With the use and adoption of computer-assisted laparoscopic technology gaining more prominence, important issues pertaining to the learning process are raised. Several modalities can be incorporated into a training program for robotic surgical development. The role and utility of various methods, including didactic instruction, virtual reality simulators, dry and wet laboratories, bedside assistance, mentoring, as well as proctorship, are still in the process of being assessed and validated. Integration of robotic training in residency and fellowship programs as well as the formation of a structured didactic robotic curriculum continues to be a challenge. Finally, methods to assess competency of training and the process for credentialing robotic surgeons still require further structuring and codification.
Introduction
The advent of computer-enhanced surgical technology has enabled many surgeons to overcome difficulties associated with conventional laparoscopy . Today, these telemanipulation systems represent the latest developments in minimally invasive surgery as they offer an improved ergonomic position to the surgeon, three-dimensional visualization of the operating field, fine instrumentation, and increased maneuverability of the instruments with 6df . The da Vinci® Surgical System (Intuitive Surgical, Inc., Sunnyvale, CA, USA) has become the most popular system for robotic-assisted laparoscopic surgery (RALS) . In the past decade, over 1.5 million operations have been performed with the da Vinci Surgical System and an increasing number of national and international centers are adopting robots in specialties including gynecology, urology, cardiac, thoracic, as well as head and neck and general surgery .
Due to the rapid uptake of robotic-assisted surgery, proper training and guidelines for safe use of this technology are necessary. Currently, no standardized system exists to evaluate surgeon competency and safety with the robotic platform. In addition, robotic surgical training poses unique challenges to educators, trainees, and institutions. During video laparoscopy and open surgery, the mentoring surgeon is scrubbed in the surgical field next to the trainee, is able to operate in tandem with the trainee, and is able take over the case at any given moment where patient safety may be compromised. This is currently not the case with robotic-assisted procedures as only one surgeon can be operating at any given time. Therefore, a certain level of skills and competency should be achieved prior to performing live cases on the console.
From the trainees’ perspective, with limits in working hours, fear of litigation, and financial constraints, the prospect of training in robotic surgery can be a daunting task . Currently, training surgeons for robotic techniques has been unstructured and the requirements have differed from hospital to hospital . Trainees and program directors have recognized that the traditional “on-the-job” training can be difficult in the context of robotic surgery. Therefore, alternative methods such as structured didactic courses, simulation training, mentoring of cases, proctorship, and minimally invasive surgery fellowship programs need to be sought to achieve competency .
Knowledge-based Training
In addition to the basic knowledge of anatomy, energy, and devices, the trainee/surgeon must understand robotic technology by acquiring knowledge about device functions and parameters, basic trouble shooting, and limits of the system. Didactic training must be also dedicated to acquiring knowledge of pelvic and abdominal anatomy that are specific to minimally invasive surgery. Inherent in endoscopic surgery, magnification of the surgical field and panoramic and close-up views make the orientation and manipulation different from laparotomy . Nezhat et al. proposed a formal gynecological endoscopy curriculum for obstetrics and gynecology residents, fellows in AAGL/SRS-sponsored fellowship programs . The curriculum defines several core objectives that should be mastered at various stages of training of minimally invasive surgery . Didactic objectives from this curriculum are applicable for didactic education in robotic surgery. After the first quarter, the trainer should be able to do the following :
- 1.
Identify normal female pelvic anatomy
- 2.
Identify divergence from normal anatomy
- 3.
Identify and endoscopically trace major pelvic blood vessels, ureters, bowel, anatomic hallmarks, and abdominal wall ligaments, among other features
- 4.
Know major branches of anterior and posterior divisions of the internal iliac artery and the implication of injury to a particular branch
- 5.
Name the major nerve supplies to the pelvis (e.g., superior and inferior hypogastric plexuses, obturator nerve, and ilioinguinal and genitofemoral nerves
- 6.
Understand the physiology and principles of creating and maintaining pneumoperitoneum
- 7.
Understand the principles of electrosurgery
- 8.
Know the difference between monopolar and bipolar electrosurgery
- 9.
Understand the principles of ultrasonic energy.
The next step of knowledge-based training is the understanding of the fundamentals necessary to performing specific surgical procedures. This includes knowledge of appropriate patient selection and indications for an RALS, preoperative preparation, patient and system positioning, port placement, system docking, procedural steps, complications and their management, and postoperative care. The objectives from the second quarter of the Nezhat curriculum are consistent with these goals :
- 1.
Appropriate patient selection and preoperative assessment including risks and benefits of counseling
- 2.
Ability to communicate with the operating room (OR) and anesthesia staff about patient positioning and preparation for surgery
- 3.
Ability to perform safe abdominal entry
- 4.
Knowledge of major limitations and pitfalls of laparoscopic abdominal entry
- 5.
