Treatment for childhood cancer with chemotherapy, radiation and/or hematopoietic cell transplant can result in adverse sequelae that may not become evident for many years. A clear understanding of the association between therapeutic exposures and specific long-term complications, and an understanding of the magnitude of the burden of morbidity borne by childhood cancer survivors, has led to the development of guidelines to support lifelong risk-based follow up for this population. It is important to develop interventions to reduce the impact of treatment-related late effects on morbidity and mortality and to continue research regarding the etiopathogenesis of therapy-related cancers and other late effects.
Key points
- •
As survival rates for pediatric cancers continue to improve, the number of childhood cancer survivors continues to increase.
- •
The burden of long-term therapy-related morbidity experienced by childhood cancer survivors is substantial.
- •
Childhood cancer survivors require lifelong follow-up care to monitor for late treatment-related sequelae.
Introduction
With advances in therapeutic strategies for common childhood malignancies such as leukemia, lymphoma, and central nervous system (CNS) tumors, the number of childhood cancer survivors in the United States continues to increase, and is estimated to exceed 500,000 by 2020. About 1 of every 530 young adults between 20 and 39 years of age in the United States is a childhood cancer survivor. Treatment of childhood cancer with chemotherapy, radiation, or hematopoietic cell transplant (HCT) can result in adverse sequelae, which may not become evident for many years. In this article, commonly occurring late effects associated with childhood cancer treatment are reviewed.
Introduction
With advances in therapeutic strategies for common childhood malignancies such as leukemia, lymphoma, and central nervous system (CNS) tumors, the number of childhood cancer survivors in the United States continues to increase, and is estimated to exceed 500,000 by 2020. About 1 of every 530 young adults between 20 and 39 years of age in the United States is a childhood cancer survivor. Treatment of childhood cancer with chemotherapy, radiation, or hematopoietic cell transplant (HCT) can result in adverse sequelae, which may not become evident for many years. In this article, commonly occurring late effects associated with childhood cancer treatment are reviewed.
Burden of morbidity
The burden of morbidity experienced by childhood cancer survivors is substantial, as shown by the fact that approximately 40% of childhood cancer survivors experience a late effect that is severe, life threatening, disabling, or fatal at 30 years from diagnosis. A primary diagnosis of Hodgkin lymphoma (HL) or brain tumors and exposure to chest radiation or anthracyclines increases the risk of these chronic health conditions. Furthermore, the burden of morbidity increases as the cohort ages. HCT recipients experience a higher burden of morbidity when compared with childhood cancer survivors treated with conventional therapy. The potential for serious therapy-related sequelae provide the rationale for ongoing follow-up of childhood cancer survivors into adult life.
Standardized recommendations for follow-up of childhood cancer
In response to a call from the Institute of Medicine for a systematic plan for lifelong surveillance of cancer survivors, the Children’s Oncology Group (COG) developed exposure-related, risk-based guidelines ( Long-Term Follow-Up Guidelines for Survivors of Childhood , Adolescent , and Young Adult Cancers ) for follow-up of patients treated for pediatric malignancies. Specially tailored patient education materials, known as Health Links, accompany the Guidelines to enhance health promotion in this population. The Guidelines and the Health Links can be downloaded from http://www.survivorshipguidelines.org . Recommendations for screening of specific treatment-related late effects are summarized in Table 1 . The COG Guideline group, along with several other guideline groups addressing survivorship care, have initiated the international harmonization of long-term follow-up guidelines for childhood cancer survivors. To use these guidelines, the first step entails the development of a treatment summary ( Box 1 ). This treatment summary allows the survivor and their health care provider to determine recommended follow-up care according to the guidelines. In this article, the more commonly occurring late effects in survivors of childhood cancer, and the relationship between these late effects and specific therapeutic exposures, are reviewed, to suggest reasonable starting points for evaluation of specific long-term problems using the screening recommendations from the COG Long – Term Follow – Up Guidelines .
