Macrolides, Chloramphenicol, and Tetracyclines
Basim I. Asmar
Nahed M. Abdel-Haq
Macrolides
Erythromycin
Erythromycin is the prototype of the macrolides. It has been one of the main antibiotics used in the treatment of pediatric infections since 1952. Erythromycin is a broad-spectrum antibiotic that has been primarily used to treat respiratory and skin and soft tissue infections (SSTIs) in children who are allergic to penicillin as well as infections due to penicillin-resistant organisms. Erythromycin is derived from Streptomyces erythreus, originally obtained from a soil sample in the Philippines (1).
Chemical Structure
Erythromycin consists of a 14-membered macrocyclic lactone ring with two appended deoxy sugar moieties, deconamine and cladinose, at positions 5 and 3 of the ring. Erythromycin base is poorly soluble in water and easily degraded by gastric acid. Because it is a large molecule, erythromycin diffuses through membranes slowly. This results in unpredictable erythromycin levels in serum and tissue following oral administration (Fig. 32.1) (2).
Mechanism of Action/Mechanism of Antibacterial Resistance
Erythromycin and other macrolides inhibit bacterial pathogens by binding to the peptidyltransferase region of the 50S subunit of bacterial 70S ribosomes causing inhibition of bacterial protein synthesis. Macrolides are bacteriostatic. Erythromycin and other macrolides appear to inhibit the translocation step during bacterial protein synthesis rather than peptide bond formation. The translocation step involves movement of the peptidyl tRNA molecule from the acceptor site of the ribosome to the donor site (3,4).
Acquired bacterial resistance to macrolides involves one of three mechanisms: (a) alteration of the ribosomal binding site, (b) decreased accumulation of the drug due to an active efflux pump, or (c) macrolide inactivation by esterases or by phosphorylation or glycosylation at the 2′-position (5). Modification of the ribosomal binding site by methylation is the most common clinically significant resistance mechanism in gram-positive bacteria. Methylase enzyme production may be inducible or constitutive and is mediated by erm genes (ermA, ermB, ermC). The MLSB phenotype is conferred by erm genes, indicating resistance to macrolides, lincosamides, and type B streptogramins, which share the same ribosomal binding site. MLSB resistance is due to modification of the 23S rRNA binding site at adenine 2058 by methylation (6,7). Resistance to the 14- or 15-membered macrolides may be the result of efflux pumps encoded by genes such as mefE in Streptococcus pneumoniae, mrsA in Staphylococcus aureus, and mefA in group A beta hemolytic Streptococcus (GABHS) (8,9,10). Chromosomal mutation involving the 50S ribosomal protein is another mechanism of resistance to macrolides in gram-positive cocci (6).
In Vitro Efficacy
In vitro activity of erythromycin and other macrolides has been shown to be greater in alkaline media possibly due to increased penetration of the bacterial cell wall. The spectrum of activity of erythromycin includes gram-positive cocci such as S. pneumoniae, Streptococcus pyogenes, and S. aureus as well as atypical and intracellular pathogens such as Chlamydia pneumoniae, Chlamydia trachomatis, Mycoplasma pneumoniae, and Ureaplasma urealyticum (11,12). Erythromycin is active in vitro against gram-positive bacilli such as Bacillus anthracis, Clostridium species, and Corynebacterium species. Erythromycin also has excellent activity against Legionella pneumophila, Bordetella pertussis, and Campylobacter jejuni. Less activity has been shown against Eikenella, Haemophilus influenzae, Pasteurella, and Brucella. Most Treponema species, Rickettsia rickettsiae, and to a lesser extent Borrelia burgdorferi and Helicobacter pylori are inhibited by erythromycin (13,14).
Clinical Indications
Erythromycin is considered the drug of choice for a number of pediatric infections. It is also considered an alternative to penicillin in patients with penicillin allergy. Erythromycin
is active against B. pertussis. In addition to eradicating nasopharyngeal carriage and preventing infection with B. pertussis, erythromycin may shorten the clinical course of illness if started during the catarrhal or early paroxysmal phase of pertussis. Erythromycin is indicated in the early treatment of pertussis as well as prophylaxis of close contacts (15). Following identification of M. pneumoniae as a frequent cause of pneumonia in school-age children, adolescents, and young adults, erythromycin became an important component of antibiotic treatment of pneumonia among these patients. Erythromycin has been shown to shorten the duration of clinical illness and time to radiologic clearance in patients with Mycoplasma pneumonia (16). In the 1970s, C. trachomatis was identified as an important cause of pneumonia in neonates and young infants. C. trachomatis causes pneumonia in infants 3 weeks to 4 months of age and ophthalmia neonatorum in infants younger than 30 days. Treatment with erythromycin has been shown to eradicate nasopharyngeal carriage of the organism and hasten recovery of conjunctivitis and pneumonia (17). The recommended treatment for both infections is erythromycin 50 mg per kg per day in four divided doses for 14 days. In addition, nongonococcal urethritis caused by C. trachomatis usually responds to a 7-day course of oral erythromycin, although doxycycline is probably more effective. The newer agents, azithromycin and ofloxacin, are also effective (18). Erythromycin is an alternative to tetracycline for treatment of C. trachomatis infection during pregnancy (19). For nongonococcal urethritis caused by U. urealyticum, doxycycline is the drug of choice, but erythromycin is also effective. This organism can also cause bacteremia, pneumonia, or meningitis in the neonate and should be treated with intravenous erythromycin (20). Erythromycin has also been found to be effective in the treatment of pneumonia caused by Chlamydophila pneumoniae, L. pneumophilia, and Legionella micdadei as well as in the treatment of Legionnaires disease (21,22). Erythromycin remains the drug of choice for Legionella pneumonia. Mild infections may be treated by oral erythromycin, whereas moderate and severe infections should be treated by intravenous erythromycin. Erythromycin in combination with rifampin should be used for very ill patients and for those not responding to erythromycin (23). Erythromycin is considered the drug of choice for treating diarrhea caused by C. jejuni when clinically indicated (24). Although the effect of erythromycin on the course of illness is controversial, erythromycin may be given to patients who have fever, bloody stools, or symptoms persisting for longer than 1 week.
