Maternal Drug Intake and the Newborn
Nada Djokanovic
Rada Boskovic
Gideon Koren
Introduction
The use of prescription drugs during pregnancy has become a concern since the thalidomide tragedy. However, medication use by pregnant women and females of reproductive age is common, and often necessary and unavoidable in chronic conditions. Recent studies have suggested that between 64% and 83% of women use at least one medication during pregnancy (1,2,3). The most commonly prescribed drugs in pregnancy include anti-infectives, analgesics, and drugs for the respiratory system. Recent Canadian study found that one in every five pregnant women was exposed to prescription drugs with potential fetal risk (4). Because, approximately 50% of pregnancies are unplanned, women whose pregnancies are unintended and unexpected are more likely to be exposed to a wide range of potential teratogens (5,6).
It has been assumed for decades that the human placenta serves as a barrier between mother and fetus, protecting the fetus from exposure to xenobiotics circulating in the mother; however, the thalidomide-induced embryopathies, recognized in 1960, reversed this concept. With the development of appropriate analytical methods, it has become apparent that the placenta not only allows most xenobiotics and their metabolites to cross from the maternal to the fetal circulation, but that it also has an extensive metabolizing capacity and can itself serve also as a site storage for chemicals (7).
The devastating lifelong impact an adverse pregnancy outcome may have on the infant reinforces the critical need for more knowledge about the pharmacokinetics of the maternal–placental–fetal unit. This chapter reviews mechanisms and factors that are involved in the transfer of pharmacologically active substances from the mother to the fetus, undesirable effects of known teratogens, and methods available to study placental drug transfer.
Pharmacokinetics of the Maternal–Fetal Unit
One cannot discuss the pharmacokinetics of the maternal–fetal unit without considering the placental anatomy and pregnancy-induced physiologic changes.
Placental Anatomy
The placenta is a multifaceted organ that plays critical roles in optimal growth and development of the embryo and fetus during pregnancy. It is virtually the sole contact that the fetus has with the mother. The placenta not only “supplies” the embryo and fetus with oxygen, water, electrolytes, and nutrients, but also “excretes” the waste products from the fetus (8). It is also an endocrine organ that produces various steroid hormones (e.g., estrogens and progesterone) and polypeptide hormones (e.g., chorionic gonadotropin and placental lactogen) that are vital for successful pregnancy.
Shortly after fertilization, the zygote (fertilized ovum) undergoes rapid mitotic cell division to form the morula. The outer cells of the morula, called the trophoblast, contribute to the formation of the placenta, and a group of centrally located cells, known as the inner cell mass (embryoblast), differentiate to form the fetus. There are two layers of trophoblastic cells: the syncytiotrophoblast does not undergo mitosis and the cytotrophoblast continuously replenishes the growing mass of the syncytiotrophoblast (9). Placental functions are generally related to the syncytiotrophoblast (10). The syncytiotrophoblast consists of two distinct transport regions: a brush border membrane that is facing the maternal side and a basal membrane that is facing the fetal side (11). The transfer of any compound from the maternal blood into fetal blood across the syncytiotrophoblast has to involve transport across the brush border membrane followed by transport across the basal membrane, and vice versa in the case of transfer from the fetal to maternal blood. As the syncytiotrophoblast is the interface of exchange between the maternal and fetal circulations, it may act as a barrier through a large number of ATP (adenosine triphosphate)-binding cassette (ABC) drug transporters such as P-glycoprotein, multidrug resistance-associated proteins (MRPs) and breast cancer resistance protein (BCRP) (12,13).
Although the conceptus is implanted in the endometrium of the uterus by the end of the first week after conception, there is still no interface established for fetal–maternal exchange. The uteroplacental circulation begins to be
established by the second week after fertilization, but it is not until the fourth week that the essential arrangements necessary for the physiologic exchange between mother and the embryo are well established (14). From the fourth week of gestation, the syncytiotrophoblast layers become thinner. The surface area for exchange increases dramatically to meet the demands of the growing fetus. At term, the human placenta has only a single cell layer separating the fetal capillary endothelium and the maternal blood; hence it does not pose a hermetic barrier to transport.
established by the second week after fertilization, but it is not until the fourth week that the essential arrangements necessary for the physiologic exchange between mother and the embryo are well established (14). From the fourth week of gestation, the syncytiotrophoblast layers become thinner. The surface area for exchange increases dramatically to meet the demands of the growing fetus. At term, the human placenta has only a single cell layer separating the fetal capillary endothelium and the maternal blood; hence it does not pose a hermetic barrier to transport.
