As more women breastfeed and breastfeed longer in keeping with the World Health Organization (WHO) and the American Academy of Pediatrics (AAP) recommendations, questions about the safety of certain medications increase. A newer, excellent resource for information about drugs during lactation has been developed with the advice from an expert panel for the National Library of Medicine called LactMed. It is a drugs and lactation database, a peer-reviewed and fully referenced database of possible drugs used during lactation. The data include maternal and infant levels of drugs, possible effects on nurslings and on lactation itself, and a list of alternative drugs. The address is http://toxnet.nlm.nih.gov.easyaccess2.lib.cuhk.edu.hk/cgi-bin/sis/htmlgen?LACT .
With the plethora of resources about drugs, many of which are available to the lay person and mother herself, there is the risk for an untrained person misinterpreting the data. , , A major problem is even the professional untrained in lactation physiology who offers medical advice based on information gleaned from these resources. A professional needs to understand not just the plasma and milk levels but the pharmacology of the drug and physiology of lactation to give helpful instructions that will mitigate the effect of the drug on an infant and avoid discontinuing breastfeeding unnecessarily.
Despite the overwhelming advantages of human milk and the advantages of being breastfed, at times the risk of a maternal medication adversely affecting a nursing infant must be considered. Even when data about the medication, such as the milk/plasma ratio, are available, a physician has to consider several factors related to each infant and each situation before deciding if breastfeeding can be initiated or continued. The more complicated a mother’s medical problems, the greater the possibility that the infant also has complications of prematurity or illness that will alter the ability to excrete the medication. This situation requires scientific information and experienced clinical judgment to appraise the problems and determine the therapeutic regimen. The clinician must determine the risk/benefit ratio of continued breastfeeding. The data are meager and sometimes conflicting for some drugs, yet maternal medication is the single most common medical problem in managing breastfeeding patients reported to the Breastfeeding and Lactation Center.
There are a number of general reviews of drugs in breast milk and hundreds of articles about the effect of a specific medication in a particular infant. The AAP Committee on Drugs has published a list of drugs and other chemicals that transfer into human breast milk. The list, which is continually updated, is divided into those that are contraindicated, those that require temporary interruption of breastfeeding, and those that are compatible with breastfeeding. Concern about the issue of drugs in breast milk has spread. The U.S. Department of Health and Human Services and the Food and Drug Administration (FDA) have proposed a standard warning on all nonprescription drugs that are absorbed by the body: “As with any drug, if you are pregnant or nursing a baby, seek professional advice before using this product.” Because studies of pregnant women have shown that they take five to eight medications on their own during pregnancy and postpartum, clinicians’ education of these patients needs to continue.
A study of more than 14,000 pregnant women in 148 hospitals in 22 countries revealed that 79% of women received an average of 3.3 drugs. The drugs most often given were analgesics and anesthetics. Of the 91% of women who initiated breastfeeding, 36% received methylergonovine and 5% antibiotics. Another study of 885 women 3 to 5 months postpartum in Oslo showed that breastfeeding women took fewer medications (daily dose/1000 women/day) than nonbreastfeeding women.
The most common medication in the latter group was oral contraceptives. Colds, dyspepsia, hemorrhoids, and breast infections were the disorders that precipitated the use of albuterol (salbutamol), clemastine fumarate (Tavist), dexchlorpheniramine maleate (cold preparations), phenylpropanolamine hydrochloride (Comtrex, Dimetane), cromolyn sodium, and methotrimeprazine hydrochloride (levomepromazine).
No substitute exists for specific knowledge. It is equally inappropriate to discontinue breastfeeding when it is not medically necessary as it is to continue breastfeeding while taking contraindicated drugs.
Consideration of the pharmacokinetics contributes to the understanding of the problems involved. Some reported data have been extrapolated from experiments performed on cows, goats, and rodents. Bovine experiments have been conducted using continuous infusions, which provide data on the passage of a drug into milk under certain pH and plasma levels. In an effort to explain and clarify the issues involved, the literature has oversimplified the problem so that individuals lacking a background in pharmacology or pediatrics have misused the published data to draw unwarranted conclusions.
Factors that influence the passage of a drug into the milk in humans include the size of the molecule, its solubility in lipids and water, whether it binds to protein, the drug’s pH, and diffusion rates. The following outline summarizes these factors:
- I.
Drug
- A.
Route of administration
- 1.
Oral
- 2.
Intravenous (IV)
- 3.
Intramuscular (IM)
- 4.
Transdermal drug delivery system (TDDS)
- 1.
- B.
Absorption rate
- C.
Half-life or peak serum time
- D.
Dissociation constant
- E.
Volume of distribution
- A.
- II.
Size of molecule
- III.
Degree of ionization
- IV.
pH of substrate
- A.