is active against B. pertussis. In addition to eradicating nasopharyngeal carriage and preventing infection with B. pertussis, erythromycin may shorten the clinical course of illness if started during the catarrhal or early paroxysmal phase of pertussis. Erythromycin is indicated in the early treatment of pertussis as well as prophylaxis of close contacts (15). Following identification of M. pneumoniae as a frequent cause of pneumonia in school-age children, adolescents, and young adults, erythromycin became an important component of antibiotic treatment of pneumonia among these patients. Erythromycin has been shown to shorten the duration of clinical illness and time to radiologic clearance in patients with Mycoplasma pneumonia (16). In the 1970s, C. trachomatis was identified as an important cause of pneumonia in neonates and young infants. C. trachomatis causes pneumonia in infants 3 weeks to 4 months of age and ophthalmia neonatorum in infants younger than 30 days. Treatment with erythromycin has been shown to eradicate nasopharyngeal carriage of the organism and hasten recovery of conjunctivitis and pneumonia (17). The recommended treatment for both infections is erythromycin 50 mg per kg per day in four divided doses for 14 days. In addition, nongonococcal urethritis caused by C. trachomatis usually responds to a 7-day course of oral erythromycin, although doxycycline is probably more effective. The newer agents, azithromycin and ofloxacin, are also effective (18). Erythromycin is an alternative to tetracycline for treatment of C. trachomatis infection during pregnancy (19). For nongonococcal urethritis caused by U. urealyticum, doxycycline is the drug of choice, but erythromycin is also effective. This organism can also cause bacteremia, pneumonia, or meningitis in the neonate and should be treated with intravenous erythromycin (20). Erythromycin has also been found to be effective in the treatment of pneumonia caused by Chlamydophila pneumoniae, L. pneumophilia, and Legionella micdadei as well as in the treatment of Legionnaires disease (21,22). Erythromycin remains the drug of choice for Legionella pneumonia. Mild infections may be treated by oral erythromycin, whereas moderate and severe infections should be treated by intravenous erythromycin. Erythromycin in combination with rifampin should be used for very ill patients and for those not responding to erythromycin (23). Erythromycin is considered the drug of choice for treating diarrhea caused by C. jejuni when clinically indicated (24). Although the effect of erythromycin on the course of illness is controversial, erythromycin may be given to patients who have fever, bloody stools, or symptoms persisting for longer than 1 week.
Erythromycin is an alternative to penicillin for the treatment of GABHS pharyngitis in patients who are allergic to penicillin. However, increased rates of infection with macrolide-resistant GABHS have been reported in areas of the world where macrolides are excessively used (such as Finland and Japan) resulting in treatment failures (25). Erythromycin is an effective alternative to penicillin G for treatment of pneumococcal pneumonia. It is also an alternative antibiotic for treatment of other pneumococcal infections outside the central nervous system (CNS) that are caused by susceptible strains. Similarly, erythromycin may be used as an alternative treatment for infections caused by susceptible strains of B. anthracis and Eikenella corrodens. Erythromycin in combination with sulfa drugs is effective against H. influenzae and Moraxella catarrhalis (26).
Although erythromycin may show in vitro susceptibility against Treponema, it is inferior to penicillin for treatment of syphilis. Erythromycin is not indicated in neurosyphilis and is no longer considered acceptable alternative therapy for penicillin-allergic pregnant women (27). It usually results in cure of the mother, but because placental transfer of the drug is inconsistent, the fetus may remain infected.
Erythromycin is considered an alternative treatment to amoxicillin for early-localized Lyme disease in children younger than 8 years who are allergic to penicillin (28).
For endocarditis chemoprophylaxis, oral erythromycin given 2 hours before the dental procedure and 6 hours later is recommended for standard-risk penicillin-allergic patients. Erythromycin is a suitable alternative to penicillin for prophylaxis against rheumatic fever.
Pharmacokinetic Properties
Degradation of erythromycin base by gastric acid results in reduced oral bioavailability. Food increases gastrointestinal acidity and may delay absorption. Thus, erythromycin base is administered as enteric-coated tablets or as capsules containing enteric-coated pellets that dissolve in the duodenum. Esters of the erythromycin base (stearate, estolate, and ethylsuccinate) have been formulated to improve acid stability and facilitate absorption. Erythromycin stearate is less readily destroyed in the stomach and is dissociated in the duodenum liberating erythromycin, which is absorbed. Erythromycin estolate is also less susceptible to acid than is the base and hence is better absorbed. Its bioavailability is not affected by food. It is absorbed mainly as ester, and then hydrolyzed in serum to active erythromycin. Erythromycin ethylsuccinate is also well absorbed after oral administration. Food increases its bioavailability (29). After absorption, erythromycin ethylsuccinate is hydrolyzed into active erythromycin.
Satisfactory serum concentrations are achieved following intravenous administration of erythromycin. Peak concentrations attained 1 hour after intravenous infusion are usually 4- to 10-fold greater than those attained after oral erythromycin (30). After intravenous administration of erythromycin lactobionate to preterm neonates at either 25 or 40 mg per kg per day divided into four doses every
6 hours, peak serum levels were 3.05 to 3.69 μg per mL and 1.92 to 2.9 μg per mL for the 40 and 25 mg per kg per day groups, respectively (31). Erythromycin diffuses well into intracellular fluids and body tissues except the cerebrospinal fluid (CSF) and the CNS. Middle ear fluid concentration is about 50% of serum concentration. This concentration may be sufficient to inhibit the highly sensitive S. pyogenes and S. pneumoniae but may be too low for treatment of otitis media caused by H. influenzae (32). Tonsillar concentrations are adequate after oral administration (33). Children with chlamydial conjunctivitis treated with erythromycin have tear fluid concentration of erythromycin approximately equal to simultaneous serum concentration. Erythromycin is concentrated in human polymorphonuclear leukocytes (34). Significant intracellular penetration occurs resulting in activity against intracellular pathogens (35).
6 hours, peak serum levels were 3.05 to 3.69 μg per mL and 1.92 to 2.9 μg per mL for the 40 and 25 mg per kg per day groups, respectively (31). Erythromycin diffuses well into intracellular fluids and body tissues except the cerebrospinal fluid (CSF) and the CNS. Middle ear fluid concentration is about 50% of serum concentration. This concentration may be sufficient to inhibit the highly sensitive S. pyogenes and S. pneumoniae but may be too low for treatment of otitis media caused by H. influenzae (32). Tonsillar concentrations are adequate after oral administration (33). Children with chlamydial conjunctivitis treated with erythromycin have tear fluid concentration of erythromycin approximately equal to simultaneous serum concentration. Erythromycin is concentrated in human polymorphonuclear leukocytes (34). Significant intracellular penetration occurs resulting in activity against intracellular pathogens (35).
Studies of single 10 mg per kg oral doses of erythromycin estolate and ethylsuccinate in infants younger than 4 months with C. trachomatis infection have shown similar peak serum concentrations (Cmax) of erythromycin (36). Similar findings were noted after repeat dosing to steady state. Single-dose studies also showed similar time to Cmax (Tmax) for both formulations. However, multiple-dose studies in infants 3 to 48 months of age showed that Tmax was longer for the estolate formulation, resulting in larger area of activity under the curve (AUC) at steady state (36). In neonates, absorption of erythromycin appears to be delayed and bioavailability is not influenced by feeding (37).
The majority of macrolides including erythromycin are eliminated via hepatic metabolism and/or biliary excretion. Erythromycin is excreted as the active form in the bile. Macrolides are substrates of the hepatic enzyme cytochrome P450 3A4. Reduction in the activity of this enzyme secondary to disease or immaturity can result in elevated levels of macrolides. At therapeutic concentrations, 80% to 90% of erythromycin in the blood is protein bound. Erythromycin crosses the placenta, but fetal plasma drug concentrations are considerably lower and less predictable than those in the mother (38). Erythromycin is partly excreted in the urine: about 2.5% of orally administered dose and 15% of parenterally administered dose. A considerable proportion of erythromycin is excreted in the bile. In general, renal or hepatic dysfunction has little effect on the pharmacokinetics of macrolides including erythromycin, and dosage adjustment is not necessary in most occasions (39,40).
