(1)
Pediatric Neurosurgical Departement, Hôpital Necker-Enfants Malades, APHP, 149, Rue de Sèvres, Paris, 75743, France
(2)
Genetic Department, National Reference Centre for Congenital Bone Diseases, Hôpital Necker-Enfants Malades, INSERM U781, Paris, France
(3)
Otorhinolaryngology Department, Hôpital Necker-Enfants Malades, Paris, France
(4)
Unité de ventilation non-invasive et du sommeil de l’enfant, INSERM U 955, Hôpital Necker-Enfants Malades, Paris, France
Abstract
The natural history of CVJ stenosis in achondroplastic children is only partially known. Achondroplastic children have a foramen magnum that is significatively smaller at birth, and it does not follow the normal growth during the first 18 months of life, leading to CVJ stenosis and, for the most severe of them, to neurological and developmental impairment and delay and even sudden death due to cervicomedullary narrowing. We reviewed our experience based on 37 patients operated for cervicomedullary decompression between 1970 and 2010 and performed a literature review. The indication for surgery should be taken on very strict clinical and radiological parameters as well as sleep studies. Under those criteria, surgical decompression of CVJ leads to neurological and developmental improvement, despite non-negligible mortality and morbidity.
Keywords
AchondroplasiaCranio-cervical junctionSudden deathForamen magnum decompressionIntroduction
Achondroplasia is the most common form of short-limb dwarfism. Its prevalence is estimated at 1/15,000 newborns per year. This condition is caused by a mutation in FGFR3 gene. In more than 99 % of the cases, the same amino acid substitution (G380R) is found, resulting in a constitutive activation of the receptor independent from its ligands [33, 38, 40]. FGFR3 plays a key role in the ossification processes, and its alteration has multiple effects in craniofacial and vertebral development especially at the level of the craniovertebral junction (CVJ) [7, 12].
Normal Bone Growth and Embryology of CVJ [22]
The normal growth of CVJ begins around the end of the fourth week of development, when 42 somites originate from the mesenchyma under notochord’s mediation and regulation. Among those somites that will give rise to the spine, the 4 occipital somites and the first 2 cervical somites will take part in the formation of the CVJ [20, 21].
At the spinal level, each somite will divide in three parts: a dorsal dermatome, a cranial myotome and a sclerotome. The sclerotome surrounds the notochord, which is central and remains the element that regulates the whole process, then appears a central cleft in each sclerotome (called “Ebner’s cleft”) dividing each sclerotome into 2 halves, 1 superior and 1 inferior. The gap between the 2 halves will be invaded by cells that will give the intervertebral disc. Progressively, the superior half of one sclerotome will merge with the inferior half of the sclerotome that is just above, leading to the formation of the spine.
At the level of the CVJ, the process is quite different. The first three occipital sclerotomes will not divide. The first two occipital sclerotomes will give the basi-occiput. The third one will form the jugular tubercles.
The fourth occipital sclerotome and the first two cervical sclerotomes will divide into three parts called hypocentrum, centrum and neural arch.
The fourth occipital sclerotome is of particular importance and called “proatlas” because of its crucial role in the formation of C1.
Concerning the proatlas, the hypocentrum will give the anterior tubercles of the clivus; the centrum will generate the apical ligament and the apex of the odontoid process. The neural arch will itself divide into one rostral contingent from which the occipital condyles, the alar and cruciform ligaments will rise and into one caudal contingent giving the posterior arch and the lateral masses of C1.
The first cervical sclerotome will give the anterior arch of C1 (hypocentrum), the odontoid process (centrum) and the inferior aspect of the posterior arch of C1 (neural arch).
At last, from the second cervical sclerotome will rise the vertebral body (centrum) and posterior arch and articular facets (neural arch) of C2.
All those elements are summarised in Table 1 and Fig. 1.
Table 1
Summary of the four occipital and first two cervical sclerotomes segmentation and evolution
Sclerotome |
Division |
Subdivision |
Formation |
---|---|---|---|
1st occipital |
No |
No |
Basi-occiput |
2nd occipital |
No |
No |
Basi-occiput |
3rd occipital |
No |
No |
Jugular tubercles |
4th occipital “proatlas” |
Hypocentrum |
No |
Anterior tubercles of clivus |
Centrum |
No |
Apical ligament, apex of odontoid | |
Neural arch |
Rostral |
Occipital condyles, alar and cruciform ligaments | |
Caudal |
Posterior arch and lateral masses of C1 | ||
1st cervical |
Hypocentrum |
No |
Anterior arch of C1 |
Centrum |
No |
Odontoid process | |
Neural arch |
No |
Postero-inferior arch of C1 | |
2nd cervical |
Hypocentrum |
– |
– |
Centrum |
No |
Vertebral body of C2 | |
Neural arch |
No |
Posterior arch and articular facets of C2 |
Craniovertebral Junction Development in Achondroplasia
Bone Growth and Embryology of the CVJ
Intra-membranous and Endochondral Ossification
There are two mechanisms of ossification at the level of the skull: intra-membranous and endochondral (Fig. 1). During the intra-membranous ossification, the bony tissue will develop by direct differentiation of mesenchymal cells that lie in the mesenchymatous matrix. Those cells will differentiate into osteoblasts and build a new, rich, osseous tissue which will grow.
