THE NORMAL GROWTH PLATE
The growth plate is an important site of involvement with many of the metabolic bone diseases. The physis, or growth plate, is composed of chondrocytes that are arranged in an orderly fashion. There are 3 histological and functional zones of the physis. The reserve, or germinal cell, zone is adjacent to the epiphysis and contains chondrocyte precursors. This is an important site of regulation of the cellular components of the physis. The proliferative and hypertrophic zones are characterized by the presence of chondrocytes that are organized into columns. The hypertrophic zone is subdivided into zones of maturation (columns of cartilage cells), degeneration, and provisional calcification. The transition between cartilage and bone occurs at the zone of provisional calcification and adjacent portion of the metaphysis. Metaphyseal vessels invade chondrocytes in the portion of the hypertrophic zone closest to the metaphysis. Mineralization of the longitudinal cartilaginous septa of the hypertrophic zone with hydroxyapatite crystal forms a dense but fragile layer, the zone of provisional calcification.
The zones of primary and secondary spongiosa are in the metaphysis immediately adjacent to the zone of provisional calcification of the growth plate. In these layers, osteoblasts ensheath the calcified cartilage columns and produce layers of osteoid. Bone formation occurs by enchondral ossification. There is also resorption of calcified cartilage in the metaphyseal spongiosa. Appropriate osteoclastic activity in the metaphysis is essential for bone modeling. The perichondral region of the metaphysis, which is located at the margin of the physis, is composed of osteoblasts and chondrocytes; this provides circumferential growth of the physis during skeletal maturation.
The growth plate and adjacent portion of the metaphysis are involved in various forms of skeletal system pathology in children. Metaphyseal infractions in abused children occur through the primary spongiosum of the metaphysis. Growth plate injuries, such as slipped capital femoral epiphysis, usually occur within the nonmineralized layer of the hypertrophic zone of the growth plate, adjacent to the zone of provisional calcification. The manifestations of rickets in the growth plate include disorganized cartilage cell growth in the hypertrophic zone, as well as deficient mineralization in the zone of provisional calcification and the metaphyseal spongiosa. Poisoning of osteoclasts in patients with lead intoxication causes failure of normal resorption of mineralized cartilage in the primary spongiosa.
On radiographs, the zone of provisional calcification appears as a thin calcified band along the surface of the metaphysis (Figure 59-1). In young infants, this zone of provisional calcification is of equal or slightly greater density than adjacent spongy bone in the metaphysis. This layer is wider and denser in older children who are experiencing rapid growth. The remainder of the physis is radiolucent. The medullary portion of the metaphysis usually has similar radiodensity as that of the diaphysis. It has the lacy character of overlapping trabeculae.
Figure 59–1
Normal growth plate.
A–C. Wrist radiographs of 3 different children, aged 1 month (A), 17 months (B), and 13 years (C). The zone of provisional calcification is a thin dense band that caps the metaphysis. This band is only faintly dense compared with the metaphysis in “A.” It is thin and well defined in “C.” The spongy bone in the metaphyses has a lacy character.

On MR, 2 zones are visible in the physis. The nonmineralized physeal cartilage, which includes the germinal and proliferative zones and adjacent portion of the hypertrophic zone, produces relatively high signal intensity on T2-weighted, short tau inversion recovery (STIR), and contrast-enhanced T1-weighted images. The adjacent zone of provisional calcification produces relatively low signal intensity. The primary spongiosa of the metaphysis is hyperintense on T2-weighted sequences. Physeal cartilage enhances uniformly with gadolinium and to a greater degree than epiphyseal cartilage.
The cartilaginous epiphysis of the young infant is composed of randomly organized chondrocytes and a cartilage matrix. Chondrocyte hypertrophy and mineralization near the center of the epiphysis form the preossification center. Subsequent invasion by epiphyseal vessels and organization of the hypertrophic chondrocytes into a spherical growth plate occurs. Eventually, the secondary ossification center grows to abut the physis and continues to mature morphologically.
