Objective
Because the fetal innate immune system is responsive, while still maturing during the preterm period, we hypothesized that the early activation of fetal inflammatory pathways may have an impact on the ultimate expression of immune competency.
Study Design
Pregnant Sprague Dawley rats (n = 7; Harlan Sprague Dawley Inc, Jerusalem, Israel) at 18 days gestation received intraperitoneal injections of saline solution or lipopolysaccharides (500 μg/kg). Pups were delivered spontaneously. At postnatal day 24, pups received intraperitoneal lipopolysaccharide (100 μg/kg), and plasma cytokine levels were measured before and 4 hours after lipopolysaccharide administration.
Results
In response to lipopolysaccharides, pups of the lipopolysaccharide-injected dams had significantly ( P < .05) reduced interleukin-6 (median [25th,75th percentile], 229 [84,6086] vs 4745 [2765,6643] pg/mL), interleukin-1β (median [25th,75th percentile], 820 [125,1196] vs 1682 [1515,2127] pg/mL), tumor necrosis factor–α (median [25th,75th percentile], 4.8 [1.2,91] vs 163 [46,205] pg/mL), and interleukin-10 responses, when compared with saline solution–injected dams.
Conclusion
Maternal lipopolysaccharide exposure suppresses offspring innate immune response to inflammatory stimuli. These results suggest that maternal inflammatory exposures during pregnancy may impair newborn infant innate responses and increase susceptibility to infection.
Epidemiologic data and animal studies both support the hypothesis that conditions that prevail in the womb during prenatal development may program adult metabolic processes and contribute to adult disease. Long latency periods between the environmental trigger and the onset of subsequent disease is recognized in the pathogenesis of certain cancers, and many lines of evidence indicate that early life events play a powerful role in influencing later susceptibility to certain chronic diseases.
In humans, low birthweight and relative thinness at birth is associated with increased rates of coronary heart disease, stroke, type 2 diabetes mellitus, adiposity, and osteoporosis in adult life. Animal models have confirmed the association of newborn infant growth restriction with the development of adult metabolic syndrome and have begun to determine the underlying mechanisms of developmental programming. Although the effects of fetal growth restriction have been examined extensively, additional gestational stressors may have an impact on the developing fetus. For example, fetal glucocorticoid exposure or in utero hypoxemia may program offspring physiologic condition and disease expression. Maternal inflammation/infection occurs commonly during the 9-month human gestation period, with viral disorders and systemic bacterial infections (eg, pneumonia or pyelonephritis) that manifest prenatally and chorioamniotic infections that manifest before or during labor. Our previous work in rats demonstrated that maternally administered lipopolysaccharides can induce fetal inflammatory responses, which is evident by dramatic increases in fetal blood proinflammatory cytokines and increases in the expression of these cytokines in the fetal brain.
The innate immune response develops during the fetal life and neonatal period, although maturation is not achieved until months after birth. The reduced fetal immune reactivity may reflect the demands of in utero existence, which include the need to avoid potentially harmful inflammatory immune reactions. Despite normal basal expression of Toll-like receptors (TLR) and membrane cluster of differentiation 14, innate immune responses of neonatal mononuclear cells to lipopolysaccharide are characterized by markedly reduced release of the proinflammatory T-helper 1–polarizing cytokines, tumor necrosis factor–alpha (TNF-α), and interferon-γ, with relative preservation of antiinflammatory T-helper 2–polarizing cytokines. Lipopolysaccharides and other inflammatory mediators may cross the placenta and initiate a cascade of events that result in fetal oxidative stress, which is thought to play an important role in the capability of the fetus to cope with the current environment.
In neonates, the innate immune response is the main defense mechanism against infections. Attachment of lipopolysaccharide to the TLR4 on the surface of the monocyte facilitates a cascade of events that initiate and mediate the inflammatory response. We sought to determine whether maternal lipopolysaccharide exposure potentially modulates and programs neonatal offspring immune responses to subsequent lipopolysaccharide stimulation.