Plasma: 7.4
- B.
Milk: 6.8
- A.
- V.
Solubility
- A.
In water
- B.
In lipids
- A.
- VI.
Protein binding more to plasma than to milk protein
Passive diffusion is the principal factor in the passage of a drug from plasma into milk. The drug may appear in an active form or as an inactive metabolite.
Finally, a most important factor that has received relatively little attention is the infant. Will the infant absorb the chemical from the intestinal tract? If the infant absorbs the chemical, can the infant detoxify and excrete it, or will minimal amounts in the milk build in the infant’s system? Is the infant premature, small for gestational age, or high risk because of complications of the pregnancy or delivery? Is the drug a material that could be safely given to an infant directly, and at what risk? What dosages and blood levels are safe? These latter two questions are more critical than the pharmacokinetic theory. The ultimate question faced by the physician is, “Can this infant be safely exposed to this chemical as it appears in breast milk without a risk that exceeds the benefits of being breastfed?” Almost any drug present in a mother’s blood will appear to some degree in her milk.
Characteristics of Drugs
Protein Binding
Drugs entering the circulation become protein bound or remain free in the circulation. The protein-bound component of the drug serves as an inactive reservoir for the drug that is in equilibrium with the free drug. Most drugs enter the mammary alveolar cells in the unbound form ( Figure 12-1 ).

At term, plasma proteins may be reduced and the fatty acid and hypoprotein fraction slightly increased in the mother, which results in the displacement of some drugs from plasma proteins. During the early postpartum period, for 5 to 7 weeks the free fraction of some drugs increases and therefore more readily crosses into milk (e.g., salicylate, phenytoin, diazepam).
For most drugs, a higher concentration will be found in the plasma than in the milk. Only the small free fraction of drug can cross the biologic membrane. The total concentration in milk is only minimally influenced by binding of drugs in milk proteins (milk protein concentration is 0.9% in mature milk). Only those drug molecules that are free in solution can pass through the endothelial pores, either by diffusion or by reverse pinocytosis. Pinocytosis is the process whereby drug molecules dissolved in the interstitial fluid attach to receptors located at the surface of the cell membrane. The cell membrane invaginates at the site of the drug attachment, bringing the drug into the cell. The membrane is pinched off, and the drug, surrounded by membrane, remains in the cell. Then the membrane is dissolved, leaving the drug molecule free in the cell.
Reverse pinocytosis is the process by which the apical membrane evaginates after fusion of the intracellular membrane-bound secretion granules with the plasma membrane. The granules include lipids, proteins, lactose, drug molecules, and other cellular constituents. The evagination of the plasma membrane is pinched off and released into the alveolar lumen. Within the extravascular space, the drug may be bound to proteins in the interstitial fluid. Some agents in free solution can pass into the alveolar milk directly by way of the spaces between the mammary alveolar cells. These paracellular areas account for a major portion of the fluid changes across the epithelium. These spaces between adjacent alveolar cells serve to carry water-soluble drugs from the tissue into the milk.
The intercellular junctions are “open” at delivery as lactation is being established and gradually “tighten” over the next few days. The amount of drug passed into milk on day 1 is greater than on day 3 or later. The composition of the milk changes from colostrum to mature milk, altering the amount of protein and fat, which could also influence drug levels in the milk. It is always important to know when plasma and milk samples were measured in relationship to the onset of lactation. Furthermore, some studies have been done on nonlactating women by pumping enough milk to measure the drug. These “weaning samples” provide only misinformation.
Ionization
Drugs that are nonionized are excreted in the milk in greater amounts than are ionized compounds. Depending on the pH of the solvent and the drug dissociation constant (p K a ), many weak electrolytes are more or less ionized in solution. Blood plasma and interstitial fluid are slightly alkaline (pH 7.4). Drugs that are weak acids are ionized to a greater extent in alkaline solution and are more extensively bound to protein. The amount of drug excreted from plasma (pH 7.4) to milk (pH 6.8 to 7.3, average 7.0) depends on the pH of the compound. Thus a weakly acidic compound has a higher concentration in plasma than in milk. Conversely, weakly alkaline compounds are in equal or higher levels in the milk than in the plasma.
The degree of drug ionization changes with the pH of the plasma and milk. Weak bases become more ionized with decreasing pH; thus the ionized component will increase in milk. The concentration in plasma and milk for the nonionized fraction will be the same, but the total amount of drug in the milk will be greater than in plasma. The sulfonamides demonstrate the effect of the p K a on the concentration of drug that reaches the milk. Sulfacetamide, with a low p K a (5.4), has a low milk/plasma (M/P) ratio (0.08), whereas sulfanilamide has a p K a of 10.4 and an M/P ratio of 1.00 ( Table 12-1 ).