On MR, the epiphysis of the young infant is homogeneous. There is subsequent progressive onset of heterogeneity, which occurs due to several factors. The preossification center produces increased signal intensity in comparison to adjacent epiphyseal cartilage on T2-weighted images. The marrow-containing secondary ossification center also produces relatively high T2 signal intensity. In some children, there are multiple ossification centers. Also, the weight-bearing regions of the cartilaginous epiphyses of the lower extremities normally have somewhat diminished signal intensity on T2-weighted images, apparently due to compression and a diminished amount of fluid. Heterogeneous signal intensity is common in the posterior aspect of the secondary ossification centers of the femoral condyles, due to multiple small centers of ossification.1,2
RICKETS
Rickets results from deficient mineralization of normal osteoid as well as interruption of the normal orderly development and mineralization of the physes in the growing skeleton. There is concomitant lack of normal mineralization of osteoid in mature cortical and spongy bone; this is termed osteomalacia. There are numerous potential causes of rickets and osteomalacia, including abnormalities of vitamin D metabolism, conditions associated with renal tubular phosphate loss, medications (e.g., phenytoin and phenobarbital), and additional rare conditions (Table 59-1). Rickets may result from an absolute deficiency of vitamin D or resistance to the action of vitamin D. The vitamin D–resistant form of rickets is often associated with congenital or acquired renal abnormalities. Vitamin D deficiency can result from nutritional deficiency, malabsorption, or inadequate exposure of the skin to sunlight. A relative deficiency of vitamin D can also occur in patients who have increased requirements for the vitamin. Anticonvulsant drugs accelerate the catabolism of vitamin D. Hypocalcemic rickets can also be caused by diminished renal synthesis of 1,25(OH)2D, which is the physiologically active form of vitamin D.3–6
Vitamin D–resistant (hypophosphatemic) and nutritional rickets are the most common types. Nutritional rickets is more common in infants, whereas renal rickets most often occurs in older children. Infants who are exclusively breastfed are at an elevated risk for nutritional rickets. Radiographic manifestations of rickets are common in low birth weight premature infants; approximately half of infants with a birth weight of less than 1000 g develop radiographic features of rickets. Neonatal rickets can also occur due to maternal vitamin D deficiency, hyperparathyroidism, or renal insufficiency. Vitamin D deficiency in otherwise normal individuals is usually related to inadequate exposure to sunlight in conjunction with a vitamin D–poor diet. Children with anicteric, biliary, or pancreatic disease may suffer poor absorption of dietary vitamin D.6–9
Vitamin D, in conjunction with parathyroid hormone (PTH) and calcitonin, serves to maintain calcium homeostasis. Vitamin D facilitates intestinal absorption and renal retention of calcium and phosphorus. It also modulates osteoblastic function in the skeletal system. Vitamin D is synthesized in the skin from a precursor, 7-deydrocholesterol, in conjunction with exposure to sunlight. There are multiple metabolites of vitamin D. In the liver, vitamin D is metabolized to 25-hydroxy vitamin D (25-OHD), which is the most abundant metabolite in the circulation. Conversion of 25-OHD to 1,25-dihydroxy vitamin D (calcitriol) occurs in the kidneys.10
An early clinical sign of rickets in infants is craniotabes, which refers to thinning of the outer table of the calvaria. Firm palpation over the occiput or posterior parietal squamosa results in a ping-pong ball sensation. Premature infants and low birth weight infants are especially prone to developing craniotabes. Older infants with rickets have delayed closure of cranial sutures. The frontal bone may be thickened, leading to bossing. Palpable enlargement of the costochondral junctions is termed the rachitic rosary. There may be palpable enlargement of the wrists, ankles, and knees. Serum blood chemistry abnormalities are present for weeks or months prior to the development of overt clinical and radiographic manifestations of rickets. Potential systemic manifestations of vitamin D deficiency include anorexia, muscular weakness, hypocalcemic seizures, and increased susceptibility to infection. Rickets causes bone softening; children with long-standing rickets who are weight bearing may develop bowing of the lower extremity long bones. The treatment of rickets is dietary and medical, with surgery reserved for children with severe deformities.11,12
In children with rickets, the resting and proliferating zones of the physis are essentially normal. The zone of maturation in the hypertrophic region is widened due to a disorganized increase in the number of cells and loss of the normal orderly columnar cellular pattern. Calcification of the cartilaginous bars in the zone of provisional calcification is deficient. There is also defective mineralization in the zone of primary spongiosa. Widening and cupping of the metaphysis in patients with rickets are predominantly due to chaotic cartilage cell growth in the zone of maturation. The mass of cartilage bulges outward and protrudes in the central area of the metaphysis. Stress at the sites of ligament attachments may also contribute to cupping. Occasionally, a thin bony rim extends from the metaphysis along the peripheral margin of the cartilage mass; this is due to periosteal intramembranous new bone.
The pathophysiological hallmark of osteomalacia is lack of normal mineralization of osteoid. Inadequately mineralized osteoid in these patients coats the surfaces of bone trabeculae. The number of trabeculae diminishes, and those that remain are thin. Prominent osteoid extends along the Haversian canals of the cortex, creating “osteoid seams.” There may also be excessive osteoid deposition in the subperiosteal regions.
The predominant radiographic manifestations of rickets are in the metaphyses, with widening of the zone of provisional calcification due to the presence of unmineralized osteoid. Cupping, fraying, and splaying of metaphyses occur. The most prominent radiographic manifestations of rickets occur in areas of fastest bone growth, including the proximal ends of the humerus and tibia, the distal ends of the femur, radius, and ulna, and the sternal ends of the ribs. The earliest radiographic finding is growth plate irregularity, that is, poor definition of the zone of provisional calcification (Figure 59-2). In normal children, the zone of provisional calcification is sharp and slightly denser than the adjacent metaphyseal medullary bone (Figure 59-1). In children with rickets, deficient mineralization in the zone of provisional calcification and cellular proliferation in the hypertrophic zone lead to physeal widening, as well as cupping, fraying, and irregularity of the adjacent portion of the metaphysis (Figure 59-3). With advanced rickets, the subphyseal portion of the metaphysis is unmineralized. The epiphyses are usually small and there may be loss of radiographic definition of the ossification centers.