Familiarity with the current literature about vascular and visceral complications of various types of abdominal entry
- 6.
Ability to describe different and alternative sites of abdominal entry and their indications including establishment of pneumoperitoneum
- 7.
Ability to perform safe diagnostic laparoscopy
- 8.
Knowledge of the differences between multifunctional electrosurgical, vessel-sealing devices and tissue-dissecting devices
Knowledge-based Training
In addition to the basic knowledge of anatomy, energy, and devices, the trainee/surgeon must understand robotic technology by acquiring knowledge about device functions and parameters, basic trouble shooting, and limits of the system. Didactic training must be also dedicated to acquiring knowledge of pelvic and abdominal anatomy that are specific to minimally invasive surgery. Inherent in endoscopic surgery, magnification of the surgical field and panoramic and close-up views make the orientation and manipulation different from laparotomy . Nezhat et al. proposed a formal gynecological endoscopy curriculum for obstetrics and gynecology residents, fellows in AAGL/SRS-sponsored fellowship programs . The curriculum defines several core objectives that should be mastered at various stages of training of minimally invasive surgery . Didactic objectives from this curriculum are applicable for didactic education in robotic surgery. After the first quarter, the trainer should be able to do the following :
- 1.
Identify normal female pelvic anatomy
- 2.
Identify divergence from normal anatomy
- 3.
Identify and endoscopically trace major pelvic blood vessels, ureters, bowel, anatomic hallmarks, and abdominal wall ligaments, among other features
- 4.
Know major branches of anterior and posterior divisions of the internal iliac artery and the implication of injury to a particular branch
- 5.
Name the major nerve supplies to the pelvis (e.g., superior and inferior hypogastric plexuses, obturator nerve, and ilioinguinal and genitofemoral nerves
- 6.
Understand the physiology and principles of creating and maintaining pneumoperitoneum
- 7.
Understand the principles of electrosurgery
- 8.
Know the difference between monopolar and bipolar electrosurgery
- 9.
Understand the principles of ultrasonic energy.
The next step of knowledge-based training is the understanding of the fundamentals necessary to performing specific surgical procedures. This includes knowledge of appropriate patient selection and indications for an RALS, preoperative preparation, patient and system positioning, port placement, system docking, procedural steps, complications and their management, and postoperative care. The objectives from the second quarter of the Nezhat curriculum are consistent with these goals :
- 1.
Appropriate patient selection and preoperative assessment including risks and benefits of counseling
- 2.
Ability to communicate with the operating room (OR) and anesthesia staff about patient positioning and preparation for surgery
- 3.
Ability to perform safe abdominal entry
- 4.
Knowledge of major limitations and pitfalls of laparoscopic abdominal entry
- 5.
Familiarity with the current literature about vascular and visceral complications of various types of abdominal entry
- 6.
Ability to describe different and alternative sites of abdominal entry and their indications including establishment of pneumoperitoneum
- 7.
Ability to perform safe diagnostic laparoscopy
- 8.
Knowledge of the differences between multifunctional electrosurgical, vessel-sealing devices and tissue-dissecting devices
Skill-based Training
Skill-based training involves acquiring the technical ability to perform a given operation safely. It is necessary to master several skills specific to robotic surgery. One must learn hand–eye coordination using the three-dimensional interface with the ability to simultaneously control the robotic arms and manipulate the camera. The trainee must also become familiar with the loss of haptic feedback associated with the robotic platform. Several modalities can be used to develop skill-based training for robotic surgery. These include the use of mechanical or virtual reality simulators, laboratory training in a dry or wet setting, and mentorship and proctoring programs .
Simulation Training
A simulator is an educational tool that allows interactive performance of a particular task in an environment that recreates or replicates a real-world clinical scenario . Surgical simulator training can be separated into two broad categories: physical (mechanical) simulators, wherein the task is performed under videoscopic guidance in the real-world environment (i.e., box trainer) and “virtual reality” (VR) simulators, wherein the task is performed on a computer-based platform using an artificially generated virtual environment . Due to the availability of a variety of simulators, the evidence supporting the effectiveness of the various platforms in terms of feasibility, reliability, validity, acceptability, educational impact, and cost-effectiveness must be explored .
Before a surgical simulator can be used to assess the competency of a trainee, the simulator must undergo initial testing across a variety of parameters. This would include the assessment of face validity , which examines how closely the simulator resembles the real task; construct validity , the ability to differentiate groups with different levels of competence; content validity , examines whether the intended content domain is actually being measured by the device/exercise ; concurrent validity , the extent to which the results of the test correlate with the “gold standard” tests known to measure the same domain; and predictive validity , the extent to which an assessment will predict future performance .