Sulfonamide | Milk/Plasma Ratio | p K a |
---|---|---|
Sulfacetamide | 0.08 | 5.4 |
Sulfadiazine | 0.21 | 6.5 |
Sulfathiazole | 0.43 | 7.1 |
Sulfamethazine | 0.51 | 7.4 |
Sulfapyridine | 0.85 | 8.4 |
Sulfanilamide | 1.00 | 10.4 |
The studies done in cows and goats with constant infusions demonstrate this principle more dramatically because the pH of bovine plasma is 7.4 to 7.5 and the pH of bovine milk is 6.5. Under normal circumstances, however, concentrations of drugs are rarely constant, and there is a delay in achieving a new equilibrium. During periods of rapidly decreasing blood levels, some back diffusion occurs into the plasma.
Molecular Weight
The passage of molecules into the milk also depends on the size of the molecule, or the molecular weight (mol wt, in daltons). Water-filled membranous pores permit the movement of molecules of less than 100 mol wt. Because of action similar to the limitation of transport of certain large-molecular-weight chemicals across the placenta, insulin and heparin are not found in human milk either, presumably because of the molecule’s size.
Solubility
The alveolar epithelium of the breast is a lipid barrier that is most permeable in the first few days of lactation, when colostrum is being produced. The solubility of a compound in water and in lipid is a determining factor in its transfer. Nonionized drugs, which are lipid soluble, usually dissolve and descend in the lipid phase of the membrane. The solubility is closely linked to the manner in which the drug crosses the membranes ( Table 12-2 ). The membrane of the alveolar epithelial cells is composed of lipoprotein, glycolipid, phospholipid, and free lipids, as described in Chapter 4 . The transfer of water-soluble drugs and ions is inhibited by this hydrophobic barrier. Water-soluble materials pass through pores in the basement membrane and paracellular spaces. Low lipid solubility of a nonionized compound will diminish its excretion into milk.
General Drug Type | Milk/Plasma (M/P) Ratio |
---|---|
Highly lipid-soluble drugs | ~ 1 |
Highly protein-bound drugs in maternal serum | < 1 |
Small (mol wt < 200) water-soluble drugs | ~ 1 |
Weak acids | ≤ 1 |
Weak bases | ≥ 1 |
Actively transported drugs | > 1 |
Lipid solubility affects the profile of the drug in the milk and plasma. A drug with high lipid solubility will have parallel elimination curves in the plasma and the milk. A drug with low lipid solubility will clear the plasma at a constant rate, but the clearance curve for the milk will peak lower and later, and the drug will linger in the milk. A prolonged terminal elimination phase may exist when time between feedings is long.
Mechanisms of Transport
Drugs pass into milk by simple diffusion, carrier-mediated diffusion, or active transport, as follows:
Simple diffusion : Concentration gradient decreases
Carrier-mediated diffusion : Concentration gradient decreases
Active transport : Concentration gradient increases
Pinocytosis
Reverse Pinocytosis
Pharmacokinetic principles relate to the specific variation with time of the drug concentration in the blood or plasma as a result of its absorption, distribution, and elimination. Ultimately, by extrapolation of these factors, one determines the effect of the drug. The most elementary kinetic model is based on the body as a single compartment. Distribution of the drug in the compartment is assumed to be uniform and rapidly equilibrated. In the single-compartment model, the volume of distribution of a drug is considered to be the same as that of the plasma, assuming a rapid uniform distribution. The volume of distribution (V d ) is calculated as follows:
V d = Total amount drug in body Concentration of drug in plasma
The absorption and elimination are considered to be exponential or first-order kinetics. A two-compartment model of drug kinetics takes into account the phase of decreasing drug concentration as the drug distributes into the tissues. Initially, concentrations fall rapidly as the drug distributes, then first-order elimination follows. When considering the pharmacokinetics of drugs in breast milk, one must also consider that elimination in the breast is by two potential routes: excreted with the milk to the infant and back diffusion into the plasma to reequilibrate with the falling level in the plasma.
With access to the volume of distribution of the drug in question, the amount of the dose, and the weight of the mother, the concentration of drug in breast milk could be theoretically calculated as follows:
Concentration in breast milk = Dose Volume of distribution
Other models have been developed for measuring the amount of drug that reaches the infant when the M/P ratio is not known. Using a stepwise linear regression for acidic and basic drugs, based on the drug’s p K a , the plasma protein binding value, and the octanol/water partition coefficient, an M/P ratio can be calculated. In a study of several proposed equations, the error is lowest for the drugs with the highest M/P ratio, that protein binding is the most important single predictor, and that the M/P ratios for basic drugs are more accurately predictable.