Figure 59–3
Rickets.
A–E. Radiographic manifestations of vitamin D deficiency in 5 different children. There is widening of the growth plates (although part of the lucency is due to deficient mineralization of the metaphyses). The metaphyses are irregular, wide, and variably cupped. There is poor definition of the epiphyses in “B.” Note periosteal new bone formation along the metacarpal shafts in “D.”


The severity of the radiographic manifestations of rickets in the shafts of the long bones slightly lags that of the metaphyses and growth plates. Typically, there is coarse demineralization of the entire bone; this finding helps in the differentiation from metaphyseal dysplasias. The cortex is thin and the cortical margins are indistinct. The spongiosum of the metaphysis develops a coarse appearance due to demineralization and resorption of secondary trabeculae. The weakened bones are susceptible to fracture (Figure 59-4). Osteomalacia in these children may result in radiolucent transverse bands in the diaphyses of the long bones, that is, Looser zones. With long-standing disease, the long bones may become bowed. With severe rickets of long duration, there is accumulation of unmineralized or poorly mineralized subperiosteal osteoid (Figure 59-5).13
Figure 59–5
Rickets.
This 2-year-old child suffered severe nutritional deprivation. There is severe osteopenia. The trabecular pattern is coarse. Deficient physeal and metaphyseal mineralization results in apparent widening of the growth plates. A Looser zone (arrow) is present in the tibia. There is also a faint linear lucency in the bowed fibula. Poorly mineralized subperiosteal osteoid extends along the bone shafts.

Pathology | Radiology |
---|---|
Lack of calcification of cartilage and osteoid |
Widening of the growth plate Irregularity and diminished density of the zone of provisional calcification |
Disorganized physeal cartilage cell growth |
Metaphyseal cupping Growth plate widening Rachitic rosary |
Osteomalacia |
Osteopenia Coarsened trabecular pattern Bowing, fracture, loss of vertebral body heights |
Secondary hyperparathyroidism |
Subperiosteal bone resorption Intracortical tunneling Generalized demineralization |
Chest radiographs of children with rickets often show flaring and fraying of the anterior rib ends (Figure 59-6). The inferior scapular angles are concave and irregular. There is cupping of the proximal humeral metaphyses. Skull radiographs show poor calvarial mineralization. There is deficient mineralization of the teeth (Figure 59-7). Delayed eruption of the teeth may be noted clinically. The margins of the carpal and tarsal bones become ill-defined and there is demineralization of the spongiosum.
In those forms of rickets that are calcipenic, including vitamin D–deficient rickets, there is compensatory elevation of PTH levels. This may lead to radiographic features of secondary hyperparathyroidism superimposed on those of rickets. The major effect of elevated PTH levels is bone resorption. Subperiosteal bone resorption leads to poor cortical definition that can progress to irregularity or frank destruction. Irregular radiolucent areas may occur in the subphyseal portions of the metaphyses. Occasionally, elevation of PTH results in paradoxical periosteal new bone formation.
The earliest radiographic manifestation of healing of rickets is the reappearance of the normal zone of provisional calcification. The zone of provisional calcification becomes sclerotic, producing a transverse band that widens with time (Figure 59-8). Additional mineralization within the portion of the metaphysis between the zone of provisional calcification and the bone shaft (the zones of primary and secondary spongiosa) occurs more slowly. The metaphyseal borders progressively become better defined and ossification occurs in the subperiosteal regions. Unless there is severe disease, bone remodeling usually restores normal morphology with time.
Figure 59–8
Healing rickets.
A forearm radiograph obtained 3 weeks into dietary supplementation therapy for nutritional rickets shows moderate sclerosis at the metaphyseal ends (with some residual cupping) and periosteal new bone formation along the bone shafts. Note residual osteopenia in the primary and secondary spongiosa of the distal radius and ulna.