Dose/Regimens
The usual dosage of erythromycin base in children is 30 to 50 mg per kg per day administered in two to four equally divided doses. The maximum daily dose is 4 g. Erythromycin is available in base form, stearate, ethyl succinate, and estolate preparations. In severe infections, erythromycin may be administered by the intravenous route at a dosage of 15 to 50 mg per kg per day in four equally divided doses. The intravenous dose should be given by slow infusion over at least 1 hour to minimize the risk of cardiac arrhythmias. For newborns, oral erythromycin is administered at a dose of 10 mg per kg given every 12 hours for those younger than 1 week and every 8 hours for those 1 week of age and older.
Adverse Effects/Toxicity/Precautions
Erythromycin is the least-tolerated antibiotic among the macrolides. The most common adverse effects of erythromycin are gastrointestinal disturbances such as abdominal pain or discomfort and nausea (41,42). An association between very early exposure to erythromycin and infantile hypertrophic pyloric stenosis was demonstrated. Cooper et al. found that erythromycin use in neonates 3 to 13 days of life was associated with nearly eightfold increased risk of pyloric stenosis (43).
The gastrointestinal side effects are possibly related to the prokinetic action of erythromycin on the gut mediated by its motilin-receptor-stimulating activity (44). Reported allergic reactions include fever, rashes, joint pain, generalized pruritus, and Stevens–Johnson syndrome. Reversible hepatic dysfunction and liver enzyme abnormalities have been reported 10 to 14 days following erythromycin use. These include colicky abdominal pain, cholestasis, elevated transaminase levels, jaundice, and eosinophilia (41,42). Cholestatic hepatitis has been reported mainly with the estolate formulation and only rarely with the other derivatives. It may represent a hypersensitivity reaction to the estolate ester (42). Reversible neurosensory hearing loss affecting all hearing frequencies has been reported mostly in association with use of high doses in patients with impaired renal function (45). Thrombophlebitis and venous irritation have been reported with intravenous erythromycin infusions. The incidence and severity of these infusion reactions can be reduced by slowing of the rate of infusion and/or reducing the concentration of erythromycin in the infusate (42). Intravenous administration of erythromycin to infants and children has been associated with cardiotoxicity manifested by bradycardia, hypotension, torsades de pointes (polymorphous ventricular tachycardia), and cardiac arrest (30).
Drug Interactions
Macrolides interact with a variety of drugs by inhibiting the metabolism of the drugs via the cytochrome P450 (CYP) hepatic microsomal enzymes (46). Significant drug interactions occur with concomitant administration of erythromycin with antihistamines, cisapride, carbamazepine, valproate, theophylline, cyclosporine, digoxin, warfarin, and benzodiazepines (46,47,48,49,50). The interaction occurs via the induction of the CYP enzymes, which subsequently convert the macrolide to a nitrosoalkane metabolite forming an inactive complex with the iron of CYP.
Concomitant administration of erythromycin and theophylline can result in increased concentrations of theophylline. This is believed to be due to decreased clearance of theophylline by erythromycin. The reduction in clearance is variable (5% to 40%) and is related to type of erythromycin formulation, type of patient, and dose and duration of erythromycin therapy (40). Concomitant administration of erythromycin and cyclosporine results in increased concentrations of cyclosporine. The increase is in the range of 75% to 215% in cyclosporine AUC and is possibly due to decreased cyclosporine clearance, increased bioavailability, or both (49). The interaction with digoxin is also believed to be via increasing the bioavailability of digoxin
secondary to inhibition of the metabolizing bacteria, particularly Eubacterium lentum, in the large bowel. The interaction occurs in a small percentage of patients (10%) and is less likely to occur with the capsule preparations of digoxin (50).
secondary to inhibition of the metabolizing bacteria, particularly Eubacterium lentum, in the large bowel. The interaction occurs in a small percentage of patients (10%) and is less likely to occur with the capsule preparations of digoxin (50).
Azithromycin
Erythromycin has long been considered one of the main antibiotics for treating pediatric infections. Erythromycin has been characterized by a number of drawbacks, however, which resulted in the search for newer macrolides. Among these drawbacks are the narrow spectrum of activity, the poor oral tolerance, and the unfavorable pharmacokinetic properties. Azithromycin is a long-acting macrolide that has been synthesized to overcome the drawbacks of erythromycin.
Chemical Structure
Azithromycin is synthesized by incorporation of a 6-methyl-substituted nitrogen atom into position 9a of the 14-member macrolide ring (Fig. 32.2). This results in the expansion of the ring to 15 members, and accordingly azithromycin is classified as an azalide rather than a macrolide. The modification at the 9a position also results in a compound with more stability to degradation by gastric acid, improved oral absorption, and improved adverse effect profile (51).
Mechanism of Action/Mechanism of Antibacterial Resistance
Similar to other macrolide antibiotics, azithromycin inhibits protein synthesis by binding to the 50S ribosomal subunit, resulting in inhibition of peptide translocation. The mechanisms of resistance to azithromycin are similar to those of erythromycin and other macrolides.
In Vitro Efficacy
In general, azithromycin is more active than erythromycin against gram-negative organisms but provides no advantage over erythromycin in activity against gram-positive organisms (52). Many of the organisms that are resistant to erythromycin are also resistant to azithromycin (53). Improved in vitro activity compared with that of erythromycin has been shown against Haemophilus species, M. catarrhalis, Legionella species, Pasteurella multocida, B. burgdorferi, Mycoplasma species, Neisseria gonorrhea, C. trachomatis, and Campylobacter species. Azithromycin has appreciable activity against Mycobacterium avium complex (MAC) and Bartonella species (54,55). Azithromycin is active against S. pneumoniae, S. pyogenes, and methicillin-susceptible S. aureus (56). Macrolide resistance is common among staphylococci (S. aureus and coagulase-negative staphylococci). Resistance has also been increasingly reported among S. pneumoniae strains and S. pyogenes (57,58). Methicillin-resistant staphylococci are also resistant to erythromycin. Streptococci and staphylococci that are resistant to erythromycin are also resistant to azithromycin and clarithromycin. Penicillin-resistant S. pneumoniae strains are usually resistant to azithromycin. The prevalence of resistance to macrolides is related to geographical variation, antibiotic usage patterns, and subsequent antibiotic selection pressure. Azithromycin has similar in vitro activity to erythromycin against C. pneumoniae and B. pertussis (59,60). Azithromycin has been shown to exhibit postantibiotic effect against respiratory tract pathogens such as S. pneumoniae, H. influenzae, and S. pyogenes (61). This effect together with the host immune defenses can contribute to clinical efficacy of azithromycin at sites of infection when serum levels fall below the minimal inhibitory concentration (MIC) of the organism. Azithromycin is also active in vitro against Toxoplasma gondii.
