Key Abbreviations
Antigen-presenting cell APC
B-cell activating factor of the tumor necrosis factor family BAFF
B-cell receptor BCR
CC receptor CCR
Chemokine (C-C motif) ligand 5 CCL5
CXC receptor CXCR
Decidual natural killer dNK
Dendritic cell DC
Fas ligand FasL
Graft-versus-host disease GVHD
Human chorionic gonadotropin hCG
Human immunodeficiency virus HIV
Human leukocyte antigen HLA
Indoleamine 2,3 dioxygenase IDO
Immunoglobulin Ig
Interferon-γ IFN-γ
Interleukin IL
Kilodalton kDa
Lipopolysaccharide LPS
Lipopolysaccharide binding protein LBP
Major histocompatibility complex MHC
Membrane attack complex MAC
Microchimerism Mc
Monocyte chemotactic protein 1 MCP-1 or CCL2
Natural killer NK
NOD-like receptor NLR
Pattern-recognition receptor PRR
Peripheral T-regulatory cell pT REG
Programmed death 1 receptor PD-1
Regulated on activation, normal T-cell expressed and secreted RANTES (CCL5)
Regulatory B cell B REG or B10
Regulatory T cell T REG
Rheumatoid arthritis RA
T-cell receptor TCR
T Helper cell type 1 Th1
T Helper cell type 2 Th2
Thymic T-regulatory cell tT REG
TNF-related apoptosis-inducing ligand/Apo-2L TRAIL
Toll-like receptor TLR
Transforming growth factor beta TGF-β
Tumor necrosis factor alpha TNF-α
Vascular endothelial growth factor VEGF
Pregnancy poses unique immunologic challenges to the mother, who must become tolerant to a genetically foreign fetus yet remain immunocompetent to fight infection. Immunology is one of the fastest moving fields in medical science with many recent advances in the understanding of immunologic changes in pregnancy. The study of maternal-fetal immunology was initially driven by a desire to understand how such a paradoxical feat could occur naturally. Sir Peter Medawar suggested several possibilities to explain fetal tolerance by the mother, including anatomic separation of the fetus and mother, antigenic immaturity of the fetus, and immunologic inertness of the mother. Over time, research has revealed that none of these explanations were adequate for several reasons. First, maternal and fetal cells were discovered to come into contact with each other throughout pregnancy; therefore neither the mother nor the fetus is truly anatomically separated from the other. Small populations of fetal cells in the mother and maternal cells in the fetus can persist for decades after pregnancy, which is known as microchimerism (Mc). Secondly, the fetus is not antigenically immature. Fetal immune cells in the skin can elicit potent immune responses. Other fetal immune cells become highly specialized to suppress the fetal immune system and prevent reactivity toward maternal microchimeric cells that enter the fetus. Finally, the mother is not immunologically inert; maintaining the ability to recognize pathogens and fight infection is paramount to her survival during pregnancy. Instead, the maternal immune system has developed an elaborate strategy to become more flexible to what she considers “self” during pregnancy in order to prevent immunologic attack of the fetus. The study of pregnancy immunology has revealed many fascinating mechanisms at work to achieve and maintain fetal tolerance during pregnancy while still allowing for normal immune defense.
In this chapter, we focus on describing pregnancy immunology as it relates to normal pregnancy and obstetric complications. In some perinatal conditions, the study of pregnancy immunology is central to the discovery of better diagnostic strategies and therapies. For example, preterm labor associated with infection is characterized by immunologic proteins in the blood, amniotic fluid, and vaginal fluid, which are thought to play a major role in triggering labor. Understanding the functions of the immune system and individual immune cells as they relate to maternal tolerance of the fetus, preterm birth, preeclampsia, pregnancy loss, and common perinatal infections will allow the clinician to gain a deeper appreciation for normal and abnormal pregnancy.
Immune System Overview: Innate and Adaptive Immunity
The immune system is classically divided into two arms, the innate ( Fig. 4-1 ) and adaptive ( Fig. 4-2 ) immune systems . Each arm of the immune system fights infection by a slightly different and complementary method. Both systems have several important mechanisms to prevent maternal immunity from targeting and killing the fetus, yet the immune system must remain competent to overcome an infection to preserve the mother’s life. Achieving a balance between controlling normal immune responses and maintaining immune function is a major challenge of pregnancy.


The innate immune system uses fast, nonspecific methods of pathogen detection to prevent and control an initial infection. Innate immunity consists of immune cells such as macrophages, dendritic cells (DCs), natural killer (NK) cells, eosinophils, and basophils. In pregnancy, these cells have been implicated in preterm labor, preeclampsia, maternal-fetal tolerance, and intrauterine growth restriction (IUGR). Many of these cells identify pathogens through pattern-recognition receptors (PRRs) that recognize common pathogen structures such as lipoteichoic acid and lipopolysaccharide (LPS), constituents of the cell walls of gram-positive and gram-negative bacteria. PRRs include the macrophage mannose receptor and Toll-like receptors (TLRs), a large family of PRRs likely responsible for the earliest immune responses to a pathogen. TLR activation is often triggered by components of bacterial cell walls, which initiate a signaling cascade that leads to release of cytokines. Cytokines are small immunologic proteins implicated in the pathogenesis of preterm labor. Another component of innate immunity is complement, a system of plasma proteins that coat pathogen surfaces with protein fragments to target them for destruction.