HYPOPHOSPHATEMIC RICKETS
Hypophosphatemic rickets is due to renal wasting of phosphate, which is a crucial component of bone. Vitamin D deficiency is not a component of this variety. Most forms of hypophosphatemic rickets are due to genetic defects. Most common is familial hypophosphatemic rickets, which is an X-linked dominant disorder. Other forms of hypophosphatemic rickets include autosomal dominant hypophosphatemic rickets that is caused by mutations in FGF23 on chromosome 12p13 encoding a member of the fibroblast growth factor (FGF) family, an autosomal recessive form caused by mutations in the DMP1 gene, an X-linked recessive form caused by mutations in the CLCN5 gene, and a form of hypophosphatemic rickets with hypercalciuria caused by mutations in the SLC34A3 gene. Hypophosphatemic rickets can occur with various other forms of renal tubular disease, including cystinosis, glycogenosis, tyrosinemia, Lowe syndrome, Wilson disease, galactosemia, and toxin-related damage. Oncogenic rickets is a rare form of hypophosphatemic rickets and osteomalacia that can occur in association with various neoplasms, such as hemangiopericytoma, osteoid osteoma, osteosarcoma, giant cell tumor, and chondrosarcoma. Other associations include fibrous bone lesions, neurofibromatosis, epidermal nevus syndrome, and McCune-Albright syndrome.14,15
Familial hypophosphatemic rickets (hereditary vitamin D–resistant rickets; X-linked hypophosphatemia) is a dominant X-linked disorder that is characterized by impaired resorption of phosphate in the proximal tubules, resulting in hypophosphatemia, poor bone mineralization, normocalcemic rickets, and short stature. The major cause of this disorder is a defect in the PHEX gene on chromosome X. The genetic defect results in diminished production of the metalloprotease PHEX within osteoblasts and other cells. Loss-of-function mutations in PHEX cause renal phosphate wasting indirectly, through a humoral factor (“phosphatonin”). The humoral factor apparently is fibroblast growth factor-23 (FGF23); elevated blood levels of this growth factor lower the renal phosphate threshold by inhibiting resorption of phosphate in the proximal tubules. Loss-of-function mutations in PHEX also affect bone mineralization by allowing an increase in the concentration of a mineralization inhibitor (“minhibin”) in bone. The prevalence of this form of rickets is 1 in 20,000 births. This is a proportionately common form of rickets in developed countries. The rare autosomal dominant variety of hypophosphatemic rickets is due to a gain-of-function mutation of FGF23 that prevents appropriate regulation by normal circulating PHEX. Oncogenic rickets is due to tumor production of FGF23 and/or minhibin. Genetic testing for mutations in PHEX or FGF23 is available.16–18
The clinical onset of familial hypophosphatemic rickets usually is later in childhood than is typical of nutritional rickets. There is considerable variability in the severity of disease, even among siblings. Most affected patients are of short stature and have bowing deformities of the lower extremities. The severity of the disease is usually less pronounced in female heterozygotes than in male hemizygotes.
Radiographs of children with familial hypophosphatemic rickets most often show mild to moderate rachitic skeletal abnormalities. There is progressive coarsening of the osseus trabecular pattern. Because these children are normocalcemic, there are typically no manifestations of secondary hyperparathyroidism in untreated patients. There are usually prominent bowing deformities. Cortical thickening occurs along the concave sides of the bowed tubular bones (Figure 59-9). Osteomalacic pseudofractures, or Looser zones, can occur. These are linear radiolucent (unmineralized osteoid) bands oriented perpendicular to the bone, with slight adjacent sclerosis. The most common sites of Looser zones are the femoral neck, ulna, scapula, and pubic ramus. The lesions are often bilateral and symmetric. Enthesopathy is a characteristic feature of hypophosphatemic rickets in adults. Generalized increase in bone density (especially in the axial skeleton) can occur in adults with this disorder. Spinal stenosis occurs due to ossification of the ligamentum flavum, facet joint hypertrophy, and laminar thickening.
Medical treatment with vitamin D analogs (calcitriol) and oral phosphate supplementation can lead to nephrocalcinosis (predominantly in the pyramids) (Figure 59-10), soft tissue calcifications, cortical thickening of long bones, radiodense metaphyseal bands, sclerotic vertebral end plates, and calvarial thickening. Growth hormone therapy mitigates short stature in selected patients with hypophosphatemic rickets.19–21
Figure 59–10
Familial hypophosphatemic rickets.
A. A lower extremity radiograph of a 2.5-year-old girl who has been treated with calcitriol and phosphate since diagnosis at age 16 months shows trabecular coarsening, coxa vara, mild long bone bowing, and metaphyseal widening/cupping. B. At 5 years of age, there has been progression of lower extremity bowing despite appropriate medical therapy. C. Sonography shows hyperechogenicity of the renal pyramids, indicating therapy-related nephrocalcinosis.


HYPERPARATHYROIDISM
Hyperparathyroidism refers to excessive production of parathyroid hormone (PTH), which is a key modulator of calcium homeostasis. PTH stimulates osteoclast-mediated bone resorption and promotes renal hydroxylation of 25-OHD. PTH also promotes renal tubular reabsorption of calcium and induces excretion of phosphate into the urine. The major effect of excess circulating PTH is stimulation of osteocytic osteolysis. With severe disease, there is replacement of medullary bone with vascular fibrous tissue, that is, osteitis fibrosa cystica.
Hyperparathyroidism occurs in primary, secondary, and tertiary forms. Primary hyperparathyroidism is due to autonomous hypersecretion of PTH; approximately 80% of these patients have a parathyroid adenoma, 20% have parathyroid gland hyperplasia, and there are rare instances of secretion by a carcinoma. Approximately 20% of patients with a nonfamilial hyperparathyroid adenoma have a somatic MEN1 mutation. The cyclin D1/PRAD1 gene has also been implicated in some patients. In current practice, most patients with primary hyperparathyroidism are diagnosed and treated prior to the onset of overt symptoms or substantial radiographic alterations. The most common reported symptoms are weakness and easy fatigability.22,23
Secondary hyperparathyroidism is due to: (1) stimulation of the parathyroid glands in response to hypocalcemia or (2) dysregulation on the normal negative feedback loop, with insensitivity of the parathyroid glands to elevated serum calcium levels (pseudohypoparathyroidism). Tertiary hyperparathyroidism refers to patients with secondary hyperparathyroidism who develop autonomous function of the parathyroid glands despite correction of the underlying cause of hyperparathyroidism; hypersecretion of PTH in these patients occurs despite normal calcium levels.