Clinical Indications
Antibiotic treatment is indicated for treatment of acute GABHS pharyngitis to decrease the duration of symptoms and to prevent the spread to others and the subsequent development of rheumatic fever. Although oral penicillin V remains the drug of choice, a shorter course of oral azithromycin may result in better compliance. Treatment of GABHS pharyngitis using azithromycin 10 mg per kg on the first day followed by 5 mg per kg once daily for the next 4 days has not been shown to be effective. Two large double-blind, multicenter trials that compared azithromycin given at a dose of 12 mg per kg once a day for 5 days with penicillin V for 10 days for treatment of GABHS pharyngitis showed higher clinical responses and higher GABHS eradication rates in the azithromycin-treated groups (62). As a result, the Food and Drug Administration (FDA) approved the use of azithromycin at 12 mg per kg once daily for treatment of GABHS pharyngitis among children 2 years of age and older. Studies with shorter courses of azithromycin for 3 days produced variable results (63,64). This short course of azithromycin is not currently recommended. Azithromycin 12 mg per kg once daily for 5 days may be used as an alternative to penicillin V for treatment of GABHS pharyngitis in areas of low incidence of GABHS macrolide resistance.
Acute otitis media (AOM) is one of the most frequent infections in children. The most common bacterial pathogens are S. pneumoniae, non-typeable H. influenzae,
and M. catarrhalis. Choice of antibiotic treatment for AOM depends on the likelihood of bacterial resistance and on compliance-related factors such as frequency, palatability, and cost of the antibiotic. In clinical trials comparing azithromycin with other drugs and in which tympanocentesis was performed at baseline, rates of clinical efficacy were variable. In a study comparing azithromycin with amoxicillin–clavulanate, Dagan et al. (65) showed that azithromycin was less likely to eradicate all bacterial pathogens from middle ear fluid in 136 evaluable patients. Bacteriologic outcomes for S. pneumoniae correlated with National Committee for Clinical Laboratory Standards (NCCLS) breakpoints and bacteriologic failures correlated with clinical failures. In this study, bacteriologic failures in AOM caused by S. pneumoniae were higher with azithromycin than with amoxicillin–clavulanate, although the difference was not statistically significant (65). Decreased bacteriologic efficacy of azithromycin was subsequently demonstrated in another study (66). These findings suggest that although azithromycin may be an acceptable second-line antibiotic for AOM, its routine use in such a common pediatric infection may lead to increased resistance rates not only to macrolides but also to other antibiotics.
and M. catarrhalis. Choice of antibiotic treatment for AOM depends on the likelihood of bacterial resistance and on compliance-related factors such as frequency, palatability, and cost of the antibiotic. In clinical trials comparing azithromycin with other drugs and in which tympanocentesis was performed at baseline, rates of clinical efficacy were variable. In a study comparing azithromycin with amoxicillin–clavulanate, Dagan et al. (65) showed that azithromycin was less likely to eradicate all bacterial pathogens from middle ear fluid in 136 evaluable patients. Bacteriologic outcomes for S. pneumoniae correlated with National Committee for Clinical Laboratory Standards (NCCLS) breakpoints and bacteriologic failures correlated with clinical failures. In this study, bacteriologic failures in AOM caused by S. pneumoniae were higher with azithromycin than with amoxicillin–clavulanate, although the difference was not statistically significant (65). Decreased bacteriologic efficacy of azithromycin was subsequently demonstrated in another study (66). These findings suggest that although azithromycin may be an acceptable second-line antibiotic for AOM, its routine use in such a common pediatric infection may lead to increased resistance rates not only to macrolides but also to other antibiotics.
Azithromycin and other macrolides may be used as first-line treatment for respiratory tract infections caused by atypical organisms such as M. pneumoniae and C. pneumoniae. Azithromycin for 5 days or erythromycin for 14 days is used to treat C. trachomatis pneumonia in young infants caused by maternal vertical transmission (67).
Azithromycin (10 mg per kg on day 1 followed by 5 mg per kg on days 2 through 5) may be considered for treatment of community-acquired pneumonia (CAP) in children older than 5 years and adolescents who are candidates for outpatient therapy. Harris et al. (68) studied the role of azithromycin in CAP in children. In a double-blind trial, azithromycin was compared with either amoxicillin–clavulanate in children 6 months to 5 years of age or erythromycin in children older than 5 years (68). Although therapeutic outcomes were satisfactory and similar in the two treatment groups, there were differences in pathogens and failure rates between age groups independent of treatment assignment. A significantly higher clinical failure rate was found in younger children than in older ones. However, the role of viral infections in younger patients was not addressed. Azithromycin and other macrolides should not be considered as the first choice for treatment of pneumonia in children younger than 5 years because broad-spectrum activity against atypical organisms is not considered important in this age group.
Treatment of SSTIs with macrolides including azithromycin depends on the local rate of resistance among S. pyogenes and S. aureus strains. The efficacy of azithromycin in treatment of SSTIs has been shown in adults and children (69). In areas where the prevalence of macrolide-resistant S. aureus is high, a child with SSTI should be treated with an antibiotic that is active against β-lactamase-producing strains of S. aureus. In recent years, community-acquired methicillin resistant S. aureus (CA-MRSA) has emerged as a major pathogen causing SSTIs. These strains may be susceptible to clindamycin, trimethoprim–sulfamethoxazole, linezolid, or quinolones but are almost always resistant to macrolides. Clinicians should therefore avoid the use of macrolide for treatment of SSTI in areas of high CA-MRSA prevalence (70).
Macrolides including erythromycin, azithromycin, and clarithromycin are recommended for the treatment and prevention of pertussis (71).
Erythromycin prevents transmission but not the course of illness. The standard treatment and prevention regimen of pertussis remains erythromycin given at a 40 to 50 mg per kg daily for 14 days. However, compliance with this regimen may be affected by the long duration of therapy, the four-times-daily dosing, and the gastrointestinal side effects. B. pertussis is susceptible in vitro to erythromycin, clarithromycin, and azithromycin (72). One study showed that azithromycin given at a dose of 10 mg per kg once daily for 5 days was effective in eradicating B. pertussis from the nasopharynx in eight of eight children after 1 week of treatment (73). In another study, azithromycin given at 10 mg per kg on day 1 followed by 5 mg per kg daily for 4 days was as effective as 10 mg per kg daily for 3 days in eradicating B. pertussis from the upper respiratory tract in infants and young children (74). Because of concerns for pyloric stenosis in young infants due to erythromycin use in the neonatal period, azithromycin is the recommended macrolide for prevention and treatment of pertussis in infants younger than one month (71).