Adverse Outcome | Therapeutic Exposures Associated with Increased Risk | Factors Associated with Highest Risk | Recommended Screening |
---|---|---|---|
Adverse psychosocial effects | Any cancer experience | CNS tumors Cranial irradiation Hearing loss Older age at diagnosis |
|
Neurocognitive deficits | Cranial irradiation Methotrexate (intrathecal, high-dose IV) Cytarabine (high-dose IV) | Female sex Younger age at treatment Cranial irradiation Intrathecal methotrexate |
|
Hearing loss | Cranial irradiation Cisplatin Carboplatin (at myeloablative doses or if given during infancy) | Younger age at treatment Higher doses of chemotherapy and radiation |
|
Cataracts | Cranial irradiation Total body irradiation Corticosteroids | Higher radiation dose Single daily radiation fraction Combination of corticosteroids and radiation |
|
Dental abnormalities | Cranial irradiation Receipt of any chemotherapy before development of permanent dentition | Younger age at treatment |
|
Cardiomyopathy Congestive heart failure | Anthracycline chemotherapy Chest and spinal irradiation | Anthracycline cumulative dose >500 mg/m 2 Female sex Younger age at treatment |
|
Atherosclerotic heart disease Myocardial infarction Valvular disease | Chest and spinal irradiation | Mediastinal irradiation | |
Pulmonary fibrosis Interstitial pneumonitis | Bleomycin, carmustine, lomustine, busulfan Chest or whole lung irradiation | Younger age at treatment Bleomycin dose >400 U/m 2 |
|
Hepatic dysfunction | Methotrexate Mercaptopurine Thioguanine Liver irradiation | Previous veno-occlusive disease of the liver Chronic viral hepatitis |
|
Renal dysfunction (glomerular or tubular) | Cisplatin Carboplatin Ifosfamide High-dose methotrexate Abdominal irradiation Nephrectomy | High-dose chemotherapy Younger age at treatment Abdominal radiation |
|
Bladder complications | Alkylating agents Abdominal/pelvic irradiation Surgery involving the bladder | Higher-dose alkylating agents administered without bladder uroprophylaxis Abdominal/pelvic irradiation |
|
Obesity | Cranial irradiation Neurosurgery involving the hypothalamic-pituitary axis | Younger age at treatment Female sex Cranial irradiation >20 Gy |
|
Hypothyroidism | Radiation impacting the thyroid gland (eg, neck, mantle) | Increasing radiation dose Female sex Older age at treatment |
|
Precocious puberty | Cranial irradiation | Female sex Younger age at treatment Cranial irradiation >18 Gy |
|
Hypogonadism (Leydig cell dysfunction in males; acute or premature ovarian failure in females) | Alkylating agents Craniospinal irradiation Abdominopelvic irradiation Gonadal irradiation | Higher cumulative doses of alkylating agents Gonadal irradiation Females: treatment during the peripubertal or postpubertal period |
|
Infertility | Alkylating agents Craniospinal irradiation Adominopelvic irradiation Gonadal irradiation | Male sex Higher doses of alkylating agents Gonadal irradiation Total body irradiation |
|
Growth hormone deficiency | Cranial irradiation | Cranial irradiation ≥18 Gy |
|
Short stature Musculoskeletal growth problems | Cranial irradiation Corticosteroids Total body irradiation | Younger age at treatment Cranial radiation dose >18 Gy Unfractionated (10 Gy) total body irradiation |
|
Scoliosis/kyphosis | Radiation involving the chest, abdomen, or spine Thoracic surgery Neurosurgery involving the spine | Younger age at irradiation Higher radiation doses Hemithoracic, abdominal, or spinal surgery |
|
Reduced bone mineral density | Corticosteroids Craniospinal irradiation Gonadal irradiation Total body irradiation | Hypothyroidism Hypogonadism Growth hormone deficiency |
|
Osteonecrosis | Corticosteroids High-dose radiation to any bone | Dexamethasone Adolescent age at treatment Female sex |
|
Life-threatening infection | Splenectomy Radiation impacting the spleen (≥40 Gy) Chronic active graft-versus-host disease | Anatomic asplenia Higher radiation doses to the spleen Ongoing immunsuppression Hypogammaglobulinemia |
|
Chronic HCV infection and HCV-related sequelae | Transfusions before 1993 | Living in hyperendemic area |
|
Therapy-related myelodysplasia Therapy-related acute myeloid leukemia | Alkylating agents Epipodophyllotoxins Anthracyclines | Increasing dose of chemotherapeutic agents Older age at treatment Autologous hematopoietic cell transplant |
|
Skin cancer (basal cell, squamous cell, melanoma) | Radiation (any field) | Orthovoltage radiation (before 1970): delivery of greater dose to skin Additional excessive exposure to sun or tanning booths |
|
Secondary brain tumor | Cranial irradiation | Increasing radiation dose Younger age at treatment |
|
Thyroid cancer | Radiation impacting the thyroid gland (eg, neck, mantle) | Increasing radiation dose up to 29 Gy Female sex Younger age at radiation |