In many cases, innate immune defenses are effective in combating pathogens. Sometimes, pathogens may evolve more rapidly than the hosts they infect, or they evade innate immune responses, like seasonal influenza viruses. The adaptive immune system must then act to control infection. Adaptive immunity results in the clonal expansion of lymphocytes (T cells and B cells) and antibodies against a specific antigen. Although slower to respond, adaptive immunity targets specific components of a pathogen and is capable of eradicating an infection that has overwhelmed the innate immune system. Adaptive immunity also requires presentation of antigen by specialized antigen-presenting cells (APCs), production and secretion of stimulatory cytokines, and ultimately, amplification of antigen-specific lymphocyte clones (T cells and B cells). These memory T and B cells provide lifelong immunity to the specific antigen.
Innate Immunity: First Line of Host Defense
Epithelial surfaces of the body are the first defenses against infection. Mechanical epithelial barriers to infection include ciliary movement of mucus and epithelial cell tight junctions that prevent microorganisms from easily penetrating intercellular spaces. Chemical mechanisms of defense include enzymes (e.g., lysozyme in saliva, pepsin), low pH in the stomach, and antibacterial peptides (e.g., defensins in the vagina) that degrade bacteria.
After a pathogen enters the tissues, it is often recognized and killed by phagocytes, a process mediated by macrophages and neutrophils. TLRs, a family of PRRs on the surface of macrophages and other innate immune and epithelial cells, represent a primary mechanism of pathogen detection. TLR activation results in secretion of cytokines that initiate inflammatory responses. Nucleotide-binding oligomerization domain receptors (NOD-like receptors, NLRs) are also PRRs, and they operate inside the cell to recognize pathogen structures once they have entered the cell through phagocytosis or via pores. NLRs can cooperate with TLRs to initiate or regulate an inflammatory or apoptotic response. Cytokines and chemokines such as interleukin-8 (IL-8) are released after activation of PRRs to recruit neutrophils to sites of inflammation; they also coordinate many immune functions that include cell activation, replication, and differentiation. Proinflammatory cytokines have been described in the mother and fetus and in the amniotic fluid of women with preterm labor and intraamniotic infection.
Antimicrobial Peptides
Antimicrobial peptides are secreted by neutrophils and epithelial cells to kill bacteria by damaging pathogen membranes. Defensins are a major family of antimicrobial peptides that protect against bacterial, fungal, and viral pathogens. Neutrophils secrete α-defensins, and epithelial cells in the gut and lung secrete β-defensins. Both α- and β-defensins are temporally expressed by endometrial epithelial cells during the menstrual cycle. Susceptibility to upper genital tract infection may be related in part to the decreased expression of antimicrobial peptides in response to hormonal changes during the menstrual cycle. Many other tissues of the female reproductive tract and the placenta secrete defensins, including the vagina, cervix, fallopian tubes, decidua, and chorion. Elevated concentrations of vaginal and amniotic fluid defensins have been associated with intraamniotic infection and preterm birth .
Macrophages
Macrophages mature from circulating monocytes that leave the circulation to migrate into tissues throughout the body. Macrophages have critical scavenger functions that likely help to prevent bacteria from establishing an intrauterine infection during pregnancy. Macrophages are one of the most abundant immune cell types in the placenta and can directly recognize, ingest, and destroy pathogens. Pathogen recognition may occur through PRRs such as TLRs, scavenger receptors, and mannose receptors. Macrophages also internalize pathogens or pathogen particles through phagocytosis, macropinocytosis, and receptor-mediated endocytosis. Multiple receptors on the macrophage can induce phagocytosis, including the mannose receptor, scavenger receptor, CD14, and complement receptors. Macrophages also release many bactericidal agents after ingesting a pathogen, such as oxygen radicals, nitric oxide, antimicrobial peptides, and lysozyme.
Uterine macrophages represent up to one third of the total leukocytes in pregnancy-associated tissue during the later parts of pregnancy and perform many critical functions to support the pregnancy. Macrophages are a major source of inducible nitric oxide synthetase, a rate-limiting enzyme for nitric oxide production. During pregnancy, nitric oxide is thought to relax uterine smooth muscle, and uterine nitric oxide synthetase activity and expression decreases before parturition. Uterine macrophages are also a major source of prostaglandins, inflammatory cytokines, and matrix metalloproteinases that are prominent during term and preterm parturition. Throughout pregnancy, macrophages are also in close proximity to invading trophoblasts that establish placentation. Placental growth involves trophoblast remodeling and programmed cell death (apoptosis). Macrophages in the placenta phagocytose apoptotic trophoblast, which also programs the macrophage to release antiinflammatory cytokines (e.g., IL-10) promoting fetal tolerance.
