Objective
This work aimed to verify the hypothesis that maternal intake of high-fat diet in critical periods of pregnancy and/or suckling period predisposes nonalcoholic fatty liver disease in adult C57BL/6 mice offspring.
Study Design
Male pups were divided into 5 groups: (1) SC, from standard chow–fed dams; (2) G, from high-fat chow (HF)–fed dams during the gestation (G) period; (3) L, from HF-fed dams during the lactation (L) period; (4) GL, from HF-fed dams during the gestation and lactation (GL) periods; and (5) GL/HF, from HF-fed dams during GL, maintaining an HF diet from postweaning to adulthood. We analyzed body mass, plasma blood, and liver structure.
Results
The G offspring showed insulin resistance and lower glucose transporter-2 expression. Hepatic steatosis was present in the G, L, GL, and mainly in GL/HF offspring. Sterol regulatory element-binding protein-1c expression was higher in G, GL, and GL/HF offspring.
Conclusion
Programming by HF chow predisposes hepatic adverse remodeling in the liver of adult offspring.
The term nonalcoholic fatty liver disease (NAFLD) is used to describe a spectrum of structural findings ranging from simple steatosis to nonalcoholic steatohepatitis (NASH) with progressive fibrosis and liver failure. The condition sine qua non of NAFLD patients includes macro- and microvesicular steatosis and in NASH patients include macrovesicular or a mix between micro- and macrovesicular steatosis with mild lobular inflammation. As fibrosis develops, changes occur within the subendothelial space and within the hepatic sinusoid.
These changes include alterations in both cellular responses and extracellular matrix composition. Activation of the hepatic stellate cells leads to accumulation of a scar (fibril-forming) matrix, which results in widening of the space of Disse and loss of endothelial fenestrae. Transport across the sinusoidal wall is hence reduced, leading to deterioration of hepatic function.
In NAFLD, triglycerides are ultimately synthesized from fatty acids (FAs). There are numerous potential sources of FAs used to generate triglycerides, but dietary FAs are a main source. The FAs derived from lipolysis of adipose tissue triglyceride depots are also delivered to the liver, taken up by hepatocytes, and converted into triglycerides.
De novo lipogenesis is another factor that leads to steatosis in NAFLD, a process regulated by transcription factors that are activated by insulin, particularly sterol regulatory element binding protein (SREBP)-1c. The high insulin levels increase the expression of SREBP-1c, which increases the expression of all lipogenic enzymes, thus increasing hepatic free fatty acid (FFA) synthesis, which also increases the hepatic expression of all hepatic lipogenic genes, thus further increasing hepatic FFA synthesis.
FAs may also accumulate within hepatocytes because their metabolism is impaired. In healthy hepatocytes, FAs are oxidized by enzymes in peroxisomes, mitochondria, and the endoplasmic reticulum (microsomes). Regardless of the source of FAs that hepatocytes use to produce triglycerides, this triglyceride is normally packaged into lipoproteins in the hepatocyte endoplasmatic reticulum and then exported to adipose depots for storage. Therefore, accumulation of fat in the liver leads to an excessive delivery of FFAs from visceral adipose tissue into the liver and from a misbalance in de novo synthesis and catabolism in hepatocytes.
Some models, such as nonhuman primate and murine, are used to correlate the intrauterine environment and development of NAFLD. Different mechanisms are involved in the offspring insult because of adverse maternal nutrition. Up-regulation of specific placental nutrient transporter isoforms constitutes a mechanism linking maternal high-fat (HF) diet and obesity to fetal overgrowth and modulates the offspring glucose homeostasis. Therefore, this study aimed to investigate how the maternal intake of HF diet in different critical periods of pregnancy and/or suckling period influences carbohydrates metabolism alterations and NAFLD in adulthood offspring.
Materials and Methods
Animals and diet
Experimental protocols were approved by the local committee according to conventional guidelines for experimentation with animals. Animals were maintained under controlled conditions of temperature and humidity, and 12 hour dark, 12 hour light cycle, with free access to water and food. C57BL/6 virgin mature females were caged with males overnight, and mating was confirmed the next morning (vaginal plug). Then females were allocated to be fed either a standard chow (SC; 17% fat, 19% protein, and 64% carbohydrate, equivalent to 16.5 kJ/g) or a HF chow (49% fat, 19% protein, and 32% carbohydrate, equivalent to 20.7 kJ/g, 25.5% more energy than the SC diet). Both diets, including the micronutrient mineral mix, followed the American Institute of Nutrition recommendation (AIN-93G).
HF feeding was targeted to specific periods in gestation (G) and/or (L) lactation ( Figure 1 ): G (HF diet was taken during the gestation); L (HF diet was taken during lactation); GL (HF diet was taken in both gestation and lactation), and GL/HF (HF diet was taken in both gestation and lactation and continued from postweaning until 3 months old). Immediately after delivery, litters were adjusted to 6 animals per mother (to assure adequate and standardized nutrition until weaning), and at weaning, 1 male pup per litter was randomly assigned to form the groups of study (7 animals/group).