|
Breast cancer | Chest irradiation | Increasing radiation dose Female sex Longer time because radiation |
|
Colorectal cancer | Abdominal/pelvic irradiation Spinal irradiation | Higher radiation dose to bowel Higher daily dose fraction Combined with chemotherapy (especially alkylating agents) |
|
Key Elements | Details |
Demographics | Name, sex, date of birth, treating institution and key team members |
Diagnosis | Diagnosis, date, site, stage Relapse(s), if applicable, with date(s) and site(s) Subsequent malignant neoplasm(s), if applicable, with type(s) and site(s) Completion of therapy date(s) |
Therapeutic Exposures | |
Chemotherapy | Names of chemotherapy agents received Routes of administration Cumulative doses (per m 2 ) for alkylators, anthracyclines, and bleomycin (at minimum) Intermediate/high (≥1000 mg/m 2 ) vs standard dosing for intravenous methotrexate and cytarabine Standard vs myeloablative dosing for carboplatin |
Radiation | Dates, type, field(s), total dose, number of fractions, dose per fraction |
Surgical procedures | Type(s), date(s) |
Hematopoietic cell transplant | Type(s), date(s), graft-versus-host disease prophylaxis/treatment |
Auditory impairment
Children with cancer often require therapy with potentially ototoxic agents, including platinum-based chemotherapy, aminoglycoside antibiotics, loop diuretics, and radiation therapy. These agents are all capable of causing sensorineural hearing loss. Risk for hearing loss is increased with higher doses of platinum-based chemotherapy, particularly cisplatin in cumulative doses exceeding 360 mg/m 2 and myeloablative doses of carboplatin, combining platinum chemotherapy with cranial irradiation, treatment with multiple ototoxic agents, age younger than 5 years at treatment, and surgery that involves cranial nerve VIII. Radiation-related hearing loss may be multifactorial. Although sensorineural loss increases in association with high doses of radiation involving the ear, treatment with higher doses of radiation has also been associated with conductive hearing loss.
Cognitive sequelae
Childhood cancer survivors are at risk for impaired cognition. Cranial radiation is a well-established risk factor for cognitive impairment, although corticosteroids and antimetabolite chemotherapy have been implicated as contributors. Cognitive impairment usually become evident within 1 to 2 years after cranial radiation and is progressive, likely because of the child’s failure to acquire new abilities at a rate similar to peers. Affected children experience academic difficulties, resulting in problems with receptive and expressive language and attention span. Fatigue and sleep disruption also serve as contributors to the cognitive impairment observed in childhood cancer survivors.
Brain tumor survivors are especially vulnerable to impairment in neurocognitive functioning; young age at exposure to cranial radiation, cerebrovascular events, and hearing or motor disabilities contribute to the vulnerability. Cranial radiation dose has a direct association with cognitive impairment. Cognitive impairment manifests as slow processing and psychomotor speed, inattention, memory impairment, and deficits in verbal and visual-spatial skills. These impairments have a negative impact on societal reintegration, such as education, employment, income, and marital status.
Survivors of childhood acute lymphoblastic leukemia (ALL) are also at risk for cognitive impairment, presenting as inattention, and impaired processing speed, executive function, and global intellectual function. Exposure to 24-Gy cranial radiation, younger age at radiation exposure, and female sex are associated with increased risk. This impairment is associated with reduced educational attainment, unemployment, and independent living. Cognitive deficits have been observed after treatment with chemotherapy alone and include deficits in attention, executive function, and complex fine-motor functioning; global intellectual function is relatively preserved.
Cardiovascular function
Cardiovascular complications such as coronary artery disease, stroke, and especially heart failure have emerged as a leading cause of morbidity and mortality in aging survivors of childhood cancer. These survivors are at a 9-fold risk of having a stroke, 10-fold risk of developing coronary artery disease, and 15-fold risk of developing heart failure when compared with the general population. This increased risk is caused by the combined effects of cardiotoxic cancer treatments (anthracycline chemotherapy, radiation) and traditional cardiovascular risk factors (hypertension, diabetes, dyslipidemia, obesity) that emerge later in life.