Natural Killer Cells
The NK cell has important functions during pregnancy and becomes the most abundant leukocyte in the pregnant uterus. NK cells differ from T and B cells in that they do not express clonally distributed receptors for foreign antigens and can lyse target cells without prior sensitization. The phenotype of decidual NK (dNK) cells is different from that of NK cells in peripheral blood, which seems to correlate with different primary functions. Most NK cells in blood (90%) have low CD56 and high CD16 expression (CD56 dim /CD16 bright ); in the uterine decidua, dNK cells have high CD56 expression (CD56 bright ). The level of CD56 expression determines whether an NK cell has a primary cytolytic (CD56 dim ) or cytokine-producing (CD56 bright ) function. During pregnancy, dNK cells are the predominant decidual immune cell with peak levels (~85%) in early pregnancy that gradually decline by mid gestation but that remain at approximately 50% of total decidual immune cells. In addition, dNK cells are thought to play a major role in the remodeling of the spiral arteries to establish normal placentation. Mice with genetically defective or low numbers of dNK cells fail to undergo spiral artery remodeling and normal development of the decidua, which are critical processes for normal placentation (see Chapter 1 ). This defect is corrected with administration of interferon-γ (IFN-γ), a prominent NK cell cytokine, which suggests that dNK cells play an important role in the angiogenesis necessary for trophoblast invasion. The cytolytic activity of dNK cells is low and is further inhibited by interactions with human leukocyte antigen G (HLA-G).
Toll-Like Receptors
TLRs are a recently discovered large family of PRRs on macrophages and many other cell types that play a key role in innate immunity. TLRs are now recognized as the principal early sensors of pathogens that can activate both the innate and adaptive immune system. Ten functional toll homologues are found in humans, and they recognize a wide range of pathogen ligands (see Fig. 4-1, B ). TLR4 is a TLR that recognizes LPS from gram-negative bacteria, which triggers a signaling cascade that leads to cytokine gene expression ( Fig. 4-3 ). TLR4 is expressed on macrophages, dendritic cells, endothelium, and numerous epithelial tissues. TLR2 recognizes motifs from gram-positive bacteria, including lipoteichoic acid and peptidoglycan. Several bacteria evade TLR recognition by producing proteins or LPS mutants that interfere with TLR signaling. For example, Yersinia pestis —the bacteria responsible for plague—expresses a tetraacetylated LPS that is poorly recognized by TLR4 and results in TLR4 antagonism. Brucella abortus , known to induce recurrent abortion in cattle, produces at least two proteins that are potent inhibitors of TLR signaling, which gives the bacteria a survival advantage in evading immune detection.

Expression of both TLR2 and TLR4 has been demonstrated in the placenta, and first-trimester trophoblast cells express both TLR2 and TLR4. Activation of TLR2 triggers Fas-mediated apoptosis, whereas TLR4 activation induces proinflammatory cytokine production. The immunologic capability of first-trimester trophoblast cells to recognize pathogens and induce apoptosis suggests that innate immunity may be an important placental mechanism for triggering spontaneous abortion. TLR4 is also expressed in villous macrophages, villous and extravillous trophoblast, and the amniochorion. Expression of TLR4 and TLR2 increases in the chorioamniotic membranes of women with intraamniotic infection and also in term labor. Although intrauterine injection of LPS induces preterm birth in many murine and nonhuman primate models, administration of LPS to TLR4 mutant mice or LPS blockade with a TLR4 antagonist does not result in preterm delivery. This finding suggests that TLR4 is required for LPS-induced preterm birth in mice and that it is an important driver of the inflammatory cascade that results from intraamniotic infection.
Maturation of TLR expression in the fetal membranes over time may explain the tendency for infection-associated preterm births to occur no earlier than the late second or early third trimester. Although TLR4 is expressed in the cytoplasm of amniotic epithelium in the first trimester, not until 25 weeks is there TLR4 expression on the apical membrane, which is in contact with amniotic fluid and potential pathogens. A similar ontogeny in TLR4 expression is seen in the fetal lung. When mouse fetal lung is exposed to LPS on fetal day 14 (term is 20 days), TLR4 expression and cytokines are undetectable. By day 17, TLR4 is expressed and an acute cytokine response occurs in fetal lungs. TLR4 likely controls the magnitude of the LPS-induced cytokine response during the perinatal period, and TLR4 placental expression is dependent on gestational age.