The concentration of the drug in the circulation of the mother depends on the mode of administration: oral, IV, IM, or TDDS. Absorption through the skin, the lungs (inhalants), or vaginally may also need to be considered.
The levels in the blood depend on the route of administration. The curves produced by bolus IV medication peak high and early and taper sharply, thus making avoiding peak plasma levels more feasible. Absorption from IM dosing is less rapid but follows a similar but less sharp curve. Oral dosing depends on other factors, such as whether the medication is taken between or during meals. Depending on the curve of uptake and removal of drug from the plasma, the area under the curve varies. Single doses are simple area-under-the-curve calculations, but calculations for multiple doses or chronic use vary with the steady state of the drug in the body. TDDS patches deliver the medication at a constant rate continuously.
Nonelectrolytes such as ethanol, urea, and antipyrine enter the milk by diffusion through the lipid membrane barrier and may reach the same concentrations in the milk as in the plasma, regardless of the pH. The main entrance site of molecules is at the basement laminal membrane, where water-soluble materials pass through the alveolar pores. Nonionized drugs cross the membrane more easily than ionized ones because of the structure of the membrane. The nonionized drugs pass through the membrane by diffusion. When simple diffusion takes place, the M/P ratio is 1.0. Passive diffusion provides the same ratio regardless of the plasma concentrations of the drug or the volume of milk secreted. Different M/P ratios depend on the binding to protein and are a measure of the protein-free fraction. The dissimilar ratios for the sulfa drugs (see Table 12-1 ) partly result from the difference in protein binding and partly from ionization.
Large molecules depend on their lipid solubility and ionization to cross the membrane, because they pass in a lipid-soluble nonionized form. The M/P ratio is determined when equilibrium exists in the amount of nonionized drug in the aqueous phase on both sides of the membrane. When drugs are only partially ionized, the nonionized fraction determines the concentration that crosses the membrane. The drugs for which the nonionized fraction is not very lipid soluble will pass only in limited degree into breast milk.
Passive drug transport may occur in the form of facilitated diffusion . The active compound is transported across the cell membrane by a carrier enzyme or protein. The gradient is toward a lesser or equal concentration in both simple diffusion and facilitated diffusion and is controlled by chemical activity gradients. Facilitated diffusion usually involves a water-soluble substance too large to pass through the membrane pores.
Active transport mechanisms provide a process whereby the gradient is “uphill,” or higher, in the milk. The process is similar to facilitated diffusion except that metabolic energy is required to overcome the gradient. Examples of substances actively transported include glucose, amino acids, calcium, magnesium, and sodium. Pinocytosis and reverse pinocytosis, as described previously, are involved in the transport of very large molecules and proteins. Chloride ions are secreted into milk via an active apical membrane pump, whereas sodium and potassium are diffused by electrical gradient. Because the level of sodium is kept low, an active return of sodium may occur into the plasma, referred to as a reverse pump . The TDDS depends on absorption of the drug through the skin at a steady rate; it has become a significant route of administration for certain medications. The delivery rate is determined by diffusion of drug from the reservoir matrix through the epidermis. This method offers some advantages, including convenience of dosing, reduced dosing frequency, ease of reaching a steady state, increased patient compliance, avoidance of first-pass hepatic biotransformation, avoidance of peaks and valleys in blood levels, and reduction of side effects through heightened selectivity of drug action. The level in the plasma remains constant during the drug’s anticipated life span while the patch is in place. The technology is limited to drugs with low molecular weight that are hydrophilic and can diffuse through the stratum corneum. The top molecular weight is 500 daltons. For patient compliance and economics the patch size is limited to 50 cm in diameter. Occasional patients experience skin irritation. Currently patches are limited to drugs that are potent in small amounts and highly diffusible through the skin. To maintain a constant rate, a surplus of drug must be present, often 20 to 30 times the amount that will be absorbed during the time of application. The potential for toxicity is great. If a patch is utilized while lactating it should be applied and covered so a nursing infant cannot accidently get to it.
TDDS patches are available for scopolamine, nicotine, clonidine, fentanyl, and other drugs ( Table 12-3 ).