In the endochondral ossification, mesenchymal cells build a cartilaginous matrix that will secondary become osseous through different stages.
Ossification of the Skull
Ossification of the skull vault and the face is principally intra-membranous (Fig. 1). There are numerous sites (called the ossification points) situated in the mesenchymal matrix that lie between the dura mater and the skin, where the ossification process begins. Bone growth is due to addition of numerous plates of ossification that grow in a centrifugal way from the ossification points and tend to merge together. The frontier between two plates represents a suture where remains a very thin band of mesenchymal tissue.
Ossification of the skull base is principally endochondral (Fig. 2). Several regions called synchondrosis can be described in the skull base (spheno-occipital synchondrosis, for example). In those regions, some mesenchymal cells differentiate into chondrocytes and constitute a cartilaginous plate that is central, whereas cells that are located at the periphery differentiate into primitive osteoprogenitors and begin the ossification process. Bone growth is due to the very active production of the cartilaginous plate. The principal receptor that is implicated in achondroplasia, FGFR3, is expressed quasi exclusively in the proliferative and prehypertrophic zone of the cartilaginous plate [4].
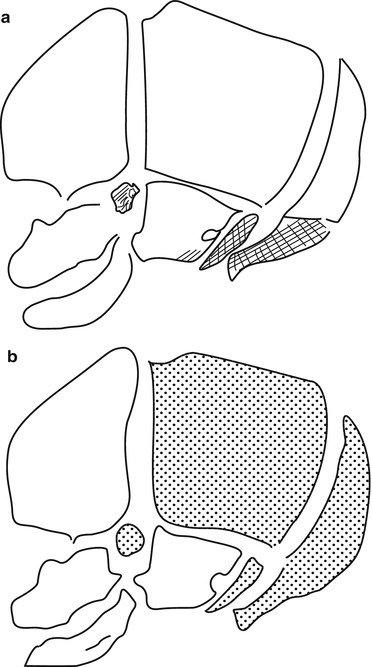
Fig. 2
Bone growth and embryology of the skull. (a) Membranous ossification (white) and endochondral ossification (shaded). (b) Neural crest origin (white) and mesoderm (dotted) (Adapted from Helms et al. [11])
CVJ Bone Growth in Achondroplastic Children
Molecular and Histologic Characteristics of Achondroplasia
Achondroplasia is the most frequent of the diseases caused by different mutations of the gene that codes for the fibroblast growth factor receptor 3 (FGFR3) [33, 38]. Mutations in FGFR3 can lead to many other forms of chondrodysplasia such as the hypochondroplasia or thanatophoric dysplasia [13] or SADDAN and even some craniostenosis such as the Muenke-Lajeunie syndrome [18, 25, 30, 35] or the Crouzon syndrome with acanthosis nigricans [24, 32]. Even if many progresses have been made since the discovery of those mutations in early 1990s, the exact molecular consequences of the mutation and the exact mechanism for each disease remain a matter of debate. Mutations of FGFR3 in those diseases have been shown to cause a gain of function of FGFR3, the receptor becoming constitutively activated. At the end, FGFR3 is thought to be one of the most important of bone growth regulators. Its activation results in an inhibition of bone growth, by altering the proliferation and the differentiation of the chondrocytes that are active in the cartilaginous growth plate of endochondral sites of ossification. With the receptor being constitutively activated in achondroplasia, this leads to a major dysfunction of the cartilaginous growth plate and bone growth [14].
Those elements are confirmed by the histologic characteristics of the cartilaginous growth plate of achondroplastic children. The chondrocytes that normally constitute the growth plate show a very poor organisation in those patients. The normal organisation in cells columns separated by collagenous septa is hardly outlined in achondroplastic subjects. Furthermore, the collagenous septa are hypertrophic, leading sometimes to the constitution of true zones of fibrosis in the cartilaginous plate [39].
Endochondral ossification is the principal mechanism of ossification occurring in long bones, in the spine and in the skull base. This explains why achondroplastic children suffer from dwarfism and lumbar stenosis and may have signs of cervicomedullary compression due to a too narrow CVJ.
At the level of the skull base, the spheno-occipital synchondrosis is particularly affected. In fact it is typically absent in achondroplastic children and replaced by ossified tissue. Such alteration is associated to an abnormal clivus that can be observed at CT or MR imaging (Fig. 3). The clival bone is, in fact, reduced in size, especially in the anterior-posterior dimension, thus participating to the midface retrusion found in these children.