Chronic renal failure is the most common cause of secondary hyperparathyroidism. The 3 major factors in the pathogenesis of renal hyperparathyroidism are hypocalcemia, hyperphosphatemia, and low 1,25(OH)2D3 levels. In the normal parathyroid glands, there is a feedback mechanism by which serum calcium activates the calcium receptor in parathyroid cells and causes decreased PTH secretion. With hypercalcemia, the receptors are inactivated and PTH is secreted. In patients with renal hyperparathyroidism, the calcium receptor protein is downregulated, and the efficiency of inhibition of PTH in response to elevation of calcium is diminished. An additional factor in these patients is elevation of serum phosphate, which inhibits the release of intracellular calcium from internal cellular stores (e.g., mitochondria) and thereby increases PTH secretion. A third pathogenic factor is stimulation of PTH mRNA transcription in response to low levels of 1,25(OH)2D3. With long-term stimulation of PTH synthesis and secretion, there is proliferation of parathyroid cells and a hyperfunctioning gland results.19
Radiographic studies of patients with hyperparathyroidism may show generalized osteopenia, often with a coarsened trabecular pattern. A characteristic finding is subperiosteal bone resorption, usually most pronounced in the hands and feet. Classically, the earliest site of subperiosteal bone resorption is along the radial aspects of the second and third middle phalanges (Figure 59-11). The lamina dura of the teeth is another site of the early radiographic alteration. Terminal tuft resorption may occur. Prominent subperiosteal resorption occasionally occurs along the medial surfaces of the femoral and humeral necks, the medial aspects of the proximal tibial metaphyses, the lamina dura, and at sites of tendon and ligament insertions (Figure 59-12). In children with open growth plates, resorption can occur in the zone of provisional calcification and adjacent metaphyseal spongiosa. This may resemble the findings of rickets on radiographic evaluation, but the lucent area consists of fibrous tissue rather than cartilage and osteoid.24
Diffuse or localized osteosclerosis sometimes occurs in patients with secondary hyperparathyroidism, probably due to a stimulatory effect of PTH on osteoblasts. Sclerosis tends to be most prominent in the axial skeleton. Subchondral vertebral body osteosclerosis in these patients can produce a striped appearance, termed the “rugger jersey spine.” Soft tissue calcification and periostitis also can occur in patients with the secondary form of hyperparathyroidism.
Brown tumors (osteoclastomas) are masses of osteoclastic and fibrous tissue that can produce eccentrically located expansile bone lesions in patients with hyperparathyroidism and renal osteodystrophy. These lesions are somewhat more common with primary hyperparathyroidism than with other types. Hemorrhagic bone cysts can also occur in patients with hyperparathyroidism; the radiographic appearance is identical to that of a brown tumor (i.e., a well-demarcated radiolucent defect). Some of these cystic lesions likely represent necrotic brown tumors. A brown tumor usually has prominent uptake on skeletal scintigraphy, whereas a bone cyst is not detectable or appears photopenic. CT and MR also show the soft tissue composition of a brown tumor, including contrast enhancement. Brown tumors and hemorrhagic bone cysts can be solitary or multiple, eccentric or central, and small or large (Figure 59-13). They can occur in the axial or appendicular portions of the skeleton.
Congenital primary hyperparathyroidism is a rare autosomal recessive disorder that usually presents during infancy. Potential clinical manifestations include hypotonia, respiratory distress, lethargy, fever, and vomiting. Physical examination may demonstrate hepatosplenomegaly and craniotabes. Most patients have hypercalcemia. Radiographic examination usually demonstrates marked features of hyperparathyroidism, with subperiosteal resorption, osteopenia, a coarsened trabecular pattern, tubular bone erosions, periosteal new bone formation, and pathological fractures.
RENAL DISEASE
Long-term kidney diseases have important effects on the skeletal system. Appropriate renal function is crucial for calcium and phosphorus homeostasis. In addition, conversion of vitamin D to the metabolically active form 1,25-dihydroxy vitamin D occurs in the kidneys. The major osseous consequences of chronic renal insufficiency include secondary hyperparathyroidism, rickets and osteomalacia, and osteoporosis. Renal diseases can be divided into those that predominantly affect the tubules, glomeruli, or both. Renal tubular disorders are often subdivided into those predominantly involving the proximal renal tubules, the distal renal tubules, or combinations of the 2. Important functions of the proximal tubules include absorption of inorganic phosphate, glucose, and amino acids. Acidification and concentration of urine occur in the distal tubules.
