Objective
The clinical utility of tumor necrosis factor–related apoptosis–inducing ligand (TRAIL) in the treatment of established human malignancies is limited by the development of resistance to TRAIL. We hypothesized that knockdown of map-kinase activating death domain containing protein (MADD), a TRAIL-resistance factor, may overcome TRAIL resistance in ovarian cancer cells.
Study Design
MADD expression in resected ovarian cancer specimens and cell lines was quantified with the use of polymerase chain reaction. Sensitivity of ovarian cancer cell lines to TRAIL, with or without MADD knockdown, was assessed.
Results
MADD is expressed at relatively higher levels in human malignant ovarian cancer tissues and cell lines, compared with normal ovarian tissues. The cell lines OVCA429 and OVCAR3 were susceptible, and cell lines CAOV-3 and SKOV-3 were resistant to TRAIL. MADD knockdown in CAOV-3 cells, but not in SKOV-3 cells, conferred TRAIL sensitivity. Knockdown of cellular Fas-associated death domain–like interleukin-1 beta-converting enzyme–inhibitory protein (c-FLIP) in SKOV-3 cells increased spontaneous and TRAIL-induced apoptosis, which was further increased on MADD knockdown.
Conclusion
MADD/c-FLIP L knockdown can render TRAIL-resistant ovarian cancer cells susceptible to TRAIL.
Ovarian cancer is a very common malignancy, with an estimated incidence of 21,880 new cases in 2010. Ovarian cancer is the most fatal gynecologic malignancy and the fifth leading cause of cancer-related deaths in women, with an estimated 13,850 deaths annually in the United States. When possible, the best outcomes for advanced disease are obtained with a combination of surgery and neoadjuvant or adjuvant chemotherapy. Initial response rates to chemotherapy vary from 60-80%; however, most patients with persistent or recurrent disease that requires chronic chemotherapy will experience drug resistance and eventually experience progression of disease. For these patients, new therapeutic strategies are needed.
Tumor necrosis factor–related apoptosis–inducing ligand (TRAIL) is a member of the tumor necrosis factor super family of cell death–inducing ligands. TRAIL can induce programmed cell death or apoptosis in a variety of tumor cell lines and not in normal or nontransformed cells. TRAIL induces apoptosis by activating the extrinsic apoptosis pathway by interacting with the death receptors (DRs), DR4 and DR5. TRAIL binding to DRs induces receptor oligomerization that results in the formation of a death-inducing signaling complex (DISC), which contains the Fas-associated death domain (FADD) and pro–caspase-8 and leads to the activation of caspase-8. Activation of caspase-8 activates caspase-3 and induces apoptosis. Alternatively, activated caspase-8 can cleave Bid, a pro–apoptotic protein, that results in truncated Bid that can translocate to mitochondria and initiate intrinsic apoptosis.
Although higher levels of expression of DRs can render cells more susceptible to TRAIL induced apoptosis, increased levels of expression of decoy DRs (DcR1 and DcR2) and anti-apoptotic proteins (FADD-like interleukin-1 beta-converting enzyme–inhibitory protein [c-FLIP], inhibitor of apoptosis protein [x-IAP], survivin, B-cell lymphoma 2 [Bcl2], myeloid cell leukemia sequence 1 [MCL1], and map-kinase–activating death domain–containing protein [MADD]) can confer significant resistance to TRAIL-induced apoptosis. DcR1 and DcR2 lack a functional death domain and compete with DR4 and DR5 for cell surface TRAIL binding but cannot transmit the apoptotic signal. The anti–apoptotic protein c-FLIP, which is an inhibitor of death ligand–induced apoptosis, comes in 2 isoforms: a long form (c-FLIP L ) and a short form (c-FLIP S ). The c-FLIP L , a 55-kd protein, contains 2 death-effector domains and a caspase-like domain, whereas c-FLIP S , a 26-kd protein consists only of 2 death-effector domains. Both isoforms are recruited to the DISC through binding to FADD, and they prevent procaspase-8 recruitment and thus block DR-mediated apoptosis. The c-FLIP L is over-expressed in a number of different tumors and often is associated with TRAIL resistance. The Bcl2 family proteins (Bcl2 and MCL1) act as mitochondrial intracellular checkpoints and resist apoptosis. Execution of the apoptotic program is also controlled by IAP, x-IAP, and survivin, which selectively can bind and inhibit caspases-3, -7, and -9 and thereby prevent cell death in response to multiple stimuli.