At present, four major robotic simulation devices are available in the market, which have undergone validation studies: da Vinci Skills Simulator® (dVSS, Intuitive Surgical, Inc., Sunnyvale, CA, USA), Mimic dV-Trainer® (MdVT, Mimic Technologies, Inc., Seattle, WA, USA), Robotic Surgery Simulator (RoSS ®, Simulated Surgical Systems; Williamsville, NY, USA), and SimSurgery Educational Platform® (SEP, SimSurgery, Oslo, Norway) .
The dVSS consists of a small case that generates the virtual environment. The case is annexed to an existing da Vinci surgeon console, thereby transforming it into a simulation platform. The primary features are built-in metrics that enable the trainee to assess skills and to measure the improvement in a given exercise, with real-time feedback and progress tracking . Exercises range from beginning to advanced and cover the following five categories: EndoWrist® manipulation, camera and clutching, fourth arm integration, system setting, needle control and driving, as well as energy and dissection. During the developmental stages, several studies have shown face, construct, and content validity for the dVSS . Recently, Hung et al. were able to demonstrate concurrent and predictive validity in a group of trainees who underwent a 10-week simulation course with dVSS by evaluating ex vivo tissue performance (concurrent validity) and final tissue performance (predictive validity) after completion of the course . The dVSS uses the same console used for operative procedures, thus making it advantageous. However, the cost and logistics for securing a console of training purposes may be a prohibitive factor in some institutions.
The MdVT is a stand-alone simulator built on a compact hardware platform engineered to closely reproduce the look and feel of the dVSS. However, unlike the dVSS, it does not require an existing console. It incorporates a software application called Mimic’s MScore™, that objectively evaluates performance from the data collected from experienced surgeons for establishing proficiency-based scoring baselines. The scoring is based on time to completion, economy of motion, instrument collisions, number of drops, missed targets, instruments out of view, blood loss, fractured vessels, excessive instrument force, and misapplied energy . Several studies have shown face, construct, and content validity for MdVT as a training tool .
The RoSS is also a stand-alone simulator. The system uses virtual reality to create a clinical case scenario in order to simulate surgical experiences ranging from beginner to advanced levels . Thus, an innovative feature of this simulator is that it offers an opportunity to develop technical skills while guiding the trainee through the operative steps of a real surgical procedure . Progression through the simulation module is based on checklist-based learning interface, meaning the user can only proceed through the procedure after learning and executing each skill successfully . Like the dVSS and Mimic trainers, the RoSS simulator incorporates the following characteristics: EndoWrist® manipulation, camera and clutching exercises, fourth arm integration, needle control and needle driving, energy and dissection exercises, as well as performance feedback . The RoSS® has demonstrated face and content validity , but studies have not been able to demonstrate construct validity , thereby making it less useful in discriminating between novices and experts.
The SEP is another available option. It lacks some of the advanced features of other simulators, but stills offers modules on EndoWrist® manipulation and performance feedback. Basic and advanced skills are organized into three groups: tissue manipulation, basic suturing, and advanced suturing . SEP has shown face, construct, and content validity, and its market price is lower than that of the simulators described previously .
Dry laboratory exercises using the da Vinci console and robot have demonstrated face, content, and construct and concurrent validity that correspond to virtual reality simulators . Animal and cadaver simulation models have the advantage of simulating the human anatomy and therefore can be used for procedural training. This type of training has been incorporated in training courses across several disciplines . In vivo training allows the trainee to practice docking the robot as well as camera insertion and instrument changes, and affords an opportunity to troubleshoot possible difficulties with equipment and maintaining pneumoperitoneum. Some drawbacks of in vivo training include the increased cost and the necessity to have a separate area and robot/console devoted exclusively for training purposes. In many countries (including Australia and the UK), this type of training is not allowed . In other countries, including the USA, in vivo training on porcine models has partially been an industry-driven process, as the industry strongly recommends that an in vivo training course be undertaken in one of their official training facilities before surgeons are given the credentials to use this technology. Currently, the American Urology Association (AUA) Standard Operating Practices for awarding credentials for robotic surgery list in vivo training as being optional ; however, recommendations across disciplines have not been standardized.
Virtual reality simulators, dry lab, and wet lab exercises are tools for novice surgeons to acquire surgical skills in a safe and structured environment; however, few studies exist to demonstrate superiority of any one modality. Hung et al. conducted a study to simultaneously correlate the performance of expert and novice/trainee robotic surgeons across inanimate, virtual reality, and in vivo platforms using a global assessment tool . The study confirmed the construct validities of the three robotic training methods (experts outperformed novices with all three methods) and demonstrated significant cross-method correlation (overall performance of inanimate tasks significantly correlated with virtual reality robotic performance and in vivo robotic performance) amongst a diverse cohort of both expert surgeons and novice operators . Of note, the performance of the inanimate tasks showed the strongest correlation to in vivo robotic performance .
