The management of critically ill pediatric patients with trauma poses many challenges because of the infrequency and diversity of severe injuries and a paucity of high-level evidence to guide care for these uncommon events. This article discusses recent recommendations for early resuscitation and blood component therapy for hypovolemic pediatric patients with trauma. It also highlights the specific types of injuries that lead to severe injury in children and presents challenges related to their management.
Key points
- •
The use of component therapy during massive transfusion can help reverse the effects of coagulopathy during trauma resuscitation.
- •
Isolated solid organ injury in hemodynamically stable patients can be observed outside of an intensive care setting.
- •
Pulmonary contusions are more common than rib fractures after pediatric injury and can increase morbidity during recovery from other injuries.
- •
In the absence of a contraindication, aspirin should be used in the management of grade I blunt cerebrovascular injury.
- •
Postpubertal and severely injured adolescent traumas should be considered for thromboembolism prophylaxis.
Introduction
The management of pediatric patients with trauma poses many unique challenges. Severe injuries in pediatric trauma are rare, making it difficult to obtain sufficient data to develop evidence-based treatment algorithms for many injuries. This lack of data results in the need to rely on either guidelines developed for adults that may not be directly applicable to children or to rely only on clinical judgment. This article provides an overview of the treatment of critically injured children and highlights several areas of management that require an integrated approach between trauma surgeons and critical care physicians.
Introduction
The management of pediatric patients with trauma poses many unique challenges. Severe injuries in pediatric trauma are rare, making it difficult to obtain sufficient data to develop evidence-based treatment algorithms for many injuries. This lack of data results in the need to rely on either guidelines developed for adults that may not be directly applicable to children or to rely only on clinical judgment. This article provides an overview of the treatment of critically injured children and highlights several areas of management that require an integrated approach between trauma surgeons and critical care physicians.
Overview
Data obtained from the National Trauma Databank in 2014 show that 42% of injured children (n = 29,725) seen at trauma centers are admitted to an intensive care unit during their hospitalizations ( Fig. 1 ). The average age of injured children admitted to an intensive care unit is 8 years old and these children spend 5 days on average in the intensive care unit. Most children who are admitted to an intensive care unit have sustained blunt trauma (91%). Children with either a head or neck injury represent the most common injuries requiring admission to the intensive care unit (57%). Among children with a head or neck injury, most had injuries classified as at least moderate ( Fig. 2 ). Excluding patients who went to the operating room and those who died in the emergency department, only 19% of patients (172 out of 908) who died during their hospitalizations were treated in the intensive care unit. More than 50% of injured children in intensive care units have an injury severity score (ISS) greater than 15, showing the complexity of these patients ( Fig. 3 ). Injured children admitted to an intensive care unit at any time during their hospitalizations have as much as a 3-fold greater risk of hospital readmission than do injured children who do not require intensive monitoring. Although care should be improved in all settings, several studies have suggested that critically injured children have lower mortality and lower hospital length of stay when treated in a pediatric hospital compared with treatment in an adult hospital. The better outcomes in these patients support the selective triage of seriously injured children to trauma centers with pediatric intensive care units when this is an available option.
Fluid resuscitation
Fluid resuscitation is a critical component of the early management of many injured children. Over-resuscitation and under-resuscitation can each have adverse effects. Inadequate resuscitation can worsen the shock state, whereas the administration of excess fluid can trigger a cascade of adverse events, including oxygen desaturation, prolongation of intubation, and edema. For hemodynamically unstable children, standard recommendations for initial resuscitation are to administer a crystalloid fluid bolus of 20 mL/kg, to repeat this bolus if needed, and to then begin transfusion of packed red blood cells (PRBCs) with an initial bolus volume of 10 mL/kg. An assessment of the patient’s signs and symptoms should direct fluid resuscitation, which includes the patient’s vital signs, the patient’s mental status, and a physical examination assessing peripheral perfusion. Although most children are resuscitated initially with crystalloid, initial resuscitation with blood components is preferred in some settings. Blood transfusion is preferred to crystalloid administration in the setting of blood loss after injury when the initial hemoglobin level is less than 7 g/dL. Although this scenario is typically not seen in the emergency department, it may be observed after admission to an inpatient setting. Because the initial administration of crystalloid may lead to hemodilution and new or worsened symptoms related to blood loss, blood products should be given even in the absence of signs or symptoms of anemia.