Offspring body mass evolution was monitored until the euthanasia. Food intake was estimated by subtracting the amount of food left on the grid and the amount of spilled food from the initial weight of food supplied. Energy intakes were calculated based on energetic value of diets. Feed efficiency was calculated as grams of the body mass gain per kilojoules of food consumed per animal.
Oral glucose tolerance test (OGTT) and plasma insulin
OGTT was made at 3 months old with 25% glucose in sterile saline (0.9% NaCl) (1 g/kg body mass [BM]) administered by orogastric gavage after 6 hours of a fasting period. Blood was collected from the tail vein at 0, 15, 30, 60, and 120 minutes after the glucose load, and blood glucose was measured using a glucometer (Accu-Chek; Roche, Sao Paulo, Brazil. The analysis considered the area under the curve to assess glucose intolerance (Prism version 5.03 for Windows; GraphPad Software, San Diego, CA).
Plasma insulin concentrations were measured by radioimmunoassay using a mouse insulin radioimmunoassay kit (catalog #RI-13K; Linco Research, St Charles, MO). All samples were analyzed in a double assay, for which the intraassay coefficient of variation was 1.4%. Insulin resistance was estimated by homeostasis model assessment for insulin resistance index (HOMA-IR) as [(fasting glucose × fasting insulin)/22.5].
Euthanasia
Offspring (3 months old) were deeply anesthetized and blood was collected by cardiac puncture. Then the liver was rapidly excised, weighed, and prepared for both light and electron microscopy. Genital fat deposit (adipose tissue surrounding the ureters, bladder, and epididymis) was dissected and weighed.
Liver enzymes and TNF-alpha
Total cholesterol (TC), triglyceride (TG), and hepatic enzymes concentrations were measured by a colorimetric assay (Bioclin; Belo Horizonte, Minas Gerais, Brazil): alanine aminotransferase (ALT), aspartate aminotransferase (AST), and alkaline phosphatase (ASP). Mice serum analysis for tumor necrosis factor (TNF)-alpha was performed using a commercially available enzyme-linked immunosorbent assay (ELISA) kit (human/mouse TNF-alpha ELISA Ready-SET-go; Bioscience, San Diego, CA).
Western blotting
Total hepatic proteins were extracted in homogenizing buffer and protease inhibitors. Thereafter the homogenates were centrifuged for 20 minutes at 4°C, and the supernatants were collected. Equal quantities of total protein were resuspended in sodium dodecyl sulfate (SDS)–containing sample buffer, heated for 5 minutes at 100°C, and separated by SDS-polyacrylamide gel electrophoresis. After electrophoresis, proteins were electroblotted on a polyvinyl difluoride transfer membrane (Amersham Biosciences, Piscataway, NJ).
The efficiency of the transfer was visualized by Ponceau solution staining. The membrane was blocked by incubation with nonfat dry milk (6% in Tween 20–Tris-buffered saline), incubated with polyclonal antibody against anti-rabbit SREBP-1c (68 kDa, SC-367; Santa Cruz Biotechnology, Santa Cruz, CA) and glucose transporter (GLUT)-2 (53-61 kDa, AB1342; Chemicon, Temecula, CA), washed, and incubated with antirabbit secondary antibody. SREBP-1c and GLUT-2 protein expressions were detected using an enhanced chemiluminescence detection system (Amersham).
Signals were visualized by autoradiography and determined by quantitative analysis of digital images of gels using ImagePro plus software, version 7.0 (Media Cybernetics, Silver Spring, MD). The integral optical density values were measured.
Liver stereology and ultrastructure
Liver fragments were immediately obtained and fixed in freshly prepared fixatives either for light microscopy (1.27 mol/L formaldehyde in 0.1 M phosphate buffer, pH 7.2) for 48 hours at room temperature or for transmission electron microscopy (0.1 M cacodylate buffer 2.5% glutaraldehyde, pH 7.2) for 2 hours, followed by postfixation in 1% osmium tetroxide for 45 minutes. For light microscopy material was embedded in Paraplast plus, sectioned at 3 μm of thickness, and stained by hematoxylin-eosin. For electron microscopy material was embedded in Epon, ultrathin sections were obtained using a Leica Ultracut ultramicrotome, counterstained with uranyl acetate and lead citrate, observed in a Zeiss EM 906 microscope (at 80 KV; Zeiss, Oberköchen, Germany).
The liver steatosis was assessed with stereology using a test system made up of 36 test points (P T ) superimposed to the microscopic fields as described elsewhere. Briefly, 6 microscopic fields per animal were analyzed at random with video-microscopy (Leica DMRBE microscope; Leica, Wetzlar, Germany; video camera; Kappa Gleichen, Germany; and Sony Trinitron monitor; Sony, Pencoed, UK). The steatosis volume density (Vv) was estimated by point counting: Vv [steatosis, liver] = P P [steatosis, liver]/P T , where P P is the number of points that hit the structure.