Complement System
An important component of the innate immune system is the complement system, which consists of a large number of plasma proteins that cooperate to destroy and facilitate the removal of pathogens (see Fig. 4-1, C ). Complement proteins are detected in the amniotic fluid during intraamniotic infection, and regulation of complement is necessary to protect placental and fetal tissues from inflammation and destruction. The nature of the initial pathogen trigger determines one of three activation pathways: 1) classic, 2) alternate, and 3) lectin-binding pathways. For example, the classic pathway of complement activation is triggered when the complement protein C1q binds to antigen-antibody complexes on the surface of pathogens. This binding then results in a series of activation and amplification steps that result in production of the membrane attack complex (MAC), which creates a pore in the pathogen membrane and leads to cell lysis. Formation of the MAC is an important mechanism of host defense against Neisseria species. Genetic deficiencies in C5-C9 complement proteins have been associated with susceptibility to N. gonorrhoeae and N. meningitidis.
Regulatory proteins exist to protect cells from the deleterious effects of complement and are expressed on the placental membranes. Placental tissues at the maternal-fetal interface strongly express several negative regulators of complement activation, including CD59 (MAC antagonist), membrane cofactor protein, and decay-accelerating factor (inhibitor of C3 and C5 convertases). Whether these regulatory proteins might become overwhelmed during an intraamniotic infection, leading to weakening of the membranes by complement proteins, is unknown.
Cytokines
The release of cytokines and chemokines by macrophages and other immune cells represents an important induced innate immune response ( Table 4-1 ; see Fig. 4-1, D ). Activated macrophages secrete cytokines—IL-1β, IL-6, IL-12, and tumor necrosis factor alpha (TNF-α)—that initiate inflammatory responses to control infections. These cytokines are often referred to as proinflammatory because they mediate fever, lymphocyte activation, tissue destruction, and shock. Higher levels of several cytokines and chemokines have been implicated in the increased morbidity and mortality with influenza during pregnancy. In lung homogenates of infected pregnant mice, levels of IL-6 and IL-8; regulated on activation, normal T-cell expressed and secreted (RANTES [CCL5]); and monocyte chemotactic protein 1 (MCP-1 [CCL2]) were higher after infection with the 2009 H1N1 influenza virus strain. Dramatic elevations in IL-6 have also been implicated in deaths, such as in the 1918 influenza virus, with an estimated mortality in pregnancy of 27%. An increase in cytokine levels is likely not the only explanation for increased morbidity and mortality from influenza infection during pregnancy. Recently, enhanced NK- and T-cell responses to influenza vaccination in pregnant women were reported, which suggests that robust cellular immune responses also play a role.
CYTOKINE | PRODUCED BY | PRIMARY ACTION |
---|---|---|
Interferons | Monocytes and macrophages | Produced in response to viruses, bacteria, parasites, and tumor cells |
Action includes killing tumor cells and inducing secretion of other inflammatory cytokines | ||
One of the first cytokines to appear during an inflammatory response | ||
Interleukin-1 | Monocytes and macrophages | Induces fever; costimulator of CD4 + helper T cells |
Interleukin-2 | Activated T cells | Primary growth factor and activation factor for T cells and natural killer cells |
Interleukin-4 | CD4 + helper T cells | B-cell growth factor for antigen-activated B cells |
Interleukin-6 | Monocytes and macrophages | Regulates growth and differentiation of lymphocytes and growth factor for plasma cells and induces the synthesis of acute-phase reactants by the liver |
Interleukin-8 | Monocytes | Chemoattractant for neutrophils |
Interleukin-10 | CD4 + helper T cells | Suppresses production of interferon, suppresses cell-mediated immunity, enhances humoral immunity |
Transforming growth factor β | T cells and monocytes | Inhibits the proliferation of lymphocytes |
During normal pregnancy, many cytokines become repressed with advancing gestation, including IFN-γ, vascular endothelial growth factor (VEGF), MCP-1 (CCL2), and eotaxin. TNF-α and granulocyte colony-stimulating factor (G-CSF) levels increase slightly with advancing gestation, which is surprising because both have been linked to proinflammatory responses, and maintaining uterine quiescence during pregnancy is thought to require repression of inflammation. Proinflammatory cytokines such as IL-1β, TNF-α, and IL-6 have also been identified in the amniotic fluid, maternal and fetal blood, and vaginal fluid of women with intraamniotic infection at much higher levels than that observed during normal pregnancy. These cytokines not only serve as a marker of intraamniotic infection, they may trigger preterm labor and lead to neonatal complications. The fetal inflammatory response syndrome describes the connection between elevated proinflammatory cytokines in fetal blood, preterm labor, and increased adverse fetal outcomes (see Chapter 29 ).