Azithromycin and clarithromycin have also been used in the treatment and prophylaxis of MAC infections. Patients with acquired immune deficiency syndrome (AIDS) are at risk of disseminated MAC. Prophylaxis with either azithromycin or clarithromycin is indicated in all human immunodeficiency virus (HIV)-infected patients with CD4 cell count less than 50 per mL (75). Although macrolide resistance has been identified among some patients receiving prophylaxis, the overall effectiveness remains high (65). MAC has also been identified as a cause of cervical lymphadenitis in children (76,77). The definite treatment of nontuberculous mycobacterial lymphadenitis including MAC is surgical excision. However, if the patient is at high risk of surgical complications or if complete surgical excision is difficult, azithromycin or clarithromycin can be given as an alternative treatment (78).
Azithromycin has been used in treatment of lymphadenitis caused by Bartonella henselae, the agent causing cat scratch disease (CSD). B. henselae has been shown to be susceptible in vitro to different antibiotics. Reports of clinical improvement of CSD with different antibiotics in addition to macrolides included rifampin, trimethoprim–sulfamethoxazole, fluoroquinolones, and gentamicin (79). In a double-blind study, Bass et al. found that azithromycin given as a 5-day course (10 mg per kg on day 1 followed by 5 mg per kg for 4 consecutive days) accelerated the resolution of lymphadenitis caused by typical, uncomplicated CSD within the first month of treatment (80). The efficacy of azithromycin in treatment of other or atypical manifestations of CSD is not clear. However, in normal children, most clinical manifestations of CSD are benign and self-limited, and the majority of infections do not require antibiotic therapy (81).
Erythromycin has historically been the most commonly used drug for treatment of Legionnaires disease. However, the newer macrolides, especially azithromycin, have excellent in vitro activity, fewer adverse events, and greater intracellular and lung tissue distribution. Thus, the newer macrolides, particularly azithromycin,
have become the oral drugs of choice for treatment of Legionnaires disease (82). In critically ill adults with legionellosis, intravenous azithromycin with or without levofloxacin has been recommended (83). The safety and efficacy of intravenous azithromycin in children younger than 16 years have not yet been established.
have become the oral drugs of choice for treatment of Legionnaires disease (82). In critically ill adults with legionellosis, intravenous azithromycin with or without levofloxacin has been recommended (83). The safety and efficacy of intravenous azithromycin in children younger than 16 years have not yet been established.
For treatment of uncomplicated chlamydia cervicitis and nongonococcal urethritis, a single 2-g dose of azithromycin has been shown to be as effective as the traditional 7-day course of oral doxycycline (84).
Azithromycin has been used to treat Cryptosporidium parvum infections in immunocompromised adults and children. C. parvum causes an asymptomatic or self-limiting gastroenteritis in otherwise healthy people. However, in immunocompromised patients, C. parvum may cause severe intestinal fluid losses with dehydration or chronic diarrhea and wasting. Different antimicrobial regimens have been used in adults with inconsistent results (85,86). No specific treatment regimen has produced consistent beneficial effects in children. Azithromycin had been used in nine children aged 14 months to 15 years with cryptosporidiosis associated with AIDS or cancer. The dosages varied from 10 to 40 mg per kg per day for 10 to 31 days. Clinical improvement had been documented early in the course of treatment in some patients (87,88). Some relapses have also occurred. The role of azithromycin in clinical resolution of cryptosporidiosis remains to be clarified.
Azithromycin may also offer an alternative treatment for controlling trachoma. Trachoma is an infectious keratoconjunctivitis caused by C. trachomatis. It is considered the most common cause of preventable blindness worldwide (89). Public health programs have focused on the use of ocular ointments of sulfonamides, tetracyclines, and erythromycin derivatives to control trachoma (90). However, these regimens may be confounded by lack of compliance and frequent side effects. Clinical trials compared oral azithromycin with the standard use of tetracycline or oxytetracycline ointments for treating trachoma in children. In two trials, a single azithromycin dose of 20 mg per kg was as effective as standard ointment therapy (91,92). A third trial showed that three different regimens of azithromycin were as effective as oxytetracycline/polymyxin B ointment (93).
Azithromycin has also been shown to have activity against enteric pathogens including Salmonella typhi (94). Preliminary studies have shown that azithromycin is effective in treating uncomplicated typhoid fever in adults and children (95,96). A study of 64 Egyptian children age 4 to 17 years compared azithromycin suspension given at 10 mg per kg per day for 7 days with intramuscular ceftriaxone given at 75 mg per kg per day for 7 days. Cure was achieved in 91% of the azithromycin group and 97% of the ceftriaxone group (96). None of the children in the azithromycin group had relapses, compared with 14% of those in the ceftriaxone group. Larger studies are needed including children living in areas with significant prevalence of multidrug-resistant S. typhi.
Pharmacokinetic Properties
When administered to children at the regimen of 10 mg per kg once followed by 5 mg per kg per day on days 2 through 5, azithromycin exhibits pharmacokinetic properties similar to those in adults (97). Food intake significantly decreases the bioavailability of the capsule and the powdered suspension of azithromycin. Therefore, azithromycin should be administered 1 hour before or 2 hours after intake of food or antacid. However, the tablet form of azithromycin may be taken without regard to food (98). Peak plasma concentrations are achieved 2 hours after a dose (97). Azithromycin distributes well into tissues including tonsils and adenoid tissues and into middle ear fluid (99,100). Clinically effective levels are achieved in these tissues and middle ear fluid long after the last dose of azithromycin is administered (99). Tissue concentrations as high as twice the serum concentrations may be achieved. Good intracellular penetration of azithromycin contributes to its efficacy in management of infections caused by intracellular organisms such as Chlamydia species and MAC. Tissue delivery is further enhanced by neutrophil and macrophage uptake of azithromycin to sites of infection (101). Despite its fairly rapid absorption and high tissue bioavailability, when azithromycin is given at the recommended dosage regimen, achievable serum concentrations are detected far below the minimum inhibitory concentration of some pediatric pathogens (0.026 to 0.043 μg per mL). This should be taken into consideration during management of potentially bacteremic organisms such as S. pneumoniae, H. influenzae, and S. pyogenes (29). Azithromycin is slowly eliminated. The elimination half-life of azithromycin in children is 32 to 64 hours. This allows once-daily dosing of azithromycin (53). Therapeutically significant concentrations of azithromycin may persist in tissues for additional 5 days after a 5-day course. Plasma protein binding of azithromycin is saturable, ranging from 50% at a concentration of 20 to 50 μg per L to 7% at 1,000 μg per L (56). Azithromycin is largely eliminated unchanged in the urine and stools (98). Significant renal impairment (creatinine clearance of <30 mL per minute) reduces the rate of urinary clearance of azithromycin (98). Azithromycin, like erythromycin, increases the rate of gastric emptying and thus may alter the absorption of azithromycin or other drugs (102).
Dose/Regimens
In most pediatric infections including otitis media and pneumonia, azithromycin is administered in single daily doses at 10 mg per kg on the first day of treatment followed by 5 mg per kg per day on days 2 through 5. The regimen for GABHS pharyngitis is 12 mg per kg per day for 5 days. The maximum daily dose is 500 mg. Azithromycin may be administered as a 3-day course of 10 mg per kg per day or as a single dose of 30 mg per kg for AOM or pneumonia. However, there are no current approved clinical indications for these regimens in pediatric infections. For adolescents and adults with uncomplicated C. trachomatis genital tract infection, azithromycin may be administered as a single 1-g oral dose (67).