Materials and Methods
Animals and treatments
The protocols and procedures for this study were approved by the Institutional Animal Care and Utilization Committee at Rappaport Research and Education Institute. Sprague-Dawley pregnant rats (n=14; Harlan Sprague Dawley, Inc, Jerusalem, Israel) were obtained at gestational day 16 (term, 21 days) and allowed to acclimate for 48 hours before experiments were begun. Animals were maintained in temperature- (37°C) and light- (6 am , lights on; 6 pm , lights off) controlled facilities with access to food (LabDiet 5001 Rodent Diet; PMI Nutrition International, LLC, Jerusalem, Israel) and water ad libitum throughout the study. At gestational day 18, the pregnant rats were assigned randomly to receive intraperitoneal injections of physiologic saline solution or lipopolysaccharides (7 dams in each group). Lipopolysaccharides ( Escherichia coli , serotype 0111:B4; Calbiochem, San Diego, CA) were reconstituted in physiologic saline solution and administered at 500 μg/kg bodyweight. The pups were delivered spontaneously (term, 21 days) and allowed to mature until postnatal day 24.
At day 24, 2-3 offspring of each dam (14-21 pups in each group) were injected with intraperitoneal lipopolysaccharides (100 μg/kg body weight) and 2-3 offspring of each dam were killed with no injection to compare the basal cytokine levels (the remaining pups of each group would be challenged with a similar protocol at an older age). Four hours after lipopolysaccharide injection, the neonate pups were anesthetized with intramuscular ketamine and xylazine. Pup heart cavities were exposed by midline incision; blood was collected by cardiac puncture and centrifuged at 4°C to isolate plasma. All samples subsequently were stored at –20°C for further processing and analyses.
Enzyme-linked immunosorbent assay determinations
Commercial enzyme-linked immunosorbent assay (R&D Systems, Minneapolis, MN) kits were used to determine neonatal plasma protein levels of the cytokines interleukin (IL)-6, -1β, and -10, as previously described. For all assays, the minimum detectable concentration was <10 pg/mL with intra- and interassay variations of <10%.
Data analyses
Blood levels of inflammatory biomarkers were compared between offspring from each group. Because neonatal responses were not distributed normally, values were analyzed by Mann-Whitney nonparametric tests. All results are expressed as median and interquartile range and means ± SEM. Difference were considered to be significant only for a probability value of < .05.
Results
Seven dams in the saline solution–treated group delivered 88 pups; 7 dams in the lipopolysaccharide-treated group delivered 78 pups (no significant difference). The 2 groups gave birth at the same gestational age (term, 21 days), and no preterm births were noted in the lipopolysaccharide group. The birthweight was significantly higher in the saline solution group, compared with the lipopolysaccharide group (6.4 ± 0.4 vs 5.8 ± 0.2 g; P < .05).
Basal inflammatory cytokine levels were undetectable in both groups. At 4 hours after lipopolysaccharide challenge (as a previous study demonstrated, peak levels of IL-6, -1β, and -10 are obtained 4-6 hours after lipopolysaccharides in rat neonates), the pups of the lipopolysaccharide-treated dams had significantly lower levels of proinflammatory cytokines IL-6 (2601 ± 727 vs 4774 ± 510 pg/mL; median [25th,75th percentile], 229 [84,6086] vs 4745 [2765,6643] pg/mL; P < .05), -1β (456 ± 198 vs 1677 ± 102 pg/mL; median [25th,75th percentile], 820 [125,1196] vs 1682 [1515,2127] pg/mL; P < .05), and TNF-α (59 ± 21 vs 160 ± 22 pg/mL; median [25th,75th percentile], 4.8 [1.2,91] vs 163 [46,205] pg/mL; P < .05), when compared with the pups of the saline solution–injected dams ( Figure 1 ). Lipopolysaccharides induced a small increase in the antiinflammatory cytokine IL-10 in both groups, although the response was reduced in the pups of lipopolysaccharide-treated dams compared with pups of saline solution–injected dams (65 ± 17 vs 127 ± 17 pg/mL; median [25th,75th percentile], 56 [0,100] vs 121 [46,175] pg/mL; P < .05; Figure 2 ).

Full access? Get Clinical Tree
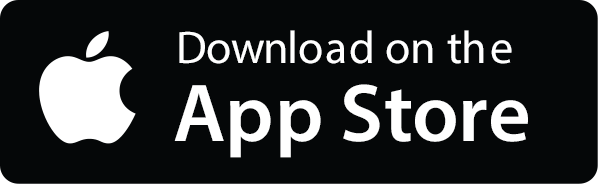
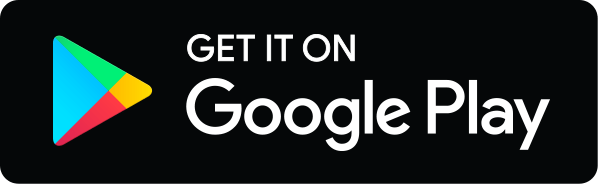