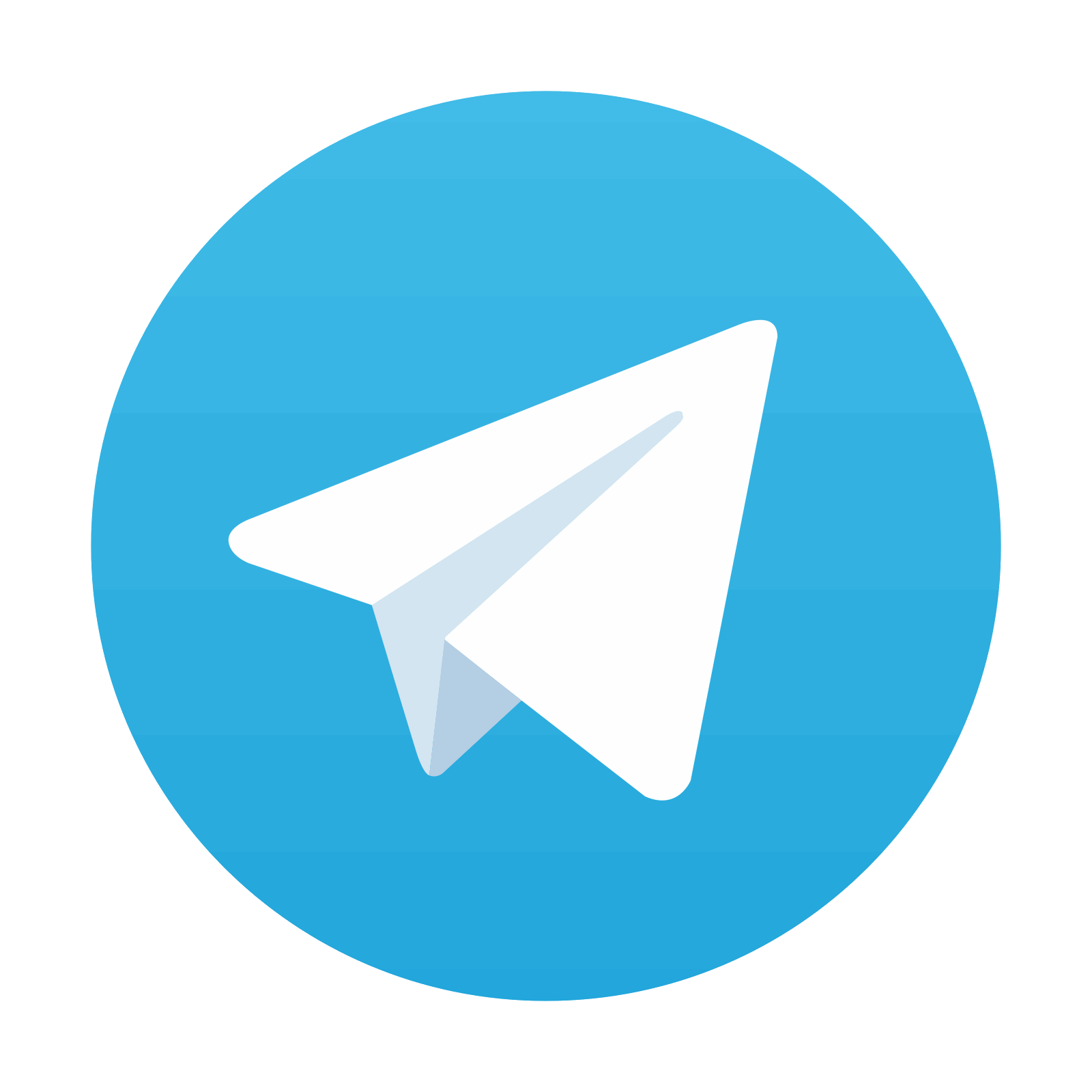
Stay updated, free articles. Join our Telegram channel

Full access? Get Clinical Tree
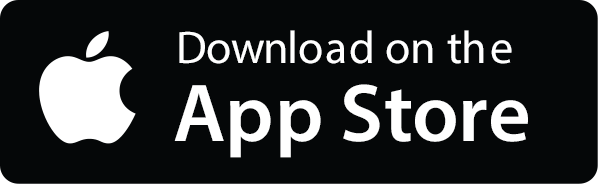
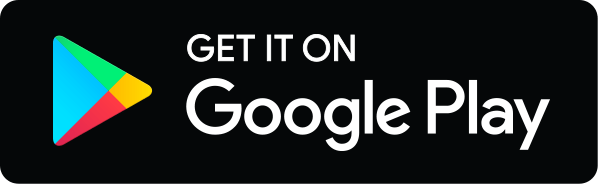