Controlled resuscitation, also known as permissive hypotension, has been increasingly adopted as a strategy for fluid management among adult patients with hemorrhagic shock. Controlled resuscitation is a nonaggressive approach to fluid administration in patients who are volume depleted because of hemorrhage. When using this strategy, normal vital sign values are not the main target of therapy. The goal of controlled resuscitation instead is to stabilize the patient’s hemodynamic status but at a lower blood pressure than normal. Several explanations have been proposed to account for the potential benefits of permissive hypotension, which include the minimization of hydrostatic pressure for stabilizing clot and reduction of the dilutional effects of coagulopathy. Animal studies have shown that fluid resuscitation reduces mortality when used for treatment of large-volume blood loss and increases mortality when used for less severe hemorrhage compared with no fluid resuscitation. These findings suggest that the benefits of fluid resuscitation do not always justify its risks and that the approach to fluid resuscitation should be tailored to the clinical setting. For example, hemodynamically stable patients who have bled from a solid organ injury without evidence of ongoing bleeding should be treated with controlled resuscitation rather than with aggressive fluid resuscitation. Findings from animal studies also show that the use of controlled resuscitation decreases mortality for injuries associated with hemorrhage, regardless of blood volume lost. Several reasons may account for the decreased mortality associated with controlled resuscitation, including fewer complications related to fluid overload (pulmonary congestion, edema, electrolyte derangements) and decreased dilutional coagulopathy.
Because of differences between adult and pediatric physiology, extrapolating findings from adult studies to make recommendations for treating injured children with hemorrhagic shock may not be appropriate. Compared with adults, children can maintain a normal blood pressure even in the setting of significant blood loss and ongoing hemorrhage. Hypotension may also not develop in children until the proportion of lost blood volume is as high as 45%, a volume requiring intervention in most patients. Despite evidence of the benefits of permissive hypotension in adults and data obtained from animal studies, its role in managing hemorrhagic shock after pediatric injury is uncertain. Limitation of fluid resuscitation may not be optimal because of the child’s delayed presentation of hypotension in hemorrhagic shock, decreasing the value of blood pressure as an end point for controlled resuscitation in children. The applicability of controlled resuscitation in children may then depend on approaches that can identify the shock state sooner than standard vital sign measurements and other physical examination adjuncts.
Successful resuscitation requires coordination of prehospital and initial hospital care. When provided with details of the injury, the hospital team can anticipate future blood loss when given initial estimates of the blood volume already lost. Early notification of the patient’s volume status can increase the timeliness of treatment in the trauma bay and intensive care unit, just as early notification by emergency medical services improves treatment of other medical conditions. With early knowledge of the patient’s volume status, initial hospital management can quickly begin, before patient arrival if needed, including the release of blood products, setting up central venous or arterial lines, or preparing the operating room. Because initiating these interventions can be time consuming, early preparation for lifesaving treatments allows for more rapid treatment and can have a major effect on patients for whom minutes and seconds matter.
Acid-base status based on arterial blood gas measurement can be useful for managing critically injured pediatric patients with trauma. Injured children who have undergone an arterial blood gas test can be classified as having a mild base deficit (greater than −5) or severe base deficit (less than or equal to −5). A severe base deficit is associated with a high risk of mortality (35%), whereas a mild base deficit is associated with survival. Among survivors of pediatric injury, most (75%) have their base deficits corrected within 48 hours. These results highlight the importance of early resuscitation and correction of acidosis on survival after pediatric injury. An admission base deficit also correlates with mortality. A higher mortality is observed in children with an admission base deficit less than −8. Injured children with an admission base deficit between −4 and −8 have a 12% mortality compared with those with base deficits between −8 and −12, who have a mortality of 32%. Injured children found to have a severe base deficit require rapid identification of the source of acidosis (eg, hemorrhage, intra-abdominal sepsis related to intestinal injury, or decreased respiratory efforts related to chest injury) and prompt intervention to reverse its effects.
Lethal triad is a term used to describe the cycle of acidosis, coagulopathy, and hypothermia that can occur during hemorrhagic shock. Each factor negatively affects the others, amplifying the deleterious effects of each. Even in the absence of significant blood loss, injured patients have an increased risk of bleeding if they have a severe injury. This bleeding diathesis is known as acute coagulopathy of trauma. It may not be related to the dilution of clotting factors associated with crystalloid fluid administration but may be the result of tissue injury and subsequent triggering of inflammatory changes. Estimates of the prevalence of acute coagulopathy of trauma vary in injured children, but it may exceed 25%. Children with blood loss large enough to require blood transfusion need monitoring of their coagulation profile early on during their admission to correct their coagulopathy.