Anthracycline chemotherapy increases the risk of developing heart failure in a dose-dependent manner; the incidence of heart failure is less than 5%, with cumulative anthracycline dose of less than 250 mg/m²; approaches 10% at doses between 250 and 600 mg/m²; and exceeds 30% for doses greater than 600 mg/m². Chest radiation, when the heart is in the treatment field, and cardiovascular risk factors, such as hypertension and diabetes, can substantially increase the risk of anthracycline-related heart failure. In cancer survivors, heart failure is associated with a poor prognosis; 5-year overall survival rates are reported to be less than 50%.
Different anthracycline analogues with potential for decreased cardiotoxicity have been studied, but long-term data regarding their efficacy in heart failure risk reduction are lacking. Cardioprotectants such as dexrazoxane have been shown to minimize cardiac injury shortly after anthracycline administration without compromising its antitumor efficacy. As in anthracycline analogues, long-term data on efficacy of dexrazoxane are lacking, and certain subgroups, particularly children, who have the greatest potential number of life years after cancer therapy, remain understudied. On the other hand, advances in cardiac imaging have helped set the stage for the development of novel prevention strategies in asymptomatic survivors at high risk for therapy-related heart failure. These advances include closer monitoring, better management of cardiovascular risk factors such as hypertension and diabetes, and consideration of pharmacologic therapy (angiotensin-converting enzyme inhibitors or β-blockers) in high-risk individuals with early changes in cardiac function.
Chest radiation therapy has been implicated in the development of constrictive pericarditis, valvular heart disease, coronary artery disease, conduction abnormalities, and heart failure. Clinically evident heart failure, although rare after chest radiation alone, is primarily manifested as left ventricular diastolic dysfunction, in contrast to the systolic dysfunction typically seen after anthracycline exposure. On the other hand, premature coronary artery disease is a well-recognized complication of radiation therapy, with a reported cumulative incidence of 21% at 20 years in individuals treated with chest radiation. However, clinically significant coronary artery disease rarely occurs in the absence of other cardiovascular risk factors such as obesity, hypertension, and dyslipidemia, emphasizing the importance of management of these risk factors to reduce subsequent coronary artery disease risk.
Pulmonary function
The lungs are exceptionally susceptible to radiation-related damage. The prevalence and extent of radiation-related lung injury are dependent on several factors, including age at exposure, total radiation dose, number of fractions, radiation type, and total lung volume in the radiation field. In very young children, the basis for respiratory damage seems to differ from that seen in adolescents or adults and is likely the result of hypoplasia of the chest wall and compromised growth of the lung parenchyma, as shown by deficits in total lung capacity, forced vital capacity, and diffusion capacity of the lung. Although refined radiation therapy techniques have resulted in a dramatically decreased incidence of pulmonary toxicity over the past 2 decades, patients who have received pulmonary radiation during childhood continue to remain at risk for declining pulmonary function over time.
Several chemotherapeutic agents are also associated with pulmonary damage, including bleomycin, busulfan, lomustine, and carmustine. The pulmonary toxicity of these agents may be increased when combined with radiation. Contemporary pediatric cancer therapy rarely includes high doses of bleomycin, decreasing the likelihood that the growing population of childhood cancer survivors will be at risk for clinically significant bleomycin-induced pulmonary disease later in life.
On the other hand, pulmonary complications remain a leading cause of morbidity and mortality in long-term survivors of HCT. This situation is largely because of the additive effects of therapy delivered before transplant and the intensity of therapy required during HCT, which magnify the risk. Obliterative bronchiolitis (BO) is an irreversible chronic obstructive lung disorder, which may occur months to years after allogeneic HCT. Radiographic imaging of patients with BO is characteristic for lung hyperinflation, and parenchymal hypoattenuation and air trapping are apparent on chest computed tomography scans. The treatment of BO remains challenging, and many patients with BO develop end-stage pulmonary disease and do not survive.
Thyroid abnormalities
Thyroid-related complications in childhood cancer survivors include primary or central hypothyroidism, benign or malignant thyroid tumors, and (rarely) hyperthyroidism. Complications related to the thyroid gland are primarily seen in survivors who were treated with radiation to the head, nasopharynx, oropharynx, or total body or those who received radiation involving the cervical, supraclavicular, or mantle fields.