The constellation of clinical alterations that occur with functional defects of the proximal convoluted tubules is termed Fanconi syndrome. Fanconi syndrome encompasses multiple congenital and acquired disorders, including inborn errors of metabolism and exposure to heavy metals (Table 59-2). Deficient proximal tubule transport function results in hypophosphatemia, hypokalemia, and hypochloremic metabolic acidosis. There are elevated urinary levels of glucose, amino acids, and phosphate. Hypophosphatemia, with or without the added effect of metabolic acidosis, leads to rickets or osteomalacia. Impaired renal conversion to the biologically active form of vitamin D contributes to the metabolic bone disease in these patients.25
Inherited disorders | Cystinosis |
Primary (idiopathic) | |
Tyrosinemia | |
Glycogen storage disease | |
Wilson disease | |
Galactosemia | |
Metachromatic leukodystrophy | |
Lowe syndrome | |
Pyruvate carboxylase deficiency | |
Toxins | Heavy metal poisoning |
Aminoglycoside | |
Ifosfamide | |
Outdated tetracycline | |
Acquired disorders | Vitamin D deficiency |
Dysproteinemia | |
Amyloidosis |
Idiopathic (primary) Fanconi syndrome occurs with a bimodal prevalence pattern. Most children with this disorder have a clinical onset during the first 6 months of life, whereas adult-onset disease tends to develop during the third or fourth decade. Infants with Fanconi syndrome may present with vomiting, polydipsia, polyuria, and constipation. There is failure to thrive. Episodes of dehydration and metabolic acidosis may occur. The major radiographic findings of Fanconi syndrome are those of rickets in children and osteomalacia in adults (Figure 59-14). If the underlying renal disease progresses to the point of glomerular dysfunction, radiographic manifestations of secondary hyperparathyroidism develop, and the imaging appearance is that of renal osteodystrophy. These patients may have short stature, bowing of tubular bones, and pathological growth plate fractures.
Figure 59–14
Fanconi syndrome.
A. A hand radiograph of an 11-year-old child with primary Fanconi syndrome shows generalized osteopenia. There is slight undergrowth of the carpal bones. B. DEXA evaluation of the right hip confirms subnormal bone mineral density. The femoral neck Z-score was -3.4. C. A longitudinal sonographic image of the right kidney shows nephromegaly, prominent parenchymal echogenicity, and medullary nephrocalcinosis.

Cystinosis is an autosomal recessive disorder in which there is abnormal lysosomal accumulation of cystine. Without appropriate therapy, clinical manifestations of Fanconi syndrome develop around 6 months of age. The clinical features include polyuria, polydipsia, constipation, fever, and failure to thrive. Loss of phosphate in the urine leads to hypophosphatemic rickets. In the absence of appropriate therapy (cysteamine), most children with cystinosis suffer progressive renal failure that leads to end-stage renal disease by approximately 10 years of age. Potential consequences of cystinosis in older children include hypothyroidism, diabetes mellitus, corneal disease, retinopathy, and cerebral atrophy.26
Renal osteodystrophy refers to a constellation of skeletal system abnormalities that occur in patients with chronic renal insufficiency. Alterations in mineral metabolism and bone structure occur in nearly all patients with significant chronic kidney disease. The pathophysiology of renal osteodystrophy is complex. In general, renal tubular diseases cause excessive loss of phosphate in the urine, which in turn leads to rickets or osteomalacia. Renal glomerular diseases lead to diminished renal phosphate excretion and deficient vitamin D–mediated intestinal absorption of calcium; the resultant high serum phosphate and low serum calcium stimulate the excretion of PTH. Severe kidney disease also affects metabolism of calcium and phosphate because of impaired renal synthesis of biologically active vitamin D (calcitriol). Children with renal disease may not respond appropriately to exogenously administered vitamin D; this vitamin D–resistant disorder is termed renal rickets. However, most of the skeletal alterations in children with renal rickets are due to the effects of excess PTH.27
Serum phosphate levels rise in patients with renal osteodystrophy, due to impaired glomerular filtration. This leads to hypocalcemia and the stimulation of PTH release. When the glomerular filtration rate falls to approximately 25% to 30% of normal, the phosphaturic renal response to PTH is lost, and secondary hyperparathyroidism develops. The elevated PTH levels lead to excessive osteoclastic resorption of bone. The severity of renal osteodystrophy is greatest in children who have onset of chronic renal failure at a young age. The severity also relates to the duration of renal failure. Failure to thrive and deficient longitudinal growth are early clinical signs of renal osteodystrophy.
The radiographic features of renal osteodystrophy in children include rickets/osteomalacia, secondary hyperparathyroidism, osteosclerosis, and soft tissue calcifications (Figure 59-15). Radiographic skeletal alterations may be visible within 2 months of the onset of renal dysfunction in the young child, but often require years of renal disease before becoming apparent in adolescents or adults. As with other forms of rickets, important skeletal findings in affected children are due to the presence of noncalcified osteoid. Radiographs show widening and irregularity of the growth plates, best visualized at the ends of rapidly growing bones. Metaphyseal cupping, tubular bone bowing, and rachitic rosary are also common. The bone age is delayed. The radiographic findings in these patients are similar to those of nutritional rickets, but tend to be more pronounced.28,29
Figure 59–15
Renal osteodystrophy.