Damage control laparotomy is a strategy used when patients with hemoperitoneum secondary to trauma, cannot undergo extensive surgical procedures because of evidence of the lethal triad. The major goals of damage control laparotomy are to control active hemorrhage from major vascular injuries as quickly as possible and to limit abdominal contamination, rather than proceeding with more time-consuming definitive surgical treatments. Active bleeding from a solid organ or compressible vessel may be controlled by tightly packing the affected abdominal region with gauze. To control intra-abdominal contamination, injured bowel is stapled off at the site of injury rather than repaired using a more time-consuming intestinal anastomosis. The abdomen is temporarily covered with an occlusive dressing and return to the operating room is planned within the next 48 hours. By reducing or stopping ongoing hemorrhage and intra-abdominal contamination, the lethal triad can be corrected or avoided better than with definitive surgery. The added time in the operating room for definitive surgical treatment can lead to continued metabolic stress, further heat loss, and worsened coagulopathy. Critically injured patients with the lethal triad treated with damage control laparotomy have a lower mortality than those undergoing definitive surgery, supporting the conceptual benefits of this approach.
The continued use of crystalloid and PRBCs without the use of other blood products in patients with the lethal triad may worsen hypothermia and coagulopathy. This understanding has led to the development of component therapy. Component therapy is the use of other blood products in addition to PRBCs (eg, fresh frozen plasma [FFP], platelets, and cryoprecipitate) during initial resuscitation. The use of all blood components is essential for the treatment of patients receiving massive transfusion to counter the effects of dilutional coagulopathy. With the administration of only PRBCs, dilution of platelets and clotting factors may occur and inhibit the contribution of each in clot formation and hemorrhage control. The goal of component therapy is to return the clotting cascade to its normal balance by replenishing all the components necessary for coagulation rather than providing only fluid replacement, which improves blood pressure and oxygen delivery.
Massive blood transfusion protocols (MBTPs) are now used at most pediatric and adult trauma centers in the United States. Although clinical judgment usually directs the activation of these protocols, several guidelines can be used to determine the need to activate the protocol, including the loss of 1 blood volume within 24 hours or the loss of half of a blood volume within 2 hours. An estimate of blood volume can be made from previously published data based on age and weight ( Table 1 ). Most centers with a massive transfusion protocol have adopted a 1:1:1 of PRBC/FFP/platelet administration. However, the ratio used at different centers varies, and the most appropriate ratio for transfusion of products in children remains controversial. Several guidelines have been established at pediatric and adult hospitals that can guide the initial implementation of component replacement ( Fig. 4 , Table 2 ).
Age | Estimated Blood Volume (mL/kg) |
---|---|
Premature infant | 90–100 |
Term infant – 3 months | 80–90 |
Children older than 3 months | 70 |
Very obese children | 65 |
Order of Packages to Transfuse a | Neonate 0–4 kg | Infant 5–9 kg | Young Child 10–24 kg | Older Child 25–49 kg | Teen/Adult ≥50 kg |
---|---|---|---|---|---|
Emergency release (A) | 0.5 RBC | 1 RBC | 2 RBC | 3 RBC | 5 RBC |
B | 0.5 RBC | 1 RBC | 2 RBC | 3 RBC | 5 RBC |
0.5 FFP | 1 FFP | 2 FFP | 3 FFP | 5 FFP | |
2 eu Plt | 3 eu Plt | 4 eu Plt | 6 eu Plt | 6 eu Plt | |
C | 0.5 RBC | 1 RBC | 2 RBC | 3 RBC | 5 RBC |
0.5 FFP | 1 FFP | 2 FFP | 3 FFP | 5 FFP | |
2 Cryo | 3 Cryo | 4 Cryo | 6 Cryo | 8 Cryo | |
B | 0.5 RBC | 1 RBC | 2 RBC | 3 RBC | 5 RBC |
0.5 FFP | 1 FFP | 2 FFP | 3 FFP | 5 FFP | |
2 eu Plt | 3 eu Plt | 4 eu Plt | 6 eu Plt | 6 eu Plt | |
C | 0.5 RBC | 1 RBC | 2 RBC | 3 RBC | 5 RBC |
0.5 FFP | 1 FFP | 2 FFP | 3 FFP | 5 FFP | |
2 Cryo | 3 Cryo | 4 Cryo | 6 eu Plt | 8 Cryo | |
B | 0.5 RBC | 1 RBC | 2 RBC | 3 RBC | 5 RBC |
0.5 FFP | 1 FFP | 2 FFP | 3 FFP | 5 FFP | |
2 eu Plt | 3 eu Plt | 4 eu Plt | 6 eu Plt | 6 eu Plt | |
C | 0.5 RBC | 1 RBC | 2 RBC | 3 RBC | 5 RBC |
0.5 FFP | 1 FFP | 2 FFP | 3 FFP | 5 FFP | |
2 Cryo | 3 Cryo | 4 Cryo | 6 eu Plt | 8 Cryo |
a Letters denote package type to be released correlating with weight-associated package contents.