The risk for hypothyroidism or thyroid nodules is especially high for those treated with radiation doses in excess of 20 Gy. Strategies to decrease risk include shielding of the thyroid gland during radiation, use of lower-dose radiation (or elimination of radiation when possible), and avoidance of concurrent use of radiation and iodide-containing contrast materials. Central hypothyroidism related to cranial radiation is typically seen only in survivors who received radiation to the hypothalamic-pituitary axis at doses of 30 Gy or higher. The risk of central hypothyroidism in patients who receive lower doses of cranial radiation (such as is typically given in patients with ALL), is very low.
Gonadal function
Males
Abnormalities of both germ (Sertoli) cell and gonadal endocrine (Leydig cell) function can result from exposure to chemotherapy, radiation, or surgery in male cancer survivors. Germ cell-producing Sertoli cells are more sensitive to the cytotoxic effects of radiation and chemotherapy than the testosterone-producing Leydig cells. Thus, males may experience impaired germ cell function (oligospermia or azoospermia) without having evidence of gonadal endocrine dysfunction (testosterone insufficiency or deficiency).
Testicular radiation is known to result in decreased testicular volume, reduced or absent production of semen, and increased follicle-stimulating hormone. The effects are dose dependent, with potentially reversible azoospermia at doses of 1 to 3 Gy; azoospermia that is less likely to be reversible at doses of 3 to 6 Gy, and azoospermia that is typically irreversible at doses greater than 6 to 8 Gy. Data regarding semen production in males treated with testicular radiation alone before puberty are limited, although there is some evidence that germ cells in the prepubertal testes may be more radiosensitive than those in males who receive testicular radiation after puberty.
Chemotherapy agents with the potential to cause germ cell injury in males include alkylating agents (particularly busulfan, procarbazine, and mechlorethamine), heavy metals (such as platinum compounds), and nonclassic alkylators (such as procarbazine and dacarbazine). Many of these agents affect spermatogenesis in a dose-dependent manner. Effects on spermatogenesis may be reversible in up to 70% of males who receive cumulative cyclophosphamide doses lower than 7.5 gm/m 2 ; however, doses greater than 7.5 gm/m 2 may result in permanent azoospermia.
In male survivors of childhood cancer exposed to gonadotoxic chemotherapy, preservation of Leydig cell function in the setting of oligospermia or azoospermia is not uncommon. Similarly, Leydig cell injury is dose dependent, inversely related to age at treatment, and typically associated with radiation doses higher than those causing germ cell injury. Thus, males treated with higher doses of testicular radiation (>20 Gy) when prepubertal or peripubertal are at high risk of both infertility and testosterone deficiency or insufficiency, with resultant delayed or absent sexual maturation. Prepubertal males who receive fractionated doses of testicular radiation lower than 12 Gy are typically able to attain sexual maturity without intervention, although these males often experience compensated Leydig cell failure (normal testosterone with increased luteinizing hormone levels). Males in the adolescent and young adult age range at the time of testicular radiation are typically able to tolerate higher doses with lower risk for Leydig cell failure, when compared with younger males.
Surgical effects on male gonadal function include secondary hypogonadism in patients undergoing resection of brain tumors involving the hypothalamus or pituitary, impotence or retrograde ejaculation in patients undergoing partial or complete pelvic exenteration or bilateral retroperitoneal lymph node dissection, and hydrocele associated with nephrectomy.
Females
Two types of ovarian failure have been described in female childhood cancer survivors. Acute ovarian failure occurs when a female loses ovarian function during or shortly after completion of cancer treatment. Premature ovarian failure (POF) occurs when a female survivor retains ovarian function after childhood cancer treatment, but experiences menopause before reaching age 40 years. Increased risk for ovarian failure is associated with alkylating chemotherapy, older age at treatment, and pelvic or abdominal radiation. Conventional fractionated total body irradiation (TBI) is associated with ovarian failure in 50% of prepubertal females and nearly all females older than 10 years at exposure.
Ovarian failure associated with alkylating chemotherapy results in dose-dependent and age-dependent toxicity, with gonadal toxicity increasing with chronologic age. However, myeloablative doses of alkylating agents are strongly associated with permanent ovarian failure in females, regardless of age at exposure. There is increasing evidence supporting the risk for the development of POF in female survivors who do not develop amenorrhea after exposure to ovarian radiation or alkylating chemotherapy. In addition to infertility, POF is also associated with increased risks for cardiovascular disease, osteoporosis, and psychosexual dysfunction.