For treatment and postexposure prophylaxis of pertussis in children younger than 6 months including neonates, the recommended dose is 10 mg per kg once daily for 5 days.
For children 6 months of age or older, the dose is 10 mg per kg on first day followed by 5 mg per kg once daily for the next 4 days (71).
For children 6 months of age or older, the dose is 10 mg per kg on first day followed by 5 mg per kg once daily for the next 4 days (71).
Intravenous azithromycin is given to adults at a dose of 500 mg once daily. There are no current approved indications for use of intravenous azithromycin in children due to lack of sufficient data. However, a single-daily dose of 10 mg per kg (maximum 500) given to children 6 months to 16 years of age was tolerated with no serious adverse events and showed comparable pharmacokinetic properties among study patients (103).
Adverse Effects/Toxicity/Precautions
Azithromycin is more tolerable than erythromycin. Azithromycin oral suspension has demonstrated an excellent safety and tolerability profile in children treated for different infections. Comparative trials with pooled tolerability results have shown overall better tolerability of azithromycin than other oral comparator agents. Adverse events with azithromycin are usually mild, infrequent, of short duration, and reversible. In children, adverse events also appear to be dose related. They were reported in 7.6% of patients receiving 30 mg per kg and 16.6% of those receiving 60 mg per kg total dose (both given once daily for 5 days). The most prevalent adverse events were gastrointestinal events such as diarrhea (3.1%), vomiting (2.5%), abdominal pain (1.9%), and loose stools (1.0%). Transient elevation of hepatic transaminases has been reported. Dermatologic complications (mainly rash) occurred in 1.5% of patients and neurologic side effects (mainly headache) occurred in 0.6% of patients (104). Children allergic to erythromycin may have cross-hypersensitivity reactions to azithromycin. Azithromycin is contraindicated in children who are allergic to erythromycin or any other macrolide antibiotics.
Drug Interactions
Food, antacids, and H2-blockers reduce the absorption of azithromycin by 50%. Azithromycin reportedly has no significant interactions with carbamazepine, cimetidine, methylprednisolone, terfenadine, or midazolam. Known or potential interactions with other drugs such as cyclosporine, digoxin, theophylline, and warfarin may occur. Levels of these medications should be monitored with concomitant azithromycin use. Azithromycin should not be administered with ergot alkaloids (55).
Clarithromycin
Clarithromycin is a broad-spectrum macrolide that has activity against pathogens causing respiratory tract infections in children. Although clarithromycin has similar in vitro activity to erythromycin, it has the advantage of additional activity against selected pathogens, a longer half-life, enhanced lipophilicity, and increased concentrations in cells and tissues (105).
Chemical Structure
Clarithromycin is a 6-methoxy-erythromycin. The addition of a methoxy group at the 6 position of the macrolide ring results in a compound that is less resistant to acid hydrolysis and therefore has fewer gastrointestinal side effects (106). Clarithromycin is metabolized to an active 14-hydroxy-clarithromycin, a metabolite that has antibacterial activity similar to the parent compound. Thus, the clinical efficacy of clarithromycin and its metabolite against organisms may be higher than that suggested by in vitro susceptibility tests (Fig. 32.3) (107).
Mechanism of Action/Mechanism of Antibacterial Resistance
Similar to other macrolide antibiotics, clarithromycin inhibits protein synthesis by binding to the 50S ribosomal subunit and inhibiting peptide translocation (98). Like other macrolides, the most clinically significant acquired resistance to clarithromycin is of the MLS type and indicates cross-resistance to other macrolides. This resistance is mediated by production of methylases promoted by erm genes (6).
In Vitro Efficacy
Clarithromycin is active against a wide range of gram-positive and gram-negative bacteria, pathogens causing atypical pneumonia (M. pneumoniae, C. pneumoniae, and Legionella species), mycobacteria, and protozoa. Clarithromycin has similar activity to other macrolides against S. pneumoniae, S. pyogenes, S. aureus, and M. catarrhalis. Streptococci and staphylococci that are resistant to erythromycin are also resistant to clarithromycin. Penicillin-resistant S. pneumoniae strains are usually resistant to clarithromycin. Anaerobes are variably susceptible to clarithromycin. Gram-positive anaerobes tend to be more susceptible to clarithromycin than gram-negative anaerobes. Clarithromycin is more active in vitro than erythromycin against C. trachomatis, C. pneumoniae, M. pneumoniae, L. pneumophilia, B. burgdorferi, and B. pertussis (60,106,107). Clarithromycin is ineffective against gram-negative enteric bacteria and methicillin-resistant staphylococci (29,108). H. influenzae is susceptible or intermediately susceptible to clarithromycin alone. However, the antimicrobially active metabolite is approximately twice as active against H. influenzae. Clarithromycin has excellent activity against Haemophilus ducreyi. Clarithromycin is more active
than azithromycin against nontuberculous mycobacteria. Clarithromycin is particularly active against MAC and has activity against other mycobacteria such as Mycobacterium chelonei and some subspecies of Mycobacterium fortuitum. Clarithromycin is also effective against Mycobacterium leprae (29,54). Mycobacterium tuberculosis is less susceptible to clarithromycin than nontuberculous mycobacteria. Clarithromycin has been shown to have inhibitory activity against T. gondii (109).
than azithromycin against nontuberculous mycobacteria. Clarithromycin is particularly active against MAC and has activity against other mycobacteria such as Mycobacterium chelonei and some subspecies of Mycobacterium fortuitum. Clarithromycin is also effective against Mycobacterium leprae (29,54). Mycobacterium tuberculosis is less susceptible to clarithromycin than nontuberculous mycobacteria. Clarithromycin has been shown to have inhibitory activity against T. gondii (109).
Clinical Indications
Clarithromycin has been shown to be an effective alternative to penicillin in treatment of GABHS pharyngitis. Clarithromycin was found to have comparable clinical success rates to penicillin V. It was also associated with higher GABHS eradication rates than penicillin V in one study (110). Clarithromycin has also been shown to be an effective and safe alternative treatment of AOM in children. Following multiple doses of clarithromycin suspension, sustained concentrations of clarithromycin and its 14-hydroxy metabolite were above the MIC of most pathogens causing AOM. Clarithromycin has been shown to have a similar safety and efficacy profile as amoxicillin in treatment of AOM in children (111). In a comparative study of clarithromycin and amoxicillin–clavulanate in treatment of AOM and in which tympanocentesis was performed in 175 of 180 patients, Aspin et al. (112) found that a 10-day course of clarithromycin at 15 mg per kg per day produced similar improvement and cure rates to amoxicillin–clavulanate at 40 mg per kg per day given for 10 days. Recurrence of infection and rates of persistence in middle ear fluid were similar in both groups. However, gastrointestinal side effects were reported less frequently among patients receiving clarithromycin (112). A 10-day course of clarithromycin has also been shown to be as effective and safe as a 3-day azithromycin course in treatment of AOM in children (113). Good clinical response and convenient dosing suggest clarithromycin as an attractive alternative therapy in AOM (114). However, similar to azithromycin, caution should be exercised in routinely prescribing clarithromycin as a first-line treatment in such a common pediatric infection.