We previously identified and characterized MADD as a TRAIL resistance factor. MADD is an isoform of the insulinoma-glucagonoma 20 ( IG20 ) gene and is expressed at very low levels in most healthy tissues but is expressed at significantly higher levels in many human tumors and tumor cell lines. Knockdown of MADD expression results in enhanced spontaneous and TRAIL-induced apoptosis in cells that are derived from cervical cancer, neuroblastoma, and thyroid cancer. Further, expression of exogenous MADD, not other IG20 splice variants, in the absence of all endogenous isoforms, can rescue these cells from undergoing apoptosis. These findings indicated that only MADD isoform of the IG20 gene can promote cancer cell survival. The current study was initiated to determine whether ovarian cancer tissues and cells express MADD at higher levels and whether it contributes to TRAIL resistance in ovarian cancer cells.
Materials and Methods
Cell culture
OVCA429, OVCAR-3, CAOV-3, and SKOV-3 ovarian cancer cells were purchased from American Type Culture Collection (Manassas, VA) and cultured according their instructions. Briefly, OVCA429 and SKOV-3 cells were cultured in RPMI 1640 (Invitrogen Life Technologies, Carlsbad, CA) supplemented with 10% fetal bovine serum. OVCAR-3 cells were cultured in RPMI 1640 that contained 20% fetal bovine serum plus 0.01 mg/mL bovine insulin. CAOV-3 cells were cultured in DMEM (Invitrogen Life Technologies) with 10% fetal bovine serum. Culture media were also supplemented with 100 units/mL of penicillin and 100 μg/mL of streptomycin. The cell lines were maintained at 37°C in a humidified chamber with 5% CO 2 .
Antibodies
Antibody to FLIP L was purchased from Enzo Life Science Inc (Farmingdale, NY). The preparation of anti-MADD exon 13L–specific antibodies has been reported earlier. The goat anti-mouse immunoglobulin G1 peroxidase-conjugated secondary antibody was obtained from Caltag Laboratories (Burlingame, CA); the anti-rabbit peroxidase-conjugated polyclonal secondary antibody was purchased from GE Healthcare (Piscataway, NJ). Antibodies against DR4, DR5, DcR1, and DcR2 were purchased from Ebioscience (San Diego, CA).
Tissue samples and RNA preparation
Snap-frozen normal, benign, and malignant ovarian cancer tissues ( Supplementary Table 1 ) were collected following the protocol that was approved by the institutional review board of the University of Illinois at Chicago. Snap frozen tissue samples were obtained from Cooperative Human Tissue Network (Midwestern Division, Ohio State University, Columbus, OH). Samples 2-9 were normal, sample 10 was benign, and samples 11-19 were malignant. Frozen tissues (100 mg) were immersed in liquid nitrogen and ground into fine powder and solubilized in reagent (TRIZOL; Invitrogen Life Technologies). Total RNA was extracted from ovarian tissues or from ovarian cancer cells according to the manufacturer’s instructions.
Design of small inhibitory RNAs
The nucleotide sequences of various short hairpin RNAs (shRNAs) that were used in this study are shown in Supplementary Table 2 . The shRNAs targeting exon 15 of MADD (MID) and the scrambled RNA (SCR) (negative control) are identical to those previously described. The small interfering RNA (siRNA) targeting c-FLIP was designed with the OligoEngine Workstation 2 (OligoEngine, Inc, Seattle, WA). These siRNAs were screened in OVCA429 cells, and the most efficient was used to construct the cFLIP- shRNA lentivirus.
Plasmid construction
The siRNAs were cloned into the pSUPER vector with the use of BgI II and HindIII sites to generate pSup-cFLIP plasmids. The shRNA cassettes (including the H1 RNA promoter and the shRNA) were excised from pSup-cFLIP using XbaI and ClaI sites and were ligated into the pNL-SIN-CMV-GFP vector to generate cFLIP lentivirus constructs (c-FLIP i ). The pcTat, pcRev, and pHIT/G were gifts from Dr B.R. Cullen (Duke University Medical Center, Durham, NC) and Dr T.J. Hope (Northwestern University, Department of Cell & Molecular Biology, Evanston, IL).