Surgical effects on female gonadal function include secondary hypogonadism in patients undergoing resection of brain tumors involving the hypothalamus or pituitary, hypogonadism requiring replacement therapy and associated infertility in females undergoing bilateral oophorectomy, and increased risk for POF associated with unilateral oophorectomy, as a result of reduced ovarian reserve.
Growth
Decreased linear growth is a common occurrence during therapy in children with cancer, but most children are able to experience catch-up growth after completion of therapy. In some instances, such as after cranial or spinal radiation exposure, short stature is permanent or even progressive.
Cranial irradiation can cause alterations in growth hormone function and secretion, resulting in short stature. The effects of cranial irradiation are age related, and children younger than 5 years at therapy are particularly susceptible. Severe growth retardation (standing height <5%) is seen in more than 50% of patients with brain tumors, when radiation doses exceed 30 Gy to the hypothalamus or pituitary gland. In ALL survivors, 24 Gy of cranial irradiation has been shown to result in a decrease in median height of about 5 to 10 cm.
Direct inhibition of vertebral growth by spinal irradiation also contributes to short stature. This change is seen most commonly in patients with brain tumors whose entire spinal columns have received doses in excess of 35 Gy. Children who are very young (<5 years) or are undergoing their adolescent growth spurt at the time of radiation therapy are at especially high risk.
The long-term effects of TBI on height in survivors of HCT are well described. After 10 Gy given as a single fraction, long-term, severe decreases in growth rates appear in most children. Fractionation of TBI seems to decrease growth retardation. The pathogenesis of short stature in children who receive conventional fractionated TBI is likely multifactorial and may be exacerbated by HCT-related complications such as graft-versus-host disease and its management.
Current approaches to cancer therapy in children include attempts to spare adverse effects on growth. Leukemia protocols are attempting to use high-dose methotrexate, cytosine arabinoside, or both, or intrathecal chemotherapy alone in lieu of radiation for CNS prophylaxis. Hyperfractionation schedules for radiation therapy and chemotherapy-only regimens are being implemented for treatment of brain tumors and as conditioning regimens for HCT. Whether these changes will permit long-term survivors to have normal growth remains to be seen.
Obesity
Obesity, defined as high body mass index (BMI, calculated as weight in kilograms divided by the square of height in meters, >30 kg/m 2 ), is well recognized among survivors of childhood ALL and brain tumors. Among patients with ALL, cranial radiation at 20 Gy or higher before the age of 5 years, African American race, Hispanic ethnicity, and use of antidepressant drugs (paroxetine) are associated with an increased risk of obesity. Among female survivors of brain tumors, young age at radiation to the hypothalamic-pituitary axis at doses exceeding 20 Gy is associated with obesity. Onset of obesity during adolescence or young adulthood is strongly associated with the subsequent development of adult-onset diabetes mellitus, hypertension, dyslipidemia, cardiovascular disease, osteoarthritis, and breast and colon cancer. High total fat percentage/lean body mass ratio is a reliable predictor of long-term morbidity. Percentage fat is significantly higher among recipients of cranial radiation, and even among individuals with normal BMI, and could explain the high prevalence of insulin resistance in this population. Leptin and adiponectin are secreted by the adipocytes and serve as part of a feedback loop between the hypothalamus, the adipocyte, and the gut with respect to hunger, satiety, and energy usage and storage. Adiponectin mirrors insulin resistance, whereas leptin reflects insulin resistance as well as anthropomorphic characteristics and body fat. Growth hormone deficiency seems to be a contributor to central obesity, insulin resistance, and dyslipidemia. Unrelated to BMI or physical activity, exposure to TBI or abdominal radiation is associated with an increased risk of diabetes.