Clarithromycin has been found to be effective in the treatment of pneumonia caused by atypical organisms such as M. pneumoniae and C. pneumoniae. Clarithromycin may be used orally in patients with CAP who do not require hospitalization (115). Clarithromycin is active in vitro against B. pertussis but has not been routinely recommended for routine treatment and prophylaxis of pertussis (105).
Clarithromycin is part of the combination therapy for H. pylori-induced peptic ulcer disease (116). Clarithromycin is also part of the multidrug regimen therapy of disseminated MAC infection, which may also include rifampin, isoniazid, ethambutol, and clofazimine (117). Clarithromycin, in combination with other antibiotics, is used for treatment of other nontuberculous mycobacterial infections (118).
Pharmacokinetic Properties
Clarithromycin is well absorbed from the gastrointestinal tract; absorption is not affected by food intake. The oral bioavailability is 52% to 55%. First-pass metabolism results in the appearance of the active 14-hydroxy metabolite (107). Clarithromycin is not extensively protein bound. The major route of elimination is biliary excretion. Approximately 30% to 40% of the administered dose is excreted in urine. Elimination half-life of clarithromycin and its 14-hydroxy metabolite is 5 and 7 hours, respectively (107). Dosage adjustments are required for patients with severe renal failure (creatinine clearance <30 mL per minute). Clarithromycin’s long half-life allows for twice-daily administration. Clarithromycin is concentrated in cells and tissues including lungs, nasal mucosa, and tonsils and in MEF. Clarithromycin and its metabolite concentrate in lungs and middle ear fluid (MEF) at higher levels than in plasma (99). Clarithromycin concentration in respiratory tract exceeds that of plasma by ratios of up to 8.82:1 in MEF, 3.1:1 in bronchial secretions, and 28.7:1 in lung tissue (119,120,121).
Dose/Regimens
The usual dose of clarithromycin in children is 7.5 mg per kg (up to 500 mg per dose) given twice daily. The duration of treatment of AOM and pneumonia in children is 10 days.
Adverse Effects/Toxicity/Precautions
Clarithromycin has fewer gastrointestinal adverse events than erythromycin. The most frequently reported adverse events among children receiving clarithromycin suspension were diarrhea (6.6%), vomiting (6.3%), abdominal pain (2.4%), and headache (1.6%). Adverse events requiring withdrawal of clarithromycin occurred in less than 2% of treated children (29,122). Hypersensitivity reactions range from mild skin rashes and urticaria to anaphylactic reactions and Stevens–Johnson syndrome. Other adverse events include stomatitis, glossitis, oral candidiasis, and dizziness. Unusual reactions reported among adults with underlying conditions include hepatic failure, cholestatic hepatitis, leukocytoclastic vasculitis, thrombocytopenic purpura, and visual hallucinations. Reversible hearing loss has been reported rarely among adults receiving clarithromycin 1,000 mg twice daily for MAC infection (117). The risk of long-term hearing impairment among children taking long-term clarithromycin therapy is unclear.
Drug Interactions
Clarithromycin interacts with other drugs via inhibition of the cytochrome P450 enzyme system, particularly CYP3A (123). In general, clarithromycin exhibits fewer drug interactions than erythromycin. However, clinically significant interactions have been reported with theophylline, digoxin, carbamazepine, cyclosporine, and tacrolimus. These agents require drug level monitoring and appropriate dose adjustment when used concomitantly with clarithromycin. Significant interactions also occur when clarithromycin is concomitantly used with rifampin and rifabutin. Both rifampin and rifabutin induce the metabolism of clarithromycin (124).
Roxithromycin
Roxithromycin is another macrolide antibiotic related to erythromycin.
Mechanism of Action/Mechanism of Antibacterial Resistance
Similar to erythromycin and other macrolides, roxithromycin interferes with protein synthesis by attachment to the 50S rRNA subunit of the bacterial ribosome (125). Roxithromycin has the ability to penetrate cellular walls and concentrate in lymphocytes and macrophages at concentrations that can exceed the plasma concentration. This explains the ability of roxithromycin to treat intracellular organisms (125). In addition, roxithromycin enhances the chemotactic activity of neutrophils. In vitro studies have also shown that roxithromycin may enhance neutrophil apoptosis with resultant decrease in the inflammatory response at sites of tissue infection and inflammation (126,127).
The mechanisms of resistance are similar to other macrolides.
In Vitro Activity
The in vitro activity of roxithromycin is similar to that of erythromycin. Roxithromycin is active against streptococci including viridans streptococci; GABHS; group B, C, F, and G streptococci; and S. pneumoniae. Roxithromycin is also active against atypical and intracellular organisms such as M. pneumoniae, C. trachomatis, B. pertussis, L. pneumophilia, and U. urealyticum. Other in vitro susceptible strains include H. influenzae, H. ducreyi, M. catarrhalis, methicillin-susceptible strains of S. aureus, P. multocida, and Listeria monocytogenes. Although activity against anaerobes including Bacteroides oralis is generally poor, roxithromycin is active against Actinomyces species, Bacteroides urealyticus, Bacteroides melaninogenicus, Peptostreptococcus species, and Propionibacterium acnes. Roxithromycin is not active against the Enterobacteriaceae group as well as the non-fermentative gram-negative bacilli such as Pseudomonas aeruginosa (29,125).
Clinical Indications
Despite the superior pharmacokinetic profile of roxithromycin over erythromycin, roxithromycin has been shown to be equivalent to erythromycin in treatment of different infections. Roxithromycin is effective in treatment of streptococcal pharyngitis, otitis media, SSTIs caused by susceptible organisms, infections in the upper and lower respiratory tract, Lyme disease, isosporiasis, and nongonococcal urethritis (128,129).
Pharmacokinetic Properties
Roxithromycin is rapidly absorbed after oral intake, and absorption is minimally affected by food (130). Peak serum levels (Cmax) are achieved in 2 hours (125). After an initial oral dose of 2.5 mg per kg, serum concentration of roxithromycin remains above the minimum inhibitory concentration for about 12 hours. Steady-state levels in children are reached after 6 days; Cmax of 8 to 10 μg per mL can be reached after a dose of 2.5 mg per kg every 12 hours for 6 days. Approximately 86% to 96% of roxithromycin in serum is protein bound (131,132,133). Roxithromycin has good distribution in various body fluids and tissues including tonsils, adenoids, and middle ear fluid. High levels have been achieved in respiratory tissues and fluids as well as in the male and female genital tracts. Distribution into the CSF and the CNS is poor (134). The distribution half-life of roxithromycin is approximately 4 hours. Because of the higher volume of distribution in children compared with adults, elimination half-life is 20 hours compared with 12 hours in adults (132). Roxithromycin is not extensively metabolized. Approximately 53% of roxithromycin dose is excreted in feces and 10% is excreted in the urine (134). The elimination half-life is increased in patients with hepatic or renal insufficiency, and dosage adjustments may be needed (134).