Pharmacokinetic Changes During Pregnancy
Pregnancy is a dynamic state with complex physiologic alterations that may affect the uptake, distribution, metabolism, and clearance of drug in the mother. During pregnancy the mother’s intestinal motility is decreased, the gastric emptying time is increased, and the extent of drug absorption may be reduced (16). As a result of increased maternal blood volume, body mass, and decreased plasma protein binding, the volume of distribution of most drugs increases during pregnancy. Albumin levels are reduced due to plasma dilution, but α-acid glycoprotein remains constant during pregnancy.
Drugs can be distributed into the amniotic fluid and may achieve there even higher values than those in the maternal and fetal plasma (17). In addition, the clearance rate of lipophobic and polar drugs is accelerated due to either an enhanced rate of hepatic metabolism or of renal elimination (increased glomerular filtration rate or increased renal blood flow). In contrast, elimination of lipophilic drugs may be decreased during pregnancy (18). All these changes in pharmacokinetics during pregnancy will affect the rate and extent of fetal drug exposure, and drug dosing during pregnancy should take into account all these alterations. Typically both peak and steady-state serum concentrations of drugs tend to be lower during pregnancy than in the nonpregnant state. For a small number of drugs (e.g., theophylline), there is evidence of a slower metabolic rate in the pregnant mother (19). Major pharmacokinetic changes during pregnancy and their potential clinical effects are summarized in Table 13.1. Compared to the nonpregnant state, there are relatively few specific pharmacokinetic data during pregnancy due to perceived ethical issues in research during pregnancy (20).
|
Mechanisms of Placental Drug Transfer
It is generally accepted that most chemical substances administered to the mother are able to permeate, to some degree, across the placenta (21). For some compounds, the placenta offers a protective barrier for the developing fetus by reducing the entry from the mother to the fetus, while for others it facilitates their passage both to and from the fetal compartment (22). Hence, the placenta may act in a similar manner to the kidney and liver in the elimination of different substances.
Transplacental exchanges may involve passive transfer, facilitated diffusion, active transport, phagocytosis, and pinocytosis. Passive transfer is the main form of exchange through the placenta. However, passive diffusion alone is not adequate to fulfill the fetal requirements for nutrients, and these are achieved through specific transport proteins for several nutrients, including the transport of amino acids, fatty acids, and glucose. Facilitated diffusion is only a minor role in the transfer of some drugs. It is likely that phagocytosis and pinocytosis are too slow to be important in the transfer of drugs, and therefore they have received little attention in the scientific literature.
Under some circumstances, the comparison of drug concentration in maternal and fetal plasma may give some idea of the exposure of the fetus to drugs that have been administered to the mother. Some drugs rapidly cross the placenta, and their concentrations in fetal and maternal plasma are similar to maternal levels, whereas other drugs cross incompletely and their fetal concentrations are lower than those in the maternal circulation. Many of the drugs fit into these two models. A limited number of drugs reach greater concentrations in fetal than in maternal plasma
(23). Ceftizoxime may serve as an example as the only antibiotic known so far with concentrations that are higher in the cord than in maternal plasma (24). This type of transfer is called “exceeding” transfer.
(23). Ceftizoxime may serve as an example as the only antibiotic known so far with concentrations that are higher in the cord than in maternal plasma (24). This type of transfer is called “exceeding” transfer.
Simple Diffusion
Many of the pharmacologically active compounds cross the human placenta by simple diffusion, which means that the crossing of molecules is a passive process occurring without the use of energy, and is described by Fick’s equation:

where K is the diffusion constant of the investigational drug, A is the surface area available for transfer, Cm is the maternal concentration of the drug, Cf is the fetal concentration of the drug, and X is the thickness of the membrane. Transfer in this case is proportional to the concentration gradient between maternal and fetal plasma. The rate of transfer is also affected by the surface area, the thickness of the membrane, the physicochemical characteristics of the drugs, and placental factors (25). Generally, compounds that have low molecular weight, are lipid soluble, unionized, and unbound to proteins, are able to readily cross the placenta (22). Physiologic substances such as water, urea, carbon dioxide, and hormones are mainly cleared from the fetus by passive diffusion (26). In addition to many pharmacologically active compounds that cross the placenta by simple diffusion, larger molecules such as antibodies may also be transported by this way (27).