Generic Drug | Brand Name | Strengths/Release Rate | Application Frequency | Total Drug Content per Patch |
---|---|---|---|---|
Clonidine | Catapres-TTS | 0.1, 0.2, 0.3 mg/24 h | 7 days | 2.5, 5, 7.5 mg |
Estradiol | Alora | 0.025, 0.05, 0.075, 0.1 mg/24 h | 7 days | 0.77, 1.5, 2.3, 3.1 mg |
Climara | 0.025, 0.0375, 0.05, 0.06, 0.75, 0.1 mg/24 h | 7 days | 2, 2.85, 3.8, 4.55, 5.7, 7.6 mg | |
Estraderm | 0.05, 0.1 mg/24 h | 3-4 days | 4 mg, 8 mg | |
Vivelle-Dot | 0.025, 0.0375, 0.05, 0.075, 0.1 mg/24 h | 3-4 days | 0.62/2.7 mg, 0.51/4.8 mg | |
Estradiol/Norelgestromin | Ortho Evra | 20 mcg/150 mcg/24 h | 7 days | 0.75 mg/6 mg |
Fentanyl | Duragesic | 12.5, 25, 50, 100 mcg/h | 72 hours | 1.25, 2.5, 5, 7.5, 10 mg |
Lidocaine | Lidoderm | 35 mg/12 h | 12 h/day | 700 mg |
Methylphenidate | Daytrana | 10, 15, 20, 30 mg/9 h | 9 h/day | 27.5, 41, 3, 55, 82.5 mg |
Nicotine | Habitrol | 7, 14, 21 mg/24 h | 16-24 h/day | 17.5, 35, 52.5 mg |
NicoDerm CQ | 7, 14, 21 mg/24 h | 16-24 h/day | 36, 78, 114 mg | |
Nitroglycerin | Nitro-Dur | 0.1, 0.2, 0.3, 0.4, 0.6, 0.8 mg/h | 12-14 h/day | 20, 40, 60, 80, 120, 160 mg |
Minitran | 0.1, 0.2, 0.4, 0.6 mg/h | 12-14 h/day | Approx 8.6, 17, 34, 51.4 mg | |
Oxybutynin | Oxytrol | 3.9 mg/24 h | 24 h | 36 mg |
Rotigotine | Neupro | 2, 4, 6 mg/24 h | 24 h | 4.5, 9, 13.5 mg |
Scopolamine | Transderm-Scop | 1.0 mg/72 h | 3 days | 1.5 mg |
Selegiline | Emsam | 6, 9, 12 mg/24 h | 24 h | 20, 30, 40 mg |
Testosterone | Androderm | 2.5, 5 mg/24 h | 24 h | 12.2, 24, 3 mg |
A summary of the steps in the passage of drugs into breast milk follows:
- 1.
Mammary alveolar epithelium represents a lipid barrier with water-filled pores and is most permeable for drugs during the colostral phase of milk secretion (first week postpartum).
- 2.
Drug excretion into milk depends on the drug’s degree of ionization, molecular weight, solubility in fat and water, and relation of pH of plasma (7.4) to pH of milk (7.0).
- 3.
Drugs preferably enter mammary cells basally in the nonionized, nonprotein-bound form by diffusion or active transport.
- 4.
Water-soluble drugs less than 200 mol wt pass through water-filled membranous pores.
- 5.
Drugs leave mammary alveolar cells apically by diffusion or active transport.
- 6.
Drugs may enter milk via spaces between mammary alveolar cells.
- 7.
Most ingested drugs appear in milk; drug amounts in milk usually do not exceed 1% of ingested dosage, and levels in the milk are independent of milk volume.
- 8.
Drugs are bound much less to milk proteins than to plasma proteins.
- 9.
Drug-metabolizing capacity of mammary epithelium is not understood.
Effect on Nursing Infant
Absorption From Gastrointestinal Tract
Although concern surrounds the amount of a given agent in the breast milk, of greater importance is the amount absorbed into an infant’s bloodstream. No accurate way exists to measure this because other factors also affect the level in an infant’s bloodstream. The tolerance of the chemical to the pH of the stomach and the enzymatic activity of the intestinal tract are significant. The volume of milk consumed is a factor as well. Some drugs are not well absorbed with food (see later discussion of food-drug interactions). Oral bioavailability of a compound is a major factor of risk for an infant.
Infant’s Ability to Detoxify and Excrete Agent
Any drug given to an infant by any route has to be evaluated according to the infant’s ability to detoxify or conjugate the chemical in the liver and excrete it in the urine or stool. Some compounds that appear in milk in very low levels are not well excreted by infants and therefore accumulate in infants’ systems to the point of toxicity.
Drugs that depend on the liver for conjugation, such as acetaminophen, are theoretic risks because of the limited reserve of the neonatal hepatic detoxification system. When actual measurements were made of neonates given acetaminophen, they were noted to handle it well because they conjugate it in the sulfhydryl system as an alternative pathway, which is used only to a small extent in adult metabolism of acetaminophen. When a single dose of a drug is given to a mother and the level is measured in her milk and in her infant, it does not give a clear picture of the potential for accumulation in the infant’s system. The competition for binding a drug to protein is also important. Some drugs, such as sulfadiazine, compete for binding sites that might normally bind bilirubin in the first week or so of life. This puts an infant in jeopardy of kernicterus at a given bilirubin level because of an increase in the fraction of bilirubin left unbound for lack of binding sites. The indirect bilirubin level may even appear to be less than the dangerous level. Some other compounds that displace bilirubin from albumin-binding sites include salicylic acid (aspirin or acetylsalicylic acid breaks down to salicylic acid), furosemide, and phenylbutazone.