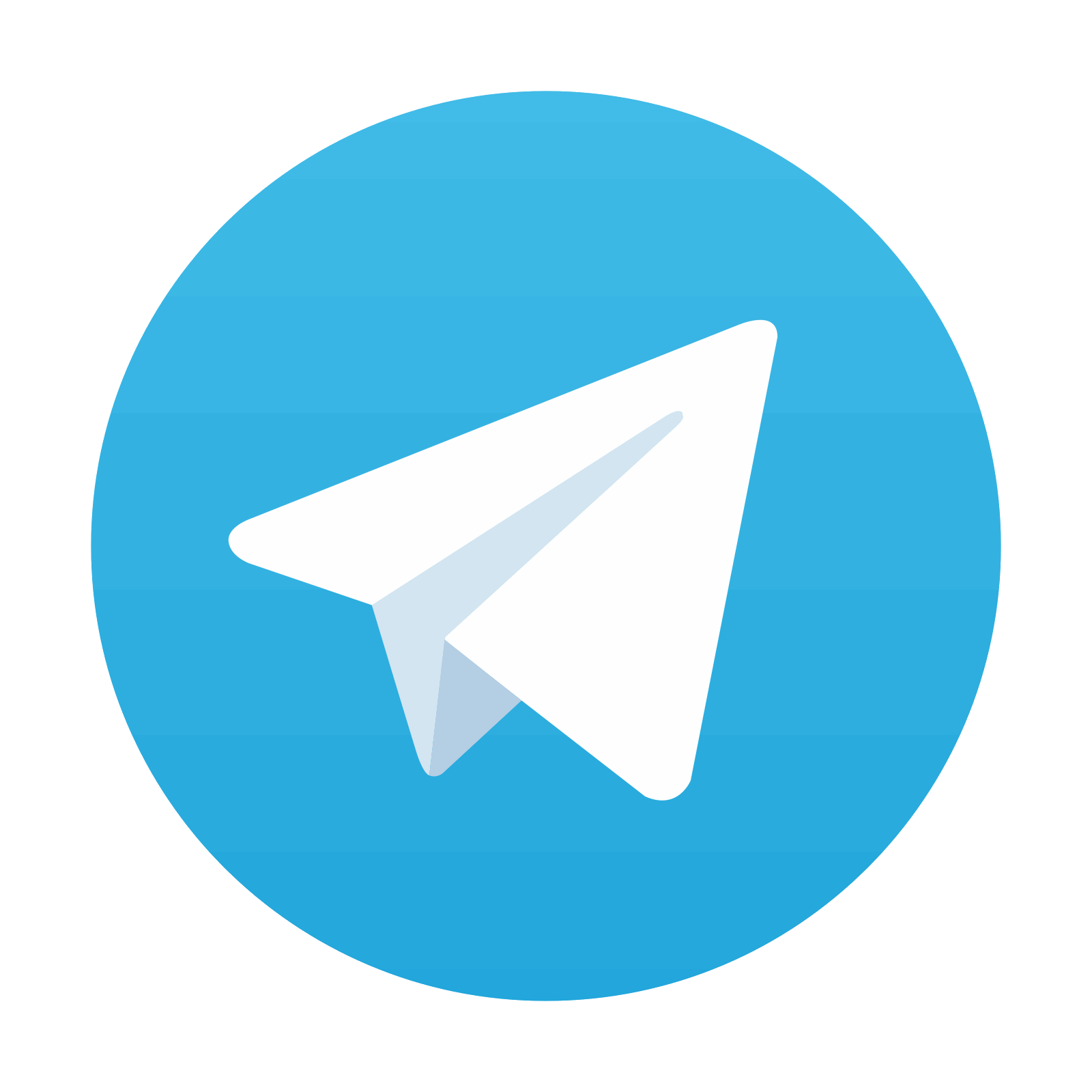
Stay updated, free articles. Join our Telegram channel

Full access? Get Clinical Tree
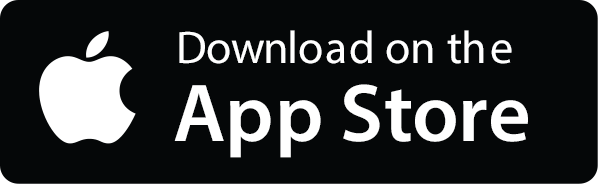
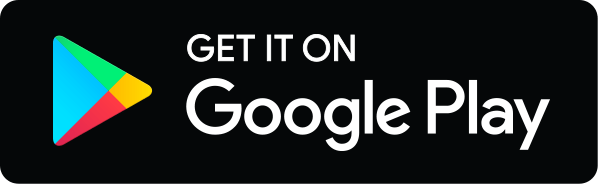