Facilitated Diffusion
Facilitated diffusion is a mechanism of transplacental transfer that is carrier-mediated, but not dependent on energy. Transfer occurs down a concentration gradient. Compounds that are structurally related to endogenous substances are assumed to be transported by this mechanism, glucose being the most important example (28). Some drugs that do not necessarily mimic endogenous compounds use this mechanism of transport with the assistance of carriers; examples are cephalexin (28) and ganciclovir (29). Theoretically, facilitated diffusion would enable the drug to reach a higher peak of concentration in the fetus than would be expected from simple diffusion, and hence it may be important for fetal therapy.
Active Transport
Active transport is a carrier-mediated process that requires energy to transfer compounds against an electrochemical or concentration gradient (26). Active transporters are located either in the brush border (apical) membrane facing the maternal blood space or the basal membrane facing the fetal capillaries, where they transfer compounds in and out of the syncytiotrophoblast (22).
There have been more than 20 different transporter proteins identified in the human placenta (30,31). The identified physiological role of most of these transporters is to transport nutrients to the fetus and to eliminate metabolic waste products from the fetus; however many of them are able to interact with pharmacological agents (11). Exogenous compounds that interact with placental transporters are often structurally similar to endogenous substrates. Therefore, if such compounds are present in the maternal blood, placental transporters may facilitate their transfer from the mother to the fetus. In other words, these influx transporters may increase the fetal exposure to such drugs. Similarly, there are placental transporters that mediate the efflux of endogenous substrates from the fetus to the mother and these transporters may protect the fetus from drug exposure. Over the last decade, increasing efforts have been devoted to investigation of the ABC (ATP–binding cassette) efflux transporters in the placenta, including P-glycoprotein, multidrug resistance-associated proteins (MRPs), and breast cancer resistance protein (BCRP). The generally accepted function of ABC transporters is that they act as a protective mechanism against a large variety of xenobiotics, as well as protecting tissues from adverse effects of endogenous compounds (32,33).
P-Glycoprotein (MDR1)
P-glycoprotein, the multidrug-resistant gene (MDR1) product, is the first discovered and so far best characterized of drug efflux transporters. P-glycoprotein is able to actively pump drugs and other xenobiotics from trophoblast cells back to the maternal circulation, thus decreasing fetal exposure (32,31). P-glycoprotein has been detected in human placental trophoblasts from the first trimester to term, however its expression decreases as gestation advances (34,35). It is conceivable that higher P-glycoprotein expression in early pregnancy protect the fetus from xenobiotic toxicity at the sensitive period of embryogenesis. Because of this, the fetus will typically be able to tolerate maternal concentrations of drugs that have a high affinity with P-glycoprotein than drugs that have poor affinity (36).
P-glycoprotein is able to transport an extremely wide variety of chemically and structurally diverse compounds. Recent studies suggest that P-glycoprotein at the apical maternal–fetal interface may provide the mechanism to protect the developing fetus from xenobiotics that are substrates for this efflux pump, including digoxin, azytromycin, anticancer drugs, human immunodeficiency virus (HIV) protease inhibitors, opioids, and antiemetics (32). Inhibition of placental P-glycoprotein (e.g., verapamil, cyclosporine) may therefore increase the fetal exposure to these compounds (37). In addition to specific inhibitors, some herbal drugs including St. John’s wort are capable of affecting P-glycoprotein function, and thus may affect the placental transfer of other compounds (32,38). Important elements to consider when studying P-glycoprotein are glucocorticosteroids, which are generally regarded as P-glycoprotein substrates. Young et al. (39) reported that steroid hormones directly influence the level of expression of some of these transporters, and therefore the investigation of this link may be key in developing a strategy for drug delivery to the mother with minimal fetal exposure (39). On the other hand, in some cases, use of P-glycoprotein inhibitors may be theoretically effective in enhancing drug availability to the fetus, while minimizing drug exposure to the mother (32). This new knowledge may lead to methods for increasing drug concentration in the fetal compartment, which is
necessary for fetal therapy (e.g., digoxin for fetal tachyarrhythmia) (40). As example, one may consider the treatment of fetal tachyarrhythmia with both digoxin and verapamil. Verapamil has the potential to enhance digoxin transfer to the fetus by inhibiting placental drug efflux through P-glycoprotein (22). However, this must be carefully monitored to ensure that digoxin toxicity to the fetus does not occur. In addition, concerns about possible drug-drug and drug-endogenous substances interactions when they compete for the same transporter have been expressed (22). Thus, caution should be taken when a substrate and an inhibitor of P-glycoprotein are concomitantly administered to pregnant women.