The maturity of an infant at birth is an extremely important factor during the first few months of life; thus the gestational age at birth should be established. Clearly, the less mature the infant, the less well tolerated drugs are, not only because of the immaturity of the organ systems but also because of differences in body composition ( Figure 12-2 ). The less mature an infant, the greater the water content of the body and the proportion of extracellular water. Although the percentage of body weight that is protein is similar for all newborns (i.e., 12%), the absolute amount of protein for binding is less the smaller an infant is. The amount of body fat is also low, by percentage of body weight and in absolute values. The distribution of highly lipid-soluble drugs therefore will be more apt to deposit in the brain of a 1000-g infant with 3% body fat by weight than in a 3500-g full-term infant with 12% body fat. This may explain the more sedating effect of a drug on the central nervous system (CNS) of a smaller, younger, and less mature infant. The relative lack of plasma protein-binding sites in a small, premature infant compared with a more mature, older infant results in more free (unbound) active drug in circulation. Complications of premature birth, such as acidosis and hypoxia, also contribute to the unavailability of albumin-binding sites and thus result in more unbound drug.

The inability of the liver to metabolize drugs effectively results in the accumulation of some compounds that might be readily cleared by an older infant. At about 42 weeks’ conceptual age, an infant’s liver is able to metabolize most drugs competently. Renal clearance similarly is less effective with decreasing maturity, which increases the risk for drug accumulation. The need to dose a premature infant only once or twice per day is common to many drugs, such as antibiotics, caffeine, and theophylline, and confirms that a small, premature infant does not clear drugs well.
Special problems in neonates in addition to the presence of jaundice or low serum albumin may require special consideration. Low Apgar scores at birth signifying some degree of stress, hypoxia, or acidosis may alter binding-site availability but may also alter metabolism and excretion of a drug. Continuing respiratory distress requiring ventilatory support, sepsis, and renal failure demand special consideration when determining if a sick neonate can receive the mother’s milk when she is being treated with certain medications. Prescribing for such a mother should be done in consultation with the neonatologist if the woman is providing her milk for her infant.
The age of an infant makes a difference in the total volume of milk consumed; in an older child, the diet includes other items so that milk does not compose the total intake. Age makes a difference because the more mature infant can metabolize drugs more effectively; thus sulfa drugs, for instance, can be given to infants after the first month of life.
If the agent is fat soluble, the fat content of the milk may be a significant variable. The fat content at any feeding increases over time; thus the so-called foremilk is low in fat and the hindmilk is four to five times richer in fat toward the end of a feeding. Even though the total amount of fat will be about the same in each 24-hour period, the total amount of fat in a given feeding is less in the morning, peaks at midday, and drops off in the evening. The coefficient of lipid solubility for a nonionized drug determines both its penetration of the biologic membrane to gain entrance to milk and its concentration in milk fat. Sulfonamides with low fat solubility are in the aqueous and protein fraction of milk, whereas many barbiturates are in the lipid fraction. An inverse relationship exists between a drug’s lipid solubility and the amount that appears in the skim fraction. The concentrations in fat differ for each member of the barbital family. Pentobarbital and secobarbital are found in the lipid phase, whereas phenobarbital is found in the aqueous phase.
The agent may appear in low levels in a mother’s serum, but mammary blood flow during lactation is 500 mL/min and a mother produces between 60 and 300 mL of milk per hour. The agent that appears in minimal concentrations in the milk may present a significant problem when one considers that 1000 mL of milk may be consumed in a day by an infant. Even though the volume is low, during the colostral phase of lactation, the breast is more permeable to drugs.
Breast Milk/Plasma Ratio for Drugs Usually Not Useful
The M/P ratio for drugs has been measured and reported for many medications. By definition, M/P ratio is the concentration of the drug in the milk versus the concentration in maternal plasma (serum) at the same time. It presumes that the relationship between the two concentrations remains constant, which in most cases it does not. If it were a constant, it would allow the estimation of the amount of drug in the milk from any given plasma level in a mother.
An inaccurate ratio, or one determined under variable circumstances, produces erroneous estimates of the amount of drug in the milk, A pharmacokinetic model is a requisite foundation for studies of drugs in breast milk. A single-point-in-time M/P ratio, or an average ratio calculated with single-dose, area-under-the-curve data, does not work for all drugs. Neither ratio accounts for the importance of time-dependent variations of drug concentration in milk.