Preparation of lentivirus stocks
Lentivirus stocks were prepared as described previously. Briefly, subconfluent 293FT cells that had been grown in 100-mm plates were co-transfected with 10.8 μg of lentivirus vector (contained either SCR, MID, or cFLIP shRNA), 1 μg pcRev, 1 μg of pcTat, and 0.5 μg of pHIT/G using calcium phosphate. Culture medium was replaced after 16 hours, and the supernatant was harvested at 40 hours and filtered with a 0.22-mm filter. The optimal viral titer for each cell type was determined as the least amount of viral supernatant that was required to transduce at least 80% of target cells without apparent cytotoxicity.
Quantitative real-time polymerase chain reaction (q-RT-PCR)
q-RT-PCR was carried out with TaqMan 1-step RT-PCR Master Mix reagents (Applied Biosystems, Carlsbad, CA), according to the manufacturer’s protocol. Briefly, 1 μg RNA was added into 25 μL of reaction mixture that contained 12.5 μL of Master mix, 0.75 μL of 40X MultiScribe, RNase inhibitor mix (Applied Biosystems, Carlsbad, CA), 0.75 nmol/L each primer, and 0.25 nmol/L probe. The primer and probe sequences are listed in Supplementary Table 3 . The RT-PCR reaction was performed in the following manner: reverse transcription at 48°C for 30 minutes, denaturation at 95°C for 10 minutes, followed by 40 cycles of denaturation at 95°C for 15 seconds, annealing at 60°C for 1 minutes. TaqMan 18s ribosomal RNA (rRNA) control reagents (Applied Biosystems) were used for amplification of the endogenous control. Delta-delta threshold cycle method was used to calculate the relative expression levels of tested genes vs to 18s rRNA. Data were analyzed using q-gene program.
Reverse transcription–polymerase chain reaction
We used 1 μg of RNA for reverse transcription-polymerase chain reaction (RT-PCR) using the Super-Script III One-Step RT-PCR system (Invitrogen Life Technologies). Briefly, the complementary DNAs were synthesized at 50°C for 30 minutes followed by incubation at 94°C for 2 minutes. Subsequently, 30 cycles of PCR were carried out with denaturation at 94°C for 50 seconds, annealing at 55°C for 50 seconds, and extension at 72°C for 1 minute, followed by a final incubation at 72°C for 7 minutes. For amplifying regions flanking exons 13L and 16, F-1 and B-1 primer pairs (5′-CGG GAC TCT GAC TCC GAA CCT AC-3′ and 5′-GCG GTT CAG CTT GCT CAG GAC-3′, respectively) were used. Glyceraldehyde-phosphate dehydrogenase that was used as a loading control was amplified using primers that have been previously published and it served as a loading control. The PCR products were then separated on a 5% polyacrylamide gel.
Immunofluorescence staining
Total of 3 × 10 5 cells were placed into 60-mm culture dishes with cover glasses and cultured overnight. The cells were fixed with acetone and permeabilized with 0.01% Triton X-100 (Sigma-Aldrich, St. Louis, MO) and subsequently blocked with 1% bovine serum albumin for 30 minutes at RT. Cells were incubated overnight with 13L antibody at 4°C, washed, and probed with a biotinylated anti-rabbit antibody (Caltag Laboratories) and streptavidin-FITC (BD Pharmingen, San Diego, CA). Normal rabbit anti-serum was used as a negative control. The image was visualized and captured with a confocal microscope (LSM 510 META; Carl Zeiss, Jena, Germany).