The relative contribution of individual cytokines and chemokines to preterm labor was studied in a unique nonhuman primate model. Preterm labor was induced by intraamniotic infusions of IL-1β and TNF-α but not by IL-6 or IL-8. IL-1β stimulated preterm labor in all cases and was associated with an intense contraction pattern. TNF-α induced a variable degree of uterine activity characterized as preterm labor in some animals or as a uterine contraction pattern of moderate intensity. Despite prolonged elevations in amniotic fluid levels, neither IL-6 nor IL-8 induced an increase in uterine contractions until near term. These results suggest a primary role for IL-1β and TNF-α in the induction of infection-associated preterm birth. Recent data suggest that parturition and prostaglandin mRNA expression was delayed in IL-6 null mutant mice by 1 day compared with wild-type mice. Furthermore, LPS did not induce preterm birth in IL-6–null mutant mice, in contrast to wild-type mice. Together, these data indicate that IL-6 plays a role in triggering normal parturition, perhaps in activation of labor pathways.
Investigation of the individual effect of a single cytokine on pregnancy or complications of pregnancy in humans has proved challenging for several reasons. Many cytokines tend to be functionally redundant, with one cytokine compensating for the absence of another. Second, multiple cytokine receptors (i.e., interleukin-1 [IL-1] receptor antagonist, IL-18 binding protein) modulate similar cytokine effects. New families of decoy or silent cytokine receptors and suppressors of cytokine signaling have also been discovered in the placenta and amniotic fluid. Finally, molecular variants of cytokines may act as receptor antagonists. Therefore individual cytokine effects during pregnancy must be interpreted in the context of cytokine receptors, receptor antagonists, silent cytokine receptors, and suppressors of cytokine signaling.
Chemokines
Chemokines are a class of cytokines that act primarily as chemoattractants that direct leukocytes to sites of infection. These chemotactic agents constitute a superfamily of small (8 to 10 kDa) molecules that can be divided into three groups—C, CC, and CXC—based on the position of either one or two cysteine residues located near the amino terminus of the protein. IL-8, CCL2 (also known as MCP-1), and RANTES (CCL5) are a few examples of chemokines. CXC chemokines, such as IL-8, bind to CXC receptors (CXCRs) and are important for neutrophil activation and mobilization. Increases in IL-8 levels have been described in the amniotic fluid, maternal blood, and vaginal fluid with infection-associated preterm birth. IL-8 and CCL2 have also been implicated in uterine stretch-induced preterm labor thought to occur in multiple gestation.
Some chemokine receptors are used as a coreceptor for the viral entry of the human immunodeficiency virus (HIV; see Chapter 53 ). The two major chemokine co-receptors for HIV are CXCR4 and CCR5, both of which are expressed on activated T cells. CCR5 is also expressed on DCs and macrophages, which allows HIV to infect these cell types. Rare resistance to HIV infection was discovered to correlate with homozygosity for a nonfunctional variant of CCR5 caused by a gene deletion in the coding region. The gene frequency for this CCR5 variant is highest in Northern Europeans but has not been detected in many black or Southeast Asian populations, in whom the prevalence of HIV infection is high. CCR3 is another chemokine coreceptor for HIV that is expressed by microglia, and it can be used by some HIV strains to infect the brain.
Adaptive Immunity
The function of the adaptive immune system is to eliminate infection as the second line of immune defense and to provide increased protection against reinfection through immunologic “memory.” Adaptive immunity comprises primarily B cells and T cells (lymphocytes), which differ from innate immune cells in several important respects, including the mechanism for pathogen recognition and lymphocyte activation. Targeting a specific pathogen component in an immune response is a critical feature of the adaptive immune system and is necessary, in most cases, for resolution of the infection. However, achieving this specificity requires generation of an incredible diversity of T-cell receptors (TCRs) and B-cell receptors (BCRs). This creates the potential for self-antigens to be mistakenly targeted, resulting in an autoimmune response. Self-reactive T cells and B cells are thought to either undergo apoptosis in the thymus or to be regulated in the periphery. A small population of regulatory T cells contributes to peripheral regulatory mechanisms to prevent autoimmune responses and is discussed specifically in reference to mechanisms of fetal tolerance.
Major Histocompatibility Complex
Discriminating cells that are “self” from those that are “nonself” is a critical function of the immune system to determine which cells should be destroyed and which to leave alone. In pregnancy, this process must be carefully regulated to prevent the killing of fetal cells, which express paternal genes that appear foreign to the maternal immune system; this in effect expands the maternal immune system’s definition of “self” to include the fetus. The ability of a lymphocyte to distinguish self from nonself is based on the expression of unique major histocompatibility complex (MHC) molecules on a cell’s surface, which present small peptides from within the cell. MHC molecules are highly polymorphic proteins produced by a cluster of genes on the short arm of chromosome 6. This gene complex is classically divided into two distinct regions referred to as class I and class II . Class I contains classical transplantation HLA genes (e.g., HLA-A, -B, and -C ) and nonclassical HLA genes distinguished by more limited polymorphism (e.g., HLA-G, -E, and -F ) . Class II contains polymorphic genes that are often matched for transplantation, including those of the HLA-DR, -DQ, and -DP families of genes. Reduced HLA matching is associated with graft rejection after transplantation through activation of T cells. This system differs significantly from the innate immune system, in which recognition of MHC is not necessary for pathogen destruction.