Data analysis
Data were tested for normal distribution and homogeneity of the variances and then reported as mean and SEM. Differences among the groups were analyzed using 1-way analysis of variance (ANOVA) followed by a post-hoc test of Tukey. A P value ≤ .05 was considered statistically significant (Prism version 5.03 for Windows; GraphPad Software).
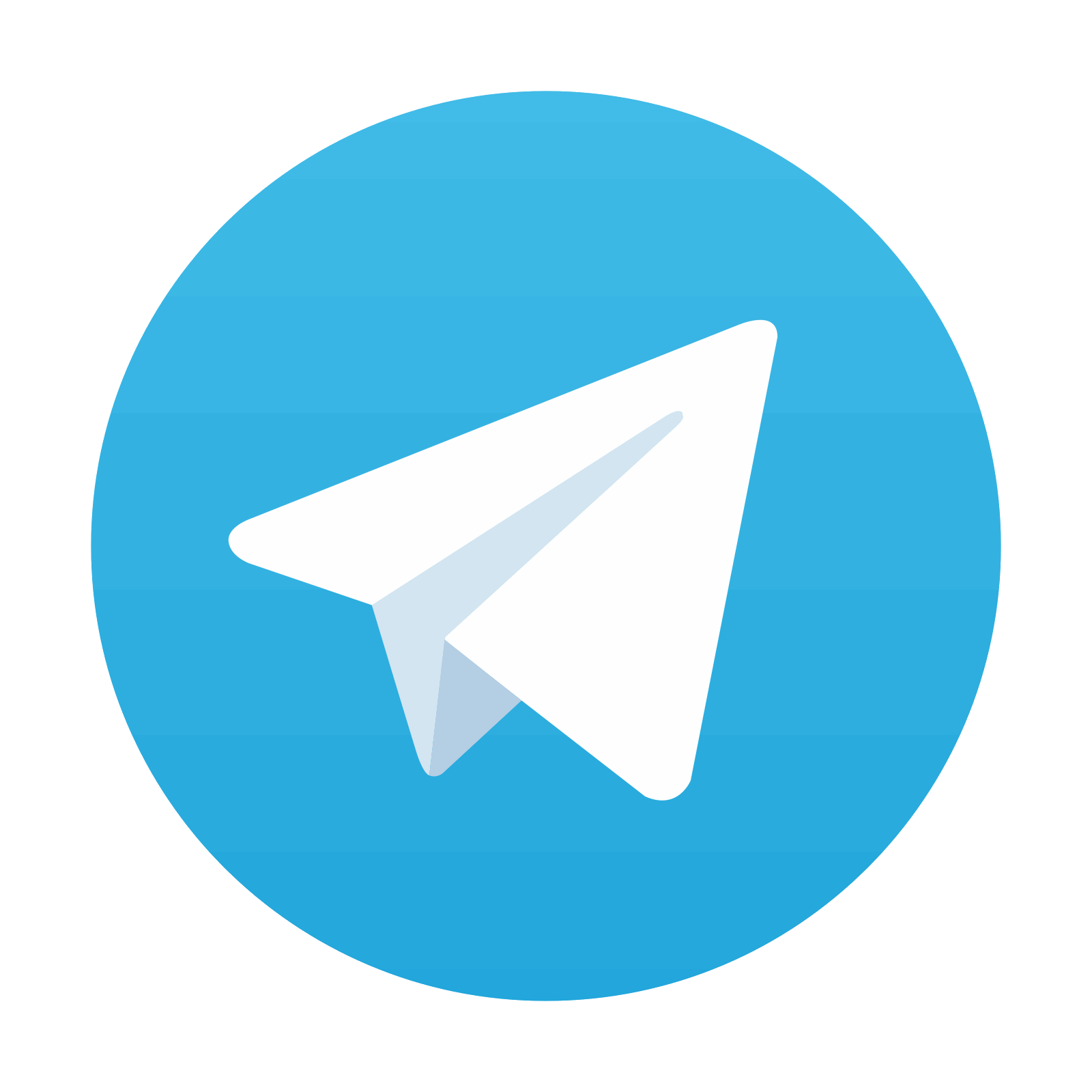
Stay updated, free articles. Join our Telegram channel

Full access? Get Clinical Tree
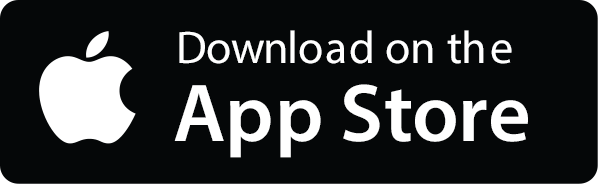
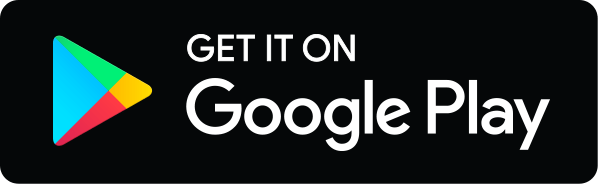