Flow cytometry to detect DRs and DcRs
Cell surface expression of DRs/DcRs was evaluated by flow cytometry as previously described. For each cell line, 1 × 10 6 cells were incubated with 5.0 μg of the appropriate anti-DR/DcR antibody (goat polyclonal antisera for DR4, DR5, and DcR1; rabbit antiserum for DcR2) or control (goat or rabbit immunoglobulin G, respectively) for 45 minutes. Cells were then washed with phosphate-buffered saline solution and incubated for 45 minutes with 2.0 μg of the appropriate FITC-conjugated secondary antibody (donkey anti-goat or goat anti-rabbit immunoglobulin G, respectively; Jackson ImmunoResearch Laboratories, West Grove, PA). Cells were then washed, fixed with 1% formaldehyde–phosphate-buffered saline solution, and analyzed with a flow cytometer (CyAn ADP; Beckman Coulter Inc, Brea, CA).
Apoptosis assay
Phycoerythrin conjugated antibody against activated caspase-3 (CloneC92-605) was used to label caspase-3 according to the manufacturer’s instructions (BD Pharmingen); samples were analyzed by flow cytometry. Briefly, ovarian cancer cells (1∼2 × 10 5 ) were plated into 6-well plates. Twenty-four hours later, cells were treated overnight with different shRNA-expressing lentiviruses, washed, and replenished with fresh warm medium. Seventy-two hours after transduction, the cells were either treated or untreated with 25 ng/mL or 50 ng/mL of TRAIL for 4 hours, trypsinized, and washed twice with cold phosphate-buffered saline solution. The cells were resuspended in BD Cytofix/Cytoperm solution (BD Pharmingen) and incubated on ice for 20 minutes. The cells were washed with 1X BD Perm/Wash buffer (BD Pharmingen) and labeled with phycoerythrin-conjugated anti–activated caspase-3 antibody at room temperature for 30 minutes before analysis by flow cytometry (Beckman Coulter Inc).
Western blot analysis
Different shRNA-expressing, lentivirus-transduced ovarian cancer cells were trypsinized and washed with phosphate-buffered saline solution and lysed at 0°C for 30 minutes in a lysis buffer (20 mmol/L Hepes, pH 7.4, 2 mmol/L EGTA, 420 mmol/L NaCL, 1% Triton X-100, 10% glycerol, 1 mmol/L dithiothreitol, 1 mmol/L phenylmethylsulfonyl fluoride, 10 μg/mL leupeptin, 10 μ/mL aprotinin, 1 mmol/L Na 3 VO 4 , and 5 mmol/L NaF). The protein content was determined with a dye-binding microassay (Bio-Rad Laboratories, Hercules, CA); after the samples were boiled for 5 minutes in a 1X sodium dodecylsulfate protein sample buffer, 50 μg of protein per lane was loaded and separated on 10% sodium dodecylsulfate–polyacrylamide gel. The proteins were blotted onto Hybond ECL membranes (GE Healthcare Bio-Sciences Corp, Piscataway, NJ), blocked with Tris-buffered saline solution with Tween-20 (10 mmol/L Tris-HCl, pH 7.4, 150 mmol/L NaCl, 0.1% Tween-20) that contained 5% milk. Blots were incubated overnight with antibodies against MADD protein or c-FLIP diluted in Tris-buffered saline solution with Tween-20 that contained 5% bovine serum albumin. The membranes were then washed, incubated with an anti-rabbit secondary antibody (1:5000) in a blocking buffer for 1 hour, and washed repeatedly. Proteins were detected with an enhanced chemiluminescence plus Western blotting detection system (Amersham International PLC, Buckinghamshire, UK). Horseradish peroxidase labeled anti–beta-actin (Sigma-Aldrich) antibodies were used to detect beta-actin, which served as a loading control.
Statistical analysis
All results are expressed as mean ± SE. The Student t test was used to determine the probability values with Microsoft Excel Software (version 2003; Microsoft Corporation, Redmond, WA). Probability values ≤ .05 were considered significant.