Humoral Immune Responses: B Cells and Antibodies
The function of B cells is to protect the extracellular spaces (e.g., plasma, vagina) in the body through which infectious pathogens usually spread (see Fig. 4-2, A ). B cells mainly fight infection by secreting antibodies, also called immunoglobulins . Many similarities are found between B and T lymphocytes. Like T cells, B cells also undergo clonal expansion after antigen stimulation and can be identified by a variety of specific cell surface markers (e.g., CD19, CD20, and BCR antigens). Activated B cells may proliferate and differentiate into antibody-secreting plasma cells. Antibodies control infection by several mechanisms that include neutralization, opsonization, and complement activation. Neutralization of a pathogen refers to the process of antibody binding, which prevents the pathogen from binding to a cell surface and internalizing. Alternatively, antibodies that coat the pathogen may enhance phagocytosis, also referred to as opsonization. Antibodies may also directly activate the classical complement pathway. Activation of the B cell drives the B cell to proliferate and differentiate into an antibody-secreting plasma cell.
Recently it was discovered that profound changes occur in many types of B cells during pregnancy. Immature B cells that are the precursors to antigen-specific mature B cells are significantly reduced with advancing gestation in the maternal bone marrow, blood, and spleens of pregnant mice. Lymphopoiesis of B cells is reduced during pregnancy, which may be mediated by the normal pregnancy rise in estradiol. Estradiol reduces levels of IL-17, a critical factor necessary for B-cell production in the bone marrow. This reduction in immature B cells is further potentiated during the second half of pregnancy by the antigen-induced deletion of immature B cells. Although immature B cells are reduced during pregnancy, the number of mature B cells is significantly increased. Surprisingly, an increased number of mature B cells are found in the lymph nodes that drain the uterus. Overall, pregnancy is associated with profound changes in the numbers of B cells in several compartments .
Autoantibodies produced by B cells against angiotensin receptor I, known as AT1-AA, are thought to play a role in inducing hypertension and proteinuria in women with preeclampsia and fetal growth restriction. AT1-AA is present in 70% to 95% of women with preeclampsia, and antibody titer is correlated with disease severity. AT1-AA can bind to endothelial and placental cells in vitro to induce oxidative stress and cytokine and endothelin production. Transfer of these autoantibodies from women with preeclampsia can also induce hypertension and proteinuria in pregnant mice. Although a wide spectrum of immunologic abnormalities is found in preeclampsia, the concept that an autoantibody can cause disease in pregnancy is well established. For example, Graves disease is the most common causes of thyrotoxicosis in pregnancy (see Chapter 42 ). More than 80% of individuals with Graves disease have anti–thyroid stimulating hormone (TSH) receptor autoantibodies. B cells are likely beneficial in establishing fetal tolerance but may also contribute to the pathogenesis of certain obstetric complications, such as preeclampsia.
Antibody Isotypes
Antibodies share the same general structure produced by the interaction and binding of four separate polypeptides ( Fig. 4-4 ). These include two identical light (L) chains (23 kDa) and two identical heavy (H) chains (55 kDa) . The composition of the H chain determines the antibody isotype, function, and distribution in the body. In humans, there are five types of H chains—designated mu (M), delta (D), gamma (G), alpha (A), and epsilon (E)—that correspond to the five major antibody isotypes (immunoglobulin M [IgM], IgD, IgG, IgA, and IgE). To effectively combat extracellular pathogens, antibodies must be specialized to cross epithelia into different bodily compartments. In fact, antibodies are made in several distinct classes or isotypes (i.e., IgM and IgG) that vary in their composition. Naïve B cells express only IgM and IgD. Activated B cells undergo isotype switching, a process that produces different antibody isotypes specialized for different functions and areas of the body.

The first antibody to be produced during an immune response is IgM because it is expressed before isotype switching. The serum concentration of IgM is 50 to 400 mg/dL, with a circulation half-life of 5 days. IgM antibodies are low in affinity, but the antibodies form pentamers that compensate by binding at multiple points to the antigen. IgM is highly efficient at activating the complement system, which is critical during the earliest stages of controlling an infection. Other isotypes dominate in the later stages of antibody responses.
IgG represents about 75% of serum immunoglobulin in adults and is further divided into four subclasses: IgG1, IgG2, IgG3, and IgG4. Two subtypes of IgG, IgG1 and IgG3, are efficiently transported across the placenta and are important in conferring humoral immune protection for the fetus after birth. The smaller size of IgG and its monomeric structure allows it to easily diffuse into extravascular sites. In mice, the level of IgG3 and IgM increase in early pregnancy but decline as pregnancy advances.