This child has chronic renal insufficiency due to posterior urethral valves. A. A knee radiograph shows manifestations of rickets, with growth plate widening and irregularity along the metaphyseal margins. B. Medullary osteopenia and lack of normal trabeculae are visible on this hand radiograph. Growth plate/metaphyseal abnormalities are visible in the wrist.

The radiographic manifestations of secondary hyperparathyroidism in children with renal osteodystrophy are similar to those of other forms of hyperparathyroidism. However, the findings tend to be more severe in the secondary form than in the primary form. Common findings include subperiosteal bone resorption and osteopenia. The most prominent sites of subperiosteal resorption in older children and adults are the radial aspects of the middle and proximal phalanges of the index and middle fingers (Figure 59-16). Radiographs of the hands may show distal tuft resorption. In infants, the femoral necks, the medial aspects of the proximal tibias, and the humeral necks are common sites of radiographically demonstrable subperiosteal bone resorption. Children with renal osteodystrophy are prone to slipped epiphyses and metaphyseal fractures. Brown tumors can occur. Osteosclerosis is more common with the secondary form of hyperparathyroidism than with other varieties (Figure 59-17). Osteosclerosis is the predominant radiographic finding in some patients with renal osteodystrophy.
Figure 59–16
Renal osteodystrophy; secondary hyperparathyroidism.
An oblique view of the index finger shows cortical irregularity (small arrows) along the radial margins of the phalanges, due to subperiosteal resorption. A small brown tumor results in a subtle oval radiolucency in the proximal phalanx (large arrow). There is also demineralization of the distal tuft.

Pathology | Radiology |
---|---|
2° hyperparathyroidism | Subperiosteal bone resorption |
Intracortical resorption (tunneling) | |
Endosteal resorption | |
Subchondral, subligamentous, subtendinous resorption | |
Osteitis fibrosa | |
Brown tumors | |
Slipped epiphysis | |
Osteosclerosis | Thickened trabeculae |
Rugger jersey spine | |
Rickets/osteomalacia | Osteopenia |
Looser zones | |
Wide, irregular growth plates | |
Metaphyseal cupping | |
Soft tissue calcification | Vascular, periarticular, visceral |
The pathophysiology of osteosclerosis in patients with renal osteodystrophy is incompletely understood. Proposed mechanisms include healing of hyperparathyroid lesions and the effects of calcitonin. Radiographs show thickening and fusion of bone trabeculae. There is often an overall increase in bone density. The trabeculae may be indistinct and have a smudged appearance. In children, osteosclerosis is usually most pronounced in the metaphyses, sometimes appearing as radiodense bands. Older children frequently have vertebral end plate involvement, resulting in the rugger jersey spine pattern. Other common sites of osteosclerosis include the pelvis, ribs, skull, and tubular bones.
Abnormal periosteal new bone formation occurs in some patients with renal osteodystrophy. Proliferation of fibrous tissue at the site of subperiosteal bone resorption can lead to elevation of the periosteum; the resultant new bone formation is termed periosteal neostosis. A characteristic radiographic feature of this form of periosteal bone formation is the presence of a radiolucent stripe of fibrous tissue that separates the new periosteal bone from the underlying cortex.
Soft tissue calcification is an occasional finding in children with renal osteodystrophy and secondary hyperparathyroidism. The calcifications may be subcutaneous or vascular. Chondrocalcinosis and visceral calcifications can also occur.
SCURVY
Scurvy is a metabolic bone disease due to deficiency of vitamin C. It is exceedingly rare in the United States, but still occurs in some developing nations. Clinical presentation only rarely occurs in children younger than 6 months of age. Common clinical manifestations include irritability, tachypnea, and anorexia. The irritability is progressive and there may be findings of musculoskeletal tenderness, particularly in the lower extremities. Scurvy is 1 of several potential causes of pseudoparalysis in infants. There is a propensity for hemorrhage in the skin, mucous membranes, and subperiosteal regions; this may be related to capillary endothelial collagen deficiency.30
The radiographic diagnosis of scurvy is generally based on the appearance of the long bones, especially in the regions of the knees. There is generalized osteopenia, but preservation of calcification of cartilage in the zone of provisional calcification. There is diminished or absent resorption of calcified cartilage in the metaphyseal spongiosa. The dense and widened zone of provisional calcification appears as a radiodense transverse line at the junction of the physis and metaphyses, termed the white line of Fränkel. An additional important finding is a radiolucent horizontal band of variable thickness adjacent to the zone of provisional calcification; this is termed the scurvy zone or Trümmerfeld zone (Figure 59-18).
Pathological fractures can occur through the weekend metaphyses of children with scurvy, sometimes mimicking the radiographic appearance of child abuse. These fractures typically occur through the spongiosa, subjacent to the zone of provisional calcification (Figure 59-19). When confined to the peripheral aspect of the metaphysis, a fracture through the scurvy zone can produce the corner sign or angle sign. Displacement of the dense zone of provisional calcification can produce Pelkan spurs (Figure 59-20). The zone of provisional calcification is brittle and subject to fractures as well, sometimes “crumpling” into the subjacent weakened metaphysis. The osteopenic epiphysis is outlined by a fine radiodense peripheral margin of calcified cartilage, termed the Wimberger ring. There is hypodensity in the subjacent spongy bone, analogous to the scurvy zone of the metaphysis. The carpal and tarsal bones also have radiodense outer margins of calcified cartilage (Figure 59-21). Children with scurvy are prone to subperiosteal hemorrhage, which leads to extensive periosteal new bone formation.