necessary for fetal therapy (e.g., digoxin for fetal tachyarrhythmia) (40). As example, one may consider the treatment of fetal tachyarrhythmia with both digoxin and verapamil. Verapamil has the potential to enhance digoxin transfer to the fetus by inhibiting placental drug efflux through P-glycoprotein (22). However, this must be carefully monitored to ensure that digoxin toxicity to the fetus does not occur. In addition, concerns about possible drug-drug and drug-endogenous substances interactions when they compete for the same transporter have been expressed (22). Thus, caution should be taken when a substrate and an inhibitor of P-glycoprotein are concomitantly administered to pregnant women.
Nevertheless, it is conceivable that assessment of P-glycoprotein activity in the placenta may improve drug choice in pregnancy. For instance, drugs that are actively transported by P-glycoprotein may be preferred to limit fetal drug exposure. In contrast, efflux of HIV protease inhibitors by P-glycoprotein may decrease fetal defense against the virus (36). To increase drug concentrations in the fetal compartment, future use of appropriate P-glycoprotein inhibitors may be advantageous since they would increase drug availability to the fetus and improve therapy outcomes.
Multidrug Resistance-Associated Proteins and Breast Cancer Resistance Protein
Other major drug efflux transporters identified in the placenta are the multidrug resistance-associated proteins (MRPs) and breast cancer resistance protein (BCRP).
The family of MRP transporters currently comprises nine members (MRP1–9) (32). These transporters are involved in the transport of numerous clinically important anionic drugs as well as their glucuronide and glutathione metabolites. Drugs transported by MRPs are the anticancer agents methotrexate, etoposide, vincristine, vinblastine and cisplatin, HIV protease inhibitors, acetaminophen glucuronide, and the antibacterials grepafloxacin and ampicillin (22). Physiologically, MRPs mediate the removal of bilirubin glucuronides from the placenta (11).
The BCRP is highly expressed in the placenta and has a similar role and substrates to P-glycoprotein. It is involved in the efflux of drugs away from the fetus, acting as a protective mechanism for the fetus (39). Functional studies in the last decade have documented that BCRP can transport a large number of drugs from various therapeutic categories including anticancer drugs, antibiotics, antihypertensive, and antidiabetics (41). Of particular interest is that various drugs commonly administered to pregnant women, including nitrofurantoin (42) and glyburide (43) are BCRP substrates. It has been demonstrated that BCRP-mediated placental transport of glyburide is at least one of the mechanisms by which fetal exposure of the drug is limited (41,43). Physiologically, the BCRP may function in the homeostasis of porphyrins (11). Recent studies have suggested that BCRP may also regulate placental estrogen synthesis through modulating intracellular concentrations of estrogen precursors, dehydroepiandrosterone sulfate (DHEAS), and estrone-3-sulfate (E3S) (41,44).