Reports from emergency medical services may indicate that several rounds of blood product administration may be necessary to stabilize a patient in the emergency department. Activation of an MBTP can then be based on prehospital reports or reports from referral hospitals. Some injured children do not require activation of the MBTP initially but may then need this additional access to blood products after reaching an inpatient unit. This delay in needed transfusion may occur because of a delayed diagnosis of injury. In this scenario, activation of the MBTP should be viewed as a bridge to more definitive therapy, such as surgery or treatment in the interventional radiology suite. It is preferred to have a low threshold when deciding whether to activate the MBTP. Returning unused items to the blood bank is preferable to the potential consequences of delayed administration of blood products.
Solid organ injury
Solid organ injury after blunt trauma includes injuries to the liver, spleen, and kidney. Solid organ injuries can be suspected based on a history of blunt torso trauma and signs and symptoms of injury. Intra-abdominal injury requiring intervention can be excluded (likelihood of injury <0.1%) based on the initial clinical presentation: the absence of abdominal pain, the absence of tenderness or external evidence of abdominal wall trauma (seat belt sign), no external evidence of chest wall injury, the absence of vomiting, and the absence of significant head injury. Except for hemodynamically unstable patients who require immediate laparotomy, solid organ injuries are usually identified by computed tomography in at-risk patients. Although focused assessment with sonography for trauma (FAST) has seen wide adoption in trauma centers worldwide, its low sensitivity and specificity for diagnosing abdominal injuries in children may limit its utility as a screening test in children. Grading scales are available based on computed tomography findings to standardize reporting of the severity of solid organ injuries ( Table 3 ). As recently as 50 years ago, it was common belief that splenic injuries were almost always fatal because of the potential for continued bleeding unless a splenectomy was performed, and that splenorrhaphy was not safe, even for minor injuries. Studies in the 1960s and 1970s showed that nonoperative management of splenic injuries was a safe option after blunt trauma. In the presence of hemodynamic stability, nonoperative management has also been shown to be appropriate for managing blunt splenic injury, even when active bleeding is identified by contrast extravasation. Angioembolization may be a lower risk alternative to surgery for solid organ injury but has yet to be established as an effective strategy in hemodynamically stable patients, even in the presence of contrast extravasation. Although nonoperative management of blunt splenic injuries has been standard practice for more than a few decades, this approach continues to be adopted, as suggested by a decline in the percentage of children undergoing splenectomy after blunt trauma (11% to 3%) over the last 2 decades.

Full access? Get Clinical Tree
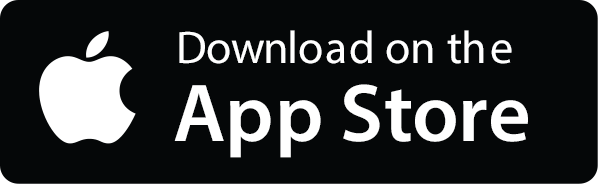
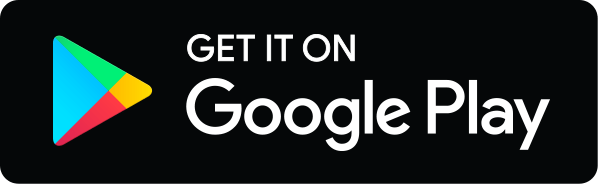