IgA is the predominant antibody class in epithelial secretions from the vagina, intestine, and lung. IgA forms dimers and mainly functions as a neutralizing antibody. As a secreted antibody, IgA is not in close contact with either phagocytes or complement and, therefore, is less efficient in opsonization and complement activation. IgA is the principal antibody in breast milk, which provides the neonate with humoral immunity from the mother (see Chapter 24 ). Neonates are particularly susceptible to infectious pathogens through their intestinal mucosa, and IgA is highly effective in neutralizing these bacteria and toxins. Epidemiologic studies indicate that deaths from diarrheal diseases could be reduced between 14- and 24-fold by breastfeeding, owing in part to the maternal-infant transmission of IgA. Levels of IgA rise toward the end of pregnancy in pregnant mice.
IgE has the lowest concentration in serum of all the antibodies but is bound efficiently by mast cell receptors. IgE binding of antibody triggers the mast cell to release granules, which results in an allergic response. Prenatal maternal exposure to allergens may have an effect on IgE in the fetus at birth; concentration of house dust mite allergens has been correlated in a dose-dependent manner with total IgE measured in neonatal heel capillary blood. IgE also plays a prominent role in immune responses to eukaryotic parasites.
T Cells
When pathogens replicate inside cells (all viruses, some bacteria and parasites), they are inaccessible to antibodies and must be destroyed by T cells. T cells are lymphocytes responsible for the cell-mediated immune responses of adaptive immunity, which require direct interactions between T lymphocytes and cells bearing the antigen that the T cells recognize. Common to all mature T cells is the TCR complex. T cells develop a vast array of antigen specificity through a series of TCR gene rearrangements, and many aspects of TCR rearrangements are similar to those that produce antibody specificity. For example, during viral replication inside a host cell, viral antigen is expressed on the surface of the infected cell. T cells, along with HLA, then recognize these foreign antigens. HLA class I molecules present peptides from proteins in the cytosol, which may include degraded host or viral proteins. HLA class II molecules bind peptides derived from proteins in intracellular vesicles and thus display peptides that originate from pathogens in macrophage vesicles internalized by phagocytic cells and from B cells.
A variety of T cells are recognized based on their expression of different cell surface markers (i.e., CD2, CD3, CD4, CD8). Cytotoxic T cells kill infected cells directly and express a variety of cell surface antigen and specific receptors, including CD8. Helper T cells activate B cells and express CD4. Cytotoxic and helper T cells recognize peptides bound to proteins of two different classes of HLA molecules (see Fig. 4-2, B ). APCs will present antigen to CD8 + T cells in the context of MHC class I molecules (e.g., HLA-A). In contrast, antigen-presenting cells that present antigens with MHC class II molecules (e.g., HLA-DR) interact with T cells bearing CD4.
HIV uses multiple strategies to disable T-cell responses, mainly by targeting CD4 + T cells. Targeting viral infection to CD4 + T cells allows the virus to control and ultimately destroy this important T-cell subset. HIV destroys CD4 + T cells through direct viral killing, which lowers the apoptosis threshold of infected cells, and through CD8 + T cells that recognize viral peptides on the CD4 + T-cell surface. CD8 + T cells likely contain the infection but are unable to eradicate the virus. Viral mutants produced during one of the earliest steps of viral infection may contribute to the escape of virus-infected cells from CD8 + T-cell killing. HIV also has an error-prone reverse transcriptase that copies the viral RNA genome into DNA, making “mistakes” that lead to production of viral variants. The presentation of peptides from HIV variants by CD4 + T cells may also interfere and downregulate the CD8 + T-cell response to the original (wild-type) virus. Finally, the HIV negative-regulation factor gene (nef) downregulates expression of MHC class II and CD4, which decreases the presentation of viral antigens on the cell surface.
Helper T-Cell Subsets
CD4 + T cells were originally classified into T-helper 1 (Th1) and T-helper 2 (Th2) subsets depending on whether their main function involved cell-mediated responses and selective production of IFN-γ (Th1) or humoral-mediated responses with production of IL-4 (Th2). The number of subsets identified continues to expand and now includes regulatory T cells (T REG ), Th17, follicular helper T cells (T FH ), Th22, and Th9. The most well-characterized subsets are Th1 and Th2. The Th1 subset is important in the control of intracellular bacterial infections such as Mycobacterium tuberculosis and Chlamydia trachomatis. Intracellular bacteria survive because the vesicles they occupy do not fuse with intracellular lysosomes, which contain a variety of enzymes and antimicrobial substances. Th1 cells activate macrophages to induce fusion of their lysosomes with vesicles that contain the bacteria. Th1 cells also release cytokines and chemokines that attract macrophages to the site of infection, like IFN-γ, TNF-α, IL-12, and IL-18. Th2 immune responses are mainly responsible for activating B cells by providing a critical “second signal.” Th2 cells produce cytokines that include IL-4, -5, -6, -10, and -13 and transforming growth factor beta (TGF-β). Although CD4 + T cells can be described in a discrete manner using named subsets like Th1 or Th2, overlap between the functions of these cells is likely.