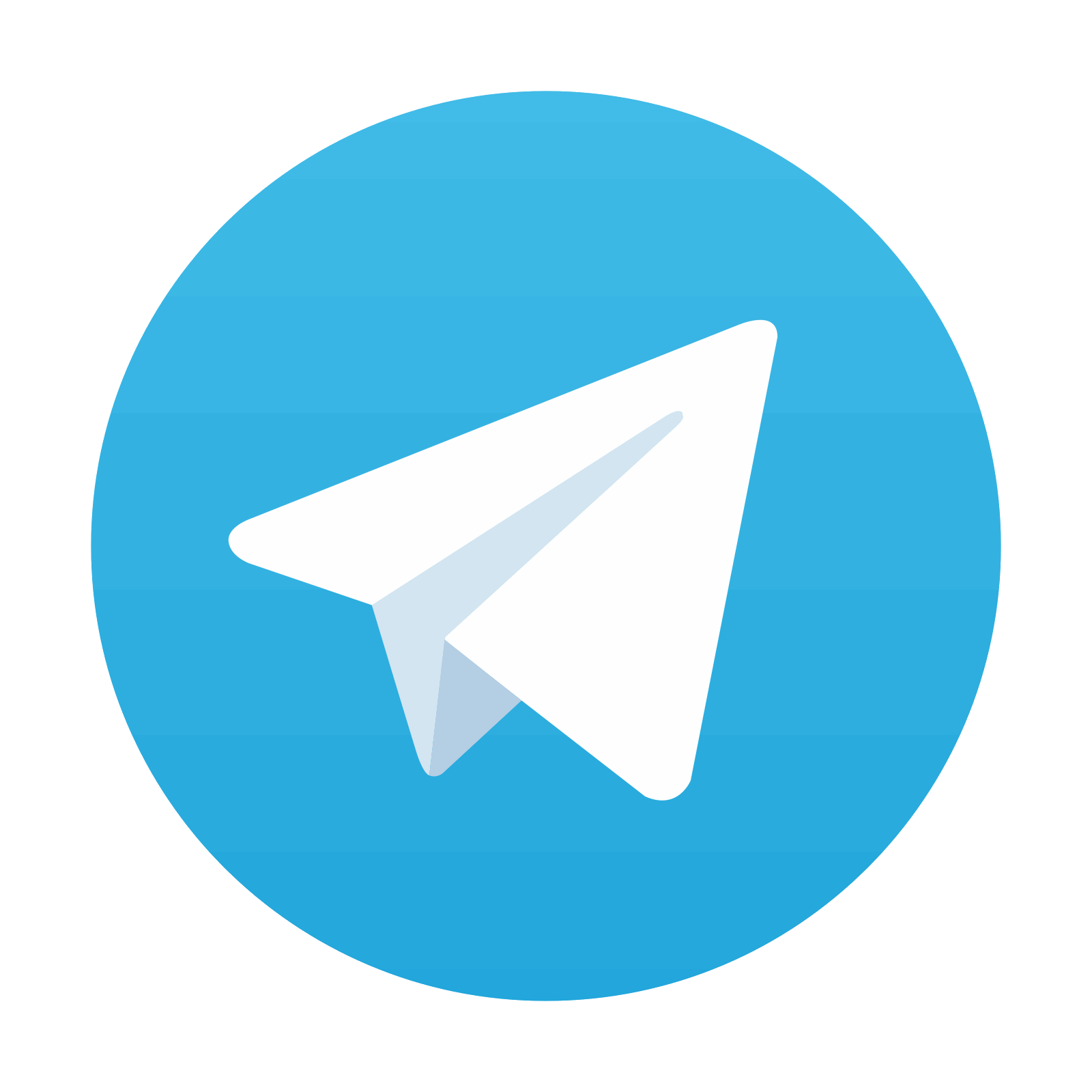
Stay updated, free articles. Join our Telegram channel

Full access? Get Clinical Tree
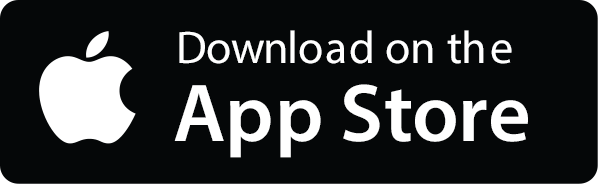
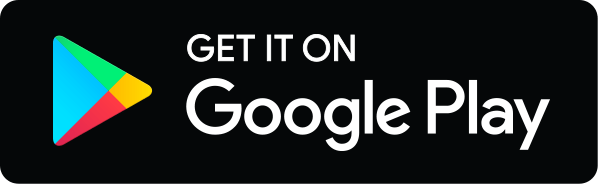