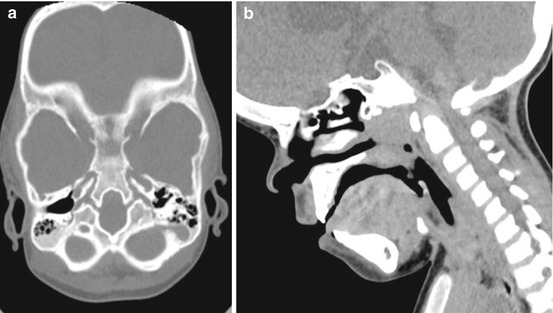
Fig. 3
Craniovertebral junction in an achondroplastic child. Axial (a) and sagittal (b) reconstruction showing the abnormal shape of the clival bone
Foramen Magnum (FM) Stenosis in Achondroplasia
The FM of achondroplastic children is known to be small [5, 19], but a quantification of the extent of this stenosis has been lacking until the 1980s. In two major studies published in 1985 and 1989 [8, 10], Hecht et al. performed computerised tomography studies of the foramen magnum in achondroplastic and non-achondroplastic children. They compared the sagittal and transversal measures of the FM in achondroplasia with those in the general population and then the same measures in symptomatic (i.e. achondroplastic children that presented signs of cervicomedullary compression) and asymptomatic achondroplastic children. The authors could demonstrate (1) that all achondroplastic children have a foramen magnum that is significantly smaller at birth, both in the transversal and sagittal diameters; (2) that the FM in achondroplastic children does not follow the outstanding growth during the first 18 months of life as the FM of normal children does; and (3) that in adulthood the FM is on average equivalent to the one of a normal newborn on the transversal diameter and to the one of a normal 2-year-old child on the sagittal diameter, meaning that the stenosis is greater along the transverse dimension. They could also show that (4) symptomatic achondroplastic children have a significantly smaller FM than asymptomatic achondroplastic children.
Actually, when the measures of achondroplastic and normal children are reported together, the curves obtained by Hecht et al. showed that whatever the clinical status is, the FM size of achondroplastic children is at least within -5SD of the mean for the transverse measure and within – 4SD for the sagittal dimension. Moreover, the authors proved that symptomatic patients had significantly smaller FM than asymptomatic ones, both transversally and sagittally (p < 0.0005 for each dimension). This last element confirms that the higher the stenosis is, the worse the clinical consequences are.
The results of the studies by Hecht et al. are confirmed by the work of Keiper et al. [16], who followed 11 initially asymptomatic achondroplastic children prospectively between 1988 and 1995. At the time of first MRI evaluation (mean age 23 weeks), the mean values of the FM were 7.9 mm (6–10 mm) for the sagittal dimension and 9.09 mm for the transverse dimension (6–12 mm). Out of these 11 patients, 4 needed to be operated for CVJ stenosis. They had a smaller mean value of 6.7 mm for the sagittal dimension and of 7.5 mm for the transverse dimension.
In our experience based on the retrospective analysis of 37 achondroplastic children that needed surgical cervicomedullary decompression between 1981 and 2010 (mean age at surgery 29.3 months), we reached the same conclusions. The mean values of FM measured on CT scan and/or on MRI were 6,01 mm for the sagittal dimension and 9.3 mm for the transversal one (Table 2). Those values are consistent with those presented by Keiper et al. [16] and slightly inferior to those described by Hecht et al. [8]. One explanation for this observation might be that in our evaluation as well as in Keiper’s one, the measures were performed using MRI, whereas in Hecht’s study the measures were obtained by CT scan. Nevertheless, all measures in achondroplastic children were far below those observed in unaffected children.
Table 2
Characteristics of the 37 patients operated between 1981 and 2010 at Necker-Enfants Malades, Paris
Child |
Age at surgery (months) |
Sagittal measurement before surgery (mm) |
Transverse measurement before surgery (mm) |
Sagittal measurement after surgery (mm) |
Transverse measurement after surgery (mm) |
---|---|---|---|---|---|
C.Z. |
18 |
7 |
5 |
9 |
10 |
E.F.S. |
7 |
4 |
9 |
7 |
9 |
M.M. |
180 |
10 |
12 |
12 |
12 |
R.A. |
20 |
8 |
10 |
8 |
10 |
K.S. |
7 |
4 |
7 |
7 |
9 |
G.K. |
13 |
– |
– |
9 |
11 |
W.L. |
132 |
– |
– |
7 |
– |
R.S. |
18 |
5 |
8 |
9 |
7 |
L.I. |
16 |
3 |
8 |
9 |
9 |
M.E. |
5 |
6 |
9 |
9 |
8 |
M.S. |
20 |
5 |
10 |
7 |
9 |
A.O. |
19 |
5 |
– |
8 |
10 |
Z.S. |
17 |
6 |
9 |
8 |
9 |
V.J. |
17 |
6 |
9 |
8 |
10 |
W.J. |
20 |
3 |
10 |
7 |
8 |
R.T. |
6 |
– |
– |
– |
– |
D.M. |
8 |
7 |
8 |
– |
– |
A.A. |
19 |
– |
– |
7 |
9 |
H.P.H. |
13 |
7 |
9 |
10 |
10 |
B.O. |
16
![]() Stay updated, free articles. Join our Telegram channel![]() Full access? Get Clinical Tree![]() ![]() ![]() |