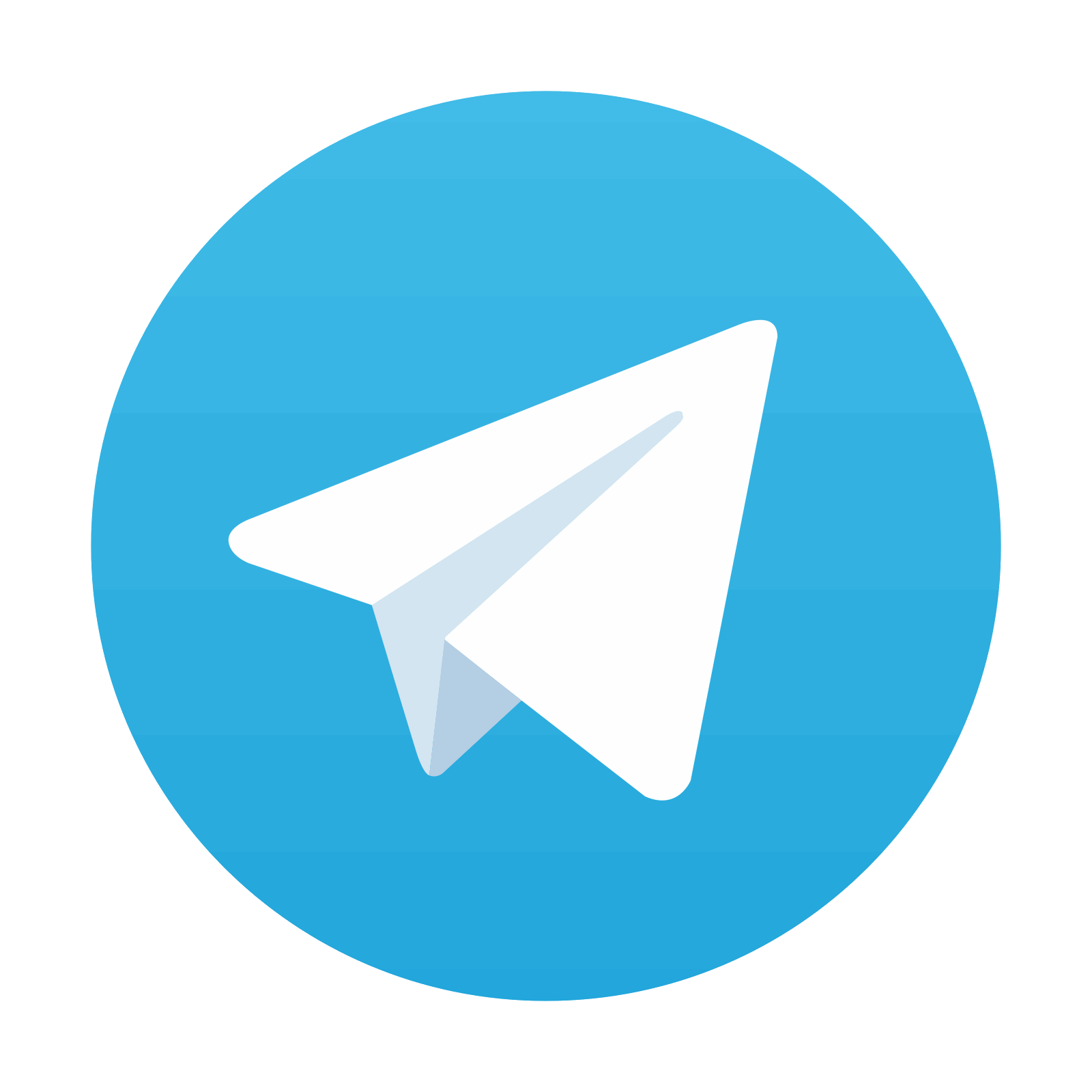
Stay updated, free articles. Join our Telegram channel

Full access? Get Clinical Tree
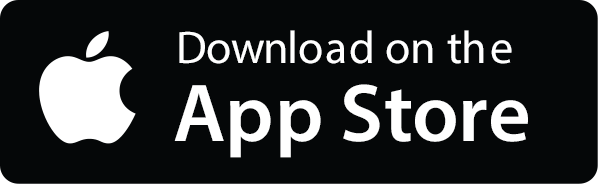
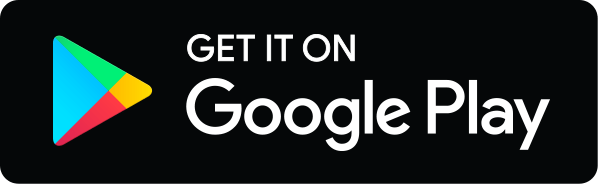