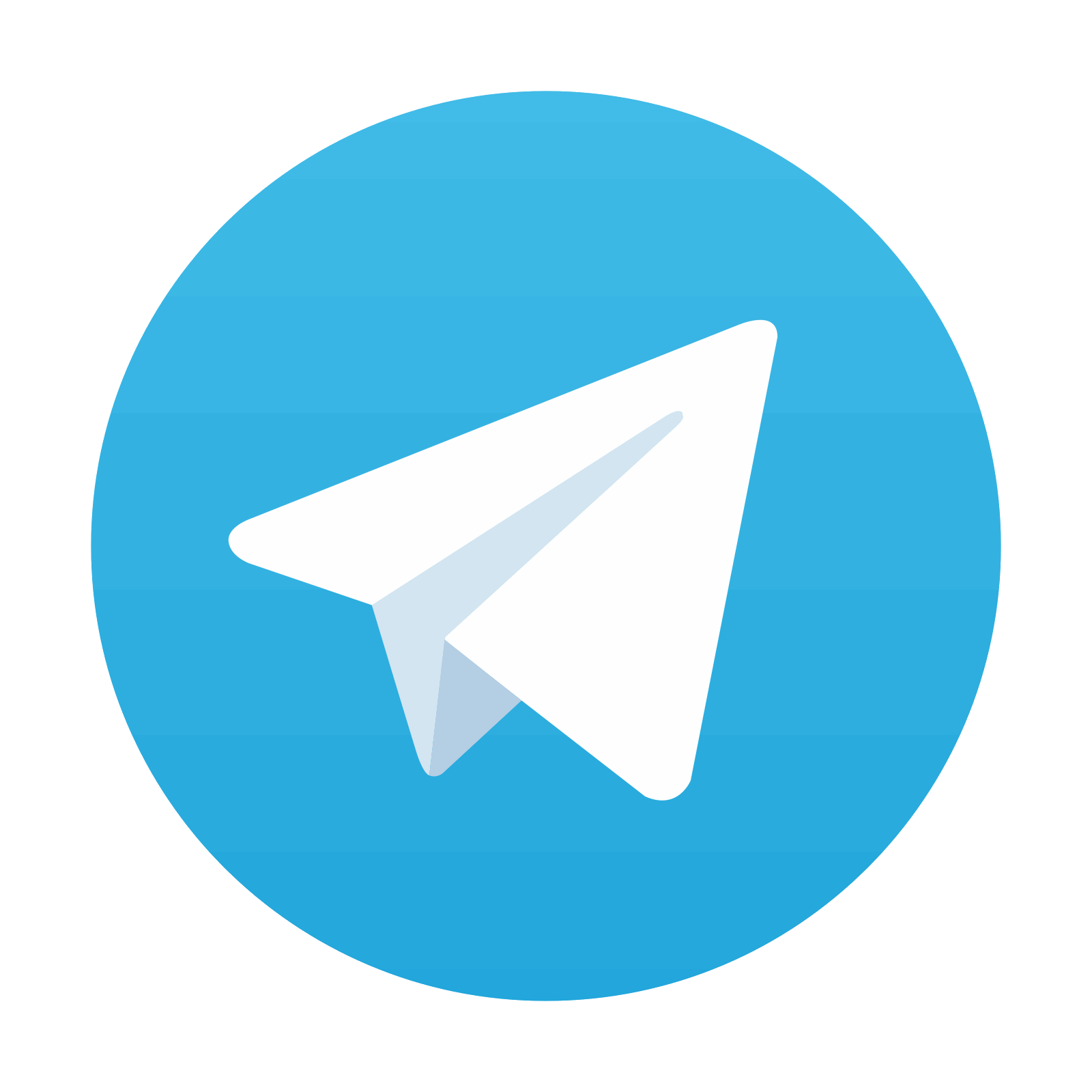
Stay updated, free articles. Join our Telegram channel

Full access? Get Clinical Tree
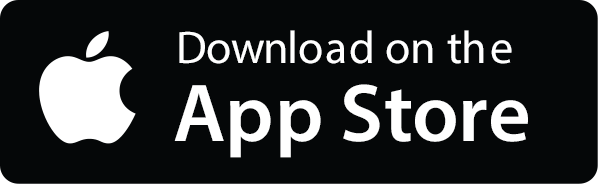
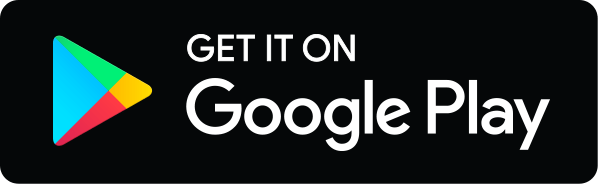