The M/P ratio is most valuable if obtained when an infant would be nursed. If the ratio is 1:0, it means only that the levels are equal. If the level is minimal in a mother’s plasma because of the large volume of distribution, and if the milk level is also low, the M/P ratio is 1:0. If levels are drawn at peak plasma level and are equal, the M/P is still 1:0, but the infant receives a large dose. Thus the M/P ratio is valuable only when the time of the measurement is known in relationship to dosing of the mother. Dose strength, duration of dosing, maternal variation in drug disposition, maternal disease, drug interactions and competition of additional drugs for metabolism or binding sites, and racial variations in drug metabolism all influence the M/P interpretation. The M/P ratio may be greater than 1, which sounds alarming; however, a drug with a large volume of distribution will have low levels in the plasma and possibly high milk levels, but neither level may be therapeutically significant. The M/P ratio only confirms that the drug gets in the milk, and the safety cannot be measured.
Evaluating Drug Data
The paucity of carefully controlled studies on large enough samples to validate the results when such a large number of variables are active has been lamented by many authors. Some data collected are not pharmacokinetically sound. A clinician needs to understand these variables as well as pharmacokinetic principles to make a reasonable judgment about a given case.
Interethnic and racial differences in drug responsiveness are well established. The increased heterogeneity of national populations has brought increased awareness of genetic diversity. Plasma binding, especially with drugs dependent on glycoproteins for binding, often varies greatly between Caucasian and Chinese subjects, for example. Such factors contribute to the differences in drug disposition and pharmacologic response.
It should be theoretically possible to determine how much of a specific drug reaches an infant in the mother’s milk by knowing all the properties of the drug, including its volume of distribution, ionization, p K a , lipid solubility, protein-binding activity, and rate of detoxification in the maternal system. Sufficient variation in the levels that reach an infant and in how the infant deals with the agent, however, makes it necessary to have specific data about a specific drug. Thus a few simple questions in the decision-making process are helpful in determining risk.
Safety for Infant
Ask, “Is this a drug that can be given to the infant directly if necessary?” Antibiotics (e.g., penicillin) that one could give an infant are in this category, whereas an antibiotic such as chloramphenicol, which one would not give an infant under ordinary circumstances, should be avoided in a nursing mother. The toxicity of chloramphenicol in an infant is dose related and associated with an unpredictable accumulation of the drug. Also, an idiosyncratic reaction occurs with chloramphenicol, which is unrelated to dose but is capable of causing pancytopenia.
If the drug in question can be given to an infant, does the amount in the milk create any risk to the infant? Phenobarbital can be given to infants for various reasons; thus the question is whether enough will reach the infant to cause difficulty. The infant should be watched for symptoms of lethargy or sleepiness, such as a change in feeding or sleeping pattern. If the infant is sleeping long periods and feeding less than usual (specifically, fewer than five or six times per day), the medication may be at fault. Phenobarbital is a significant drug for a mother with seizures; therefore a careful review of the risk/benefit ratio to both mother and infant should be undertaken. Barbiturates vary in their effect in young infants. A newborn does not handle the short-acting barbiturates well because they are dependent on detoxification in the liver, whereas phenobarbital depends more on the kidney for excretion.
If the drug was taken during pregnancy, as for epilepsy, an infant will already have the drug in his or her system via the placenta at a steady state and will have to begin to excrete it on his or her own after delivery. Enzyme induction may have taken place in the neonate, however, because of exposure to the drug in utero; phenobarbital hastens maturation of the fetal liver. Enzyme induction of the hepatic oxygenase system by phenobarbital, phenytoin, primidone, and carbamazepine is well established. Valproate, however, does not induce enzyme activity.
If one can safely give a drug to an infant, administration becomes a question of watching for any symptoms of excessive accumulation. The age of the infant affects the ability to clear the drug.
When the drug in question is one not normally given to an infant at that particular age, weight, or degree of maturity, decision-making is more difficult. Specific information about the amount of the drug that appears in the milk is essential in decision-making. Often, conflicting information is available. Many lists of drug-milk levels have perpetuated the same errors in calculation; thus having more than one reference report the same information may not provide confirmation of its accuracy.
If a medication will have to be taken for weeks or months, as with cardiovascular drugs, the drug has greater potential impact than when taken only for a few days. If the drug exposure has already occurred for 9 months in utero, some think it is less of a problem; however, it may compound the problem.