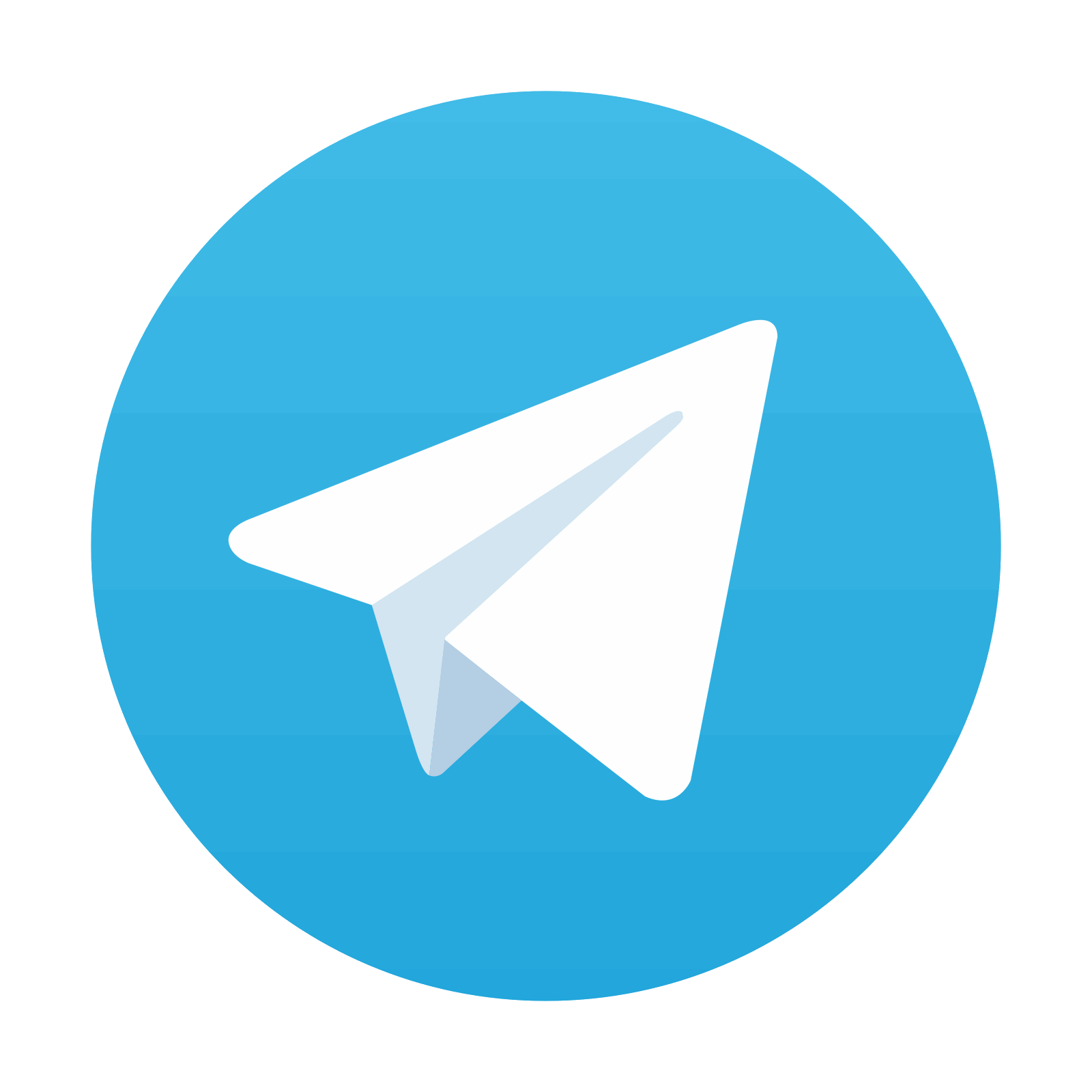
Stay updated, free articles. Join our Telegram channel

Full access? Get Clinical Tree
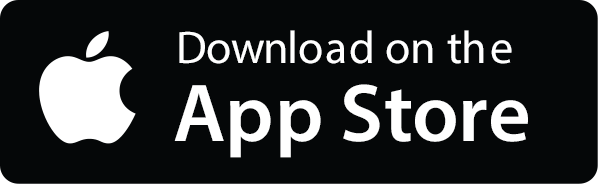
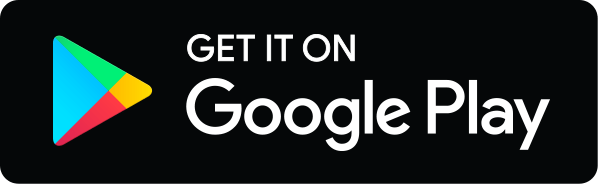