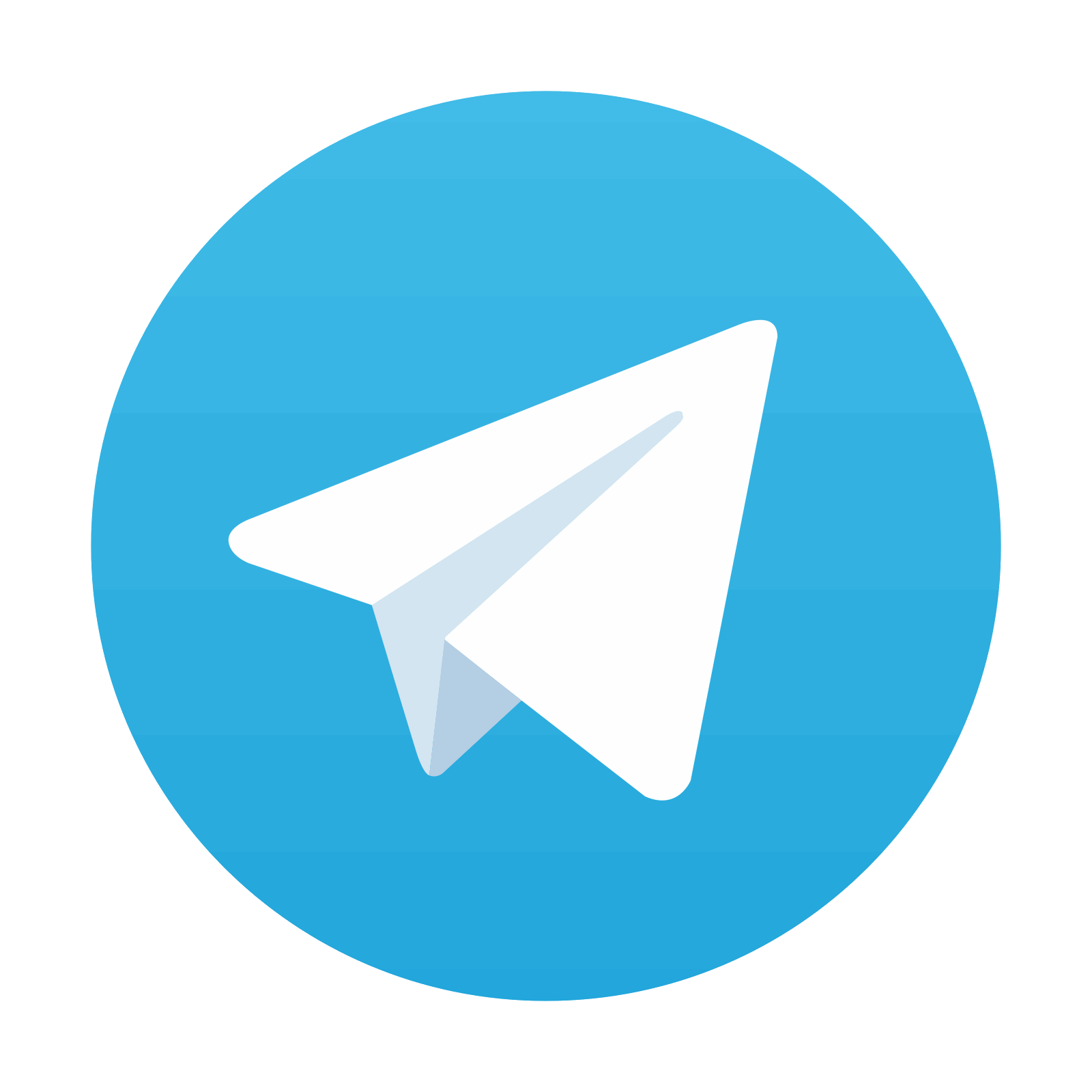
Stay updated, free articles. Join our Telegram channel

Full access? Get Clinical Tree
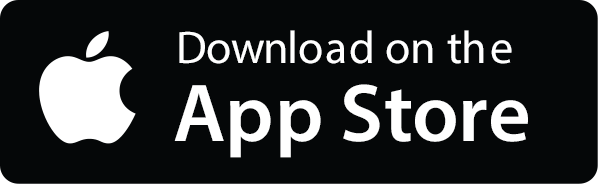
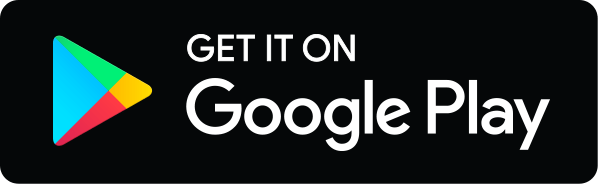