To determine the dose delivered to an infant, the following formula is used:
Dose / 24 hours = Concentration of drug in milk × Weight kg of infant × Volume of milk per kg ingested in 24 hours Dose / 24 hours = C milk × Weight × Volume / kg / 24 hours
It has been recommended by Ito and by Hale that the calculation be the relative infant dose (RID), which is calculated as follows:
RID = Absolute infant dose mg / kg / day Maternal dose mg / kg / day × 100
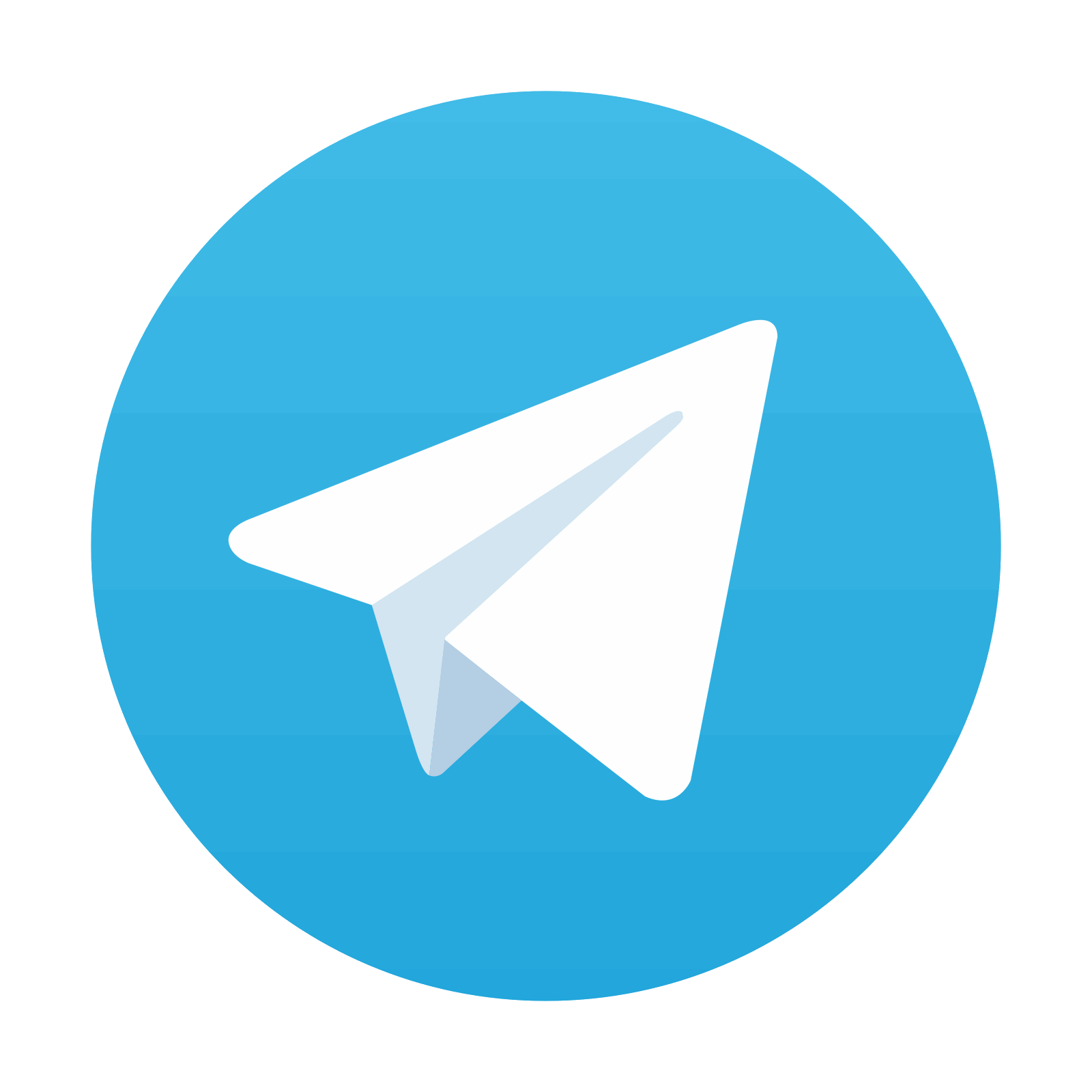
Stay updated, free articles. Join our Telegram channel

Full access? Get Clinical Tree
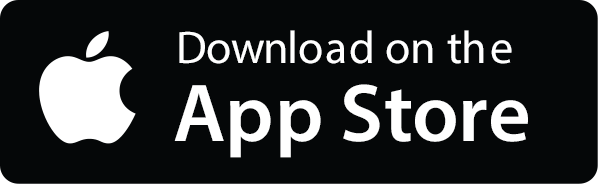
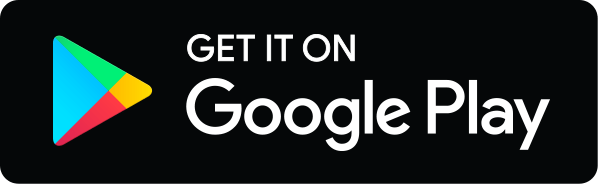