Dose/Regimen
The dose in children for most clinical indications is 2.5 to 5 mg per kg every 12 hours. The duration of treatment depends on the severity and type of infection. The usual adult dose is 150 to 300 mg twice daily.
Adverse Effects/Toxicity/Precautions
The most common adverse events associated with roxithromycin use are gastrointestinal events, occurring in 5% of patients. These include anorexia, abdominal cramps, nausea, vomiting, and diarrhea (135). Other mild adverse events are headache and itching, and have been reported in 1% to 5% of patients receiving roxithromycin (136). Asymptomatic elevation of liver enzymes has been reported in patients receiving roxithromycin. Individual case reports of substantial liver enzyme elevation and fulminant hepatitis have also been reported (137). Other rarely reported adverse events include transient eosinophilia and thrombocytosis, pancreatitis, hyperglycemia, and nail discoloration. However, a causal relationship with roxithromycin therapy has not been established (138,139,140).
Drug Interactions
Although roxithromycin has a less inhibitory effect on cytochrome P450 enzymes than does erythromycin, some reports suggest that roxithromycin can interact with other medications leading to potentially significant clinical interaction. Similar to other macrolide antibiotics, roxithromycin may decrease the metabolism of other medications such as benzodiazepines, causing elevated plasma concentrations of these medications (141). Concomitant administration of roxithromycin and carbamazepine was not found to affect the pharmacokinetics of carbamazepine (142). Potential decreased intestinal degradation of digoxin by roxithromycin can cause an increase in digoxin serum concentration (143). Like erythromycin, roxithromycin has the potential to exacerbate the cardiac toxic effects of astemizole and pimozide when roxithromycin is concomitantly administered with either drug
(144). Roxithromycin does not appear to interfere with the efficacy of oral contraceptives (145). Coadministration of proton pump inhibitors and roxithromycin does not alter the bioavailability of either medication. The interaction potential of roxithromycin with theophylline or warfarin is controversial (146,147,148).
(144). Roxithromycin does not appear to interfere with the efficacy of oral contraceptives (145). Coadministration of proton pump inhibitors and roxithromycin does not alter the bioavailability of either medication. The interaction potential of roxithromycin with theophylline or warfarin is controversial (146,147,148).
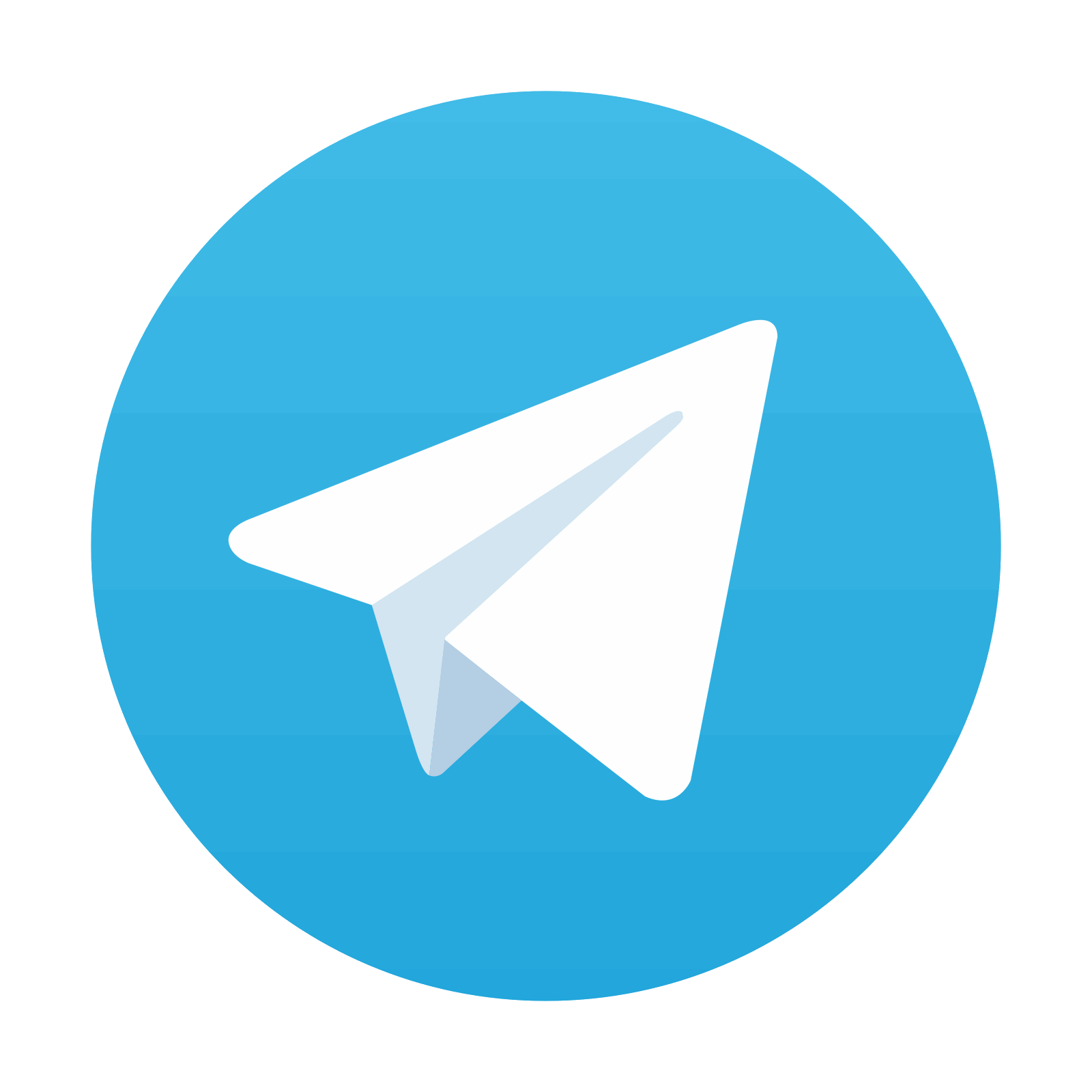
Stay updated, free articles. Join our Telegram channel

Full access? Get Clinical Tree
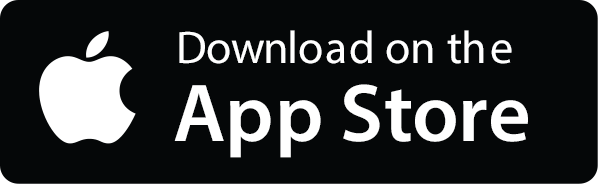
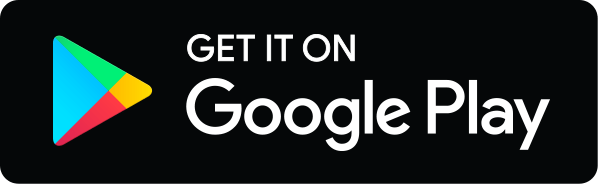
