Valproic acid, or valproate (VPA), has been used in the treatment of epilepsy for more than 50 years (1). During this time, it became one of the major antiepileptic drugs (AEDs), mainly because it was the first (and until the 1990s, the only) drug with a broad spectrum of activity against different seizure types, and because of its relatively low sedative effect. In addition to being a highly effective treatment for several primarily generalized seizure types, such as absences, myoclonic seizures, and generalized tonic–clonic seizures, VPA also was found to have some effectiveness in the treatment of partial seizures, Lennox–Gastaut syndrome, infantile spasms, neonatal seizures, and febrile seizures. Thus, VPA became an important drug for use by pediatric neurologists. In addition to its place in the treatment of epilepsy, VPA has been used to treat affective disorders in psychiatry and for the prophylaxis of migraine headaches. Effectiveness in the treatment of Sydenham’s chorea also has been suggested (2), indications that will not be included in this chapter.
CHEMISTRY, ANIMAL PHARMACOLOGY, AND MECHANISM OF ACTION
VPA (Figure 66.1) is a colorless liquid of molecular weight (MW) 144.21 with low solubility in water. Sodium valproate (MW 166.19) is a highly water-soluble and highly hygroscopic, white, crystalline material. Sodium hydrogen divalproate (divalproex sodium) is a mixture of equal parts of VPA and sodium valproate. Being a short-chain branched fatty acid, VPA differs chemically from all other known AEDs. The anticonvulsant effect of VPA was discovered serendipitously when it was used as a solvent for compounds being tested in an animal model of seizures (3). The antiepileptic activity of VPA was well demonstrated in several animal models (4–6). Valproate protects against maximal electroshock (MES)-induced seizures; seizures induced chemically by pentylenetetrazol (PTZ), bicuculline, glutamic acid, kainic acid, strychnine, ouabain, nicotine, and intramuscular penicillin; and seizures induced by kindling (7). This broad spectrum of efficacy of VPA in animal models suggests that it is effective in both preventing the spread and lowering the threshold of seizures, and this is consistent with the broad spectrum of antiepileptic activity of VPA observed in humans.
Extensive studies have been carried out to elucidate the mechanism of action of VPA. Several effects of VPA have been demonstrated at the cellular level, but the precise mechanism underlying the antiepileptic effect of VPA has not been identified; more than one mechanism may be involved, and none of the identified actions has been widely accepted as the predominant relevant mechanism (8). VPA raises brain levels of the inhibitory neurotransmitter gamma-aminobutyric acid (GABA) (5,9). This increase may be due to the inhibition of GABA-transaminase, the first step in GABA deactivation (10); inhibition of succinic semialdehyde dehydrogenase, the second step in GABA deactivation (11); or an increase in activity of glutamic acid decarboxylase, an enzyme involved in the synthesis of GABA (9), or downregulation of GABA transporter proteins (12). However, because it occurs at much higher-than-usual therapeutic doses and because its time course lags behind the anticonvulsant effect (13), elevation of GABA is unlikely to be the predominant mechanism of the antiepileptic effect of VPA. In addition to its effect on tissue levels of GABA, VPA reduces sustained repetitive high-frequency firing by blocking voltage-sensitive sodium channels (14) or by activating calcium-dependent potassium conductance (15). It was shown in mice that VPA can decrease brain levels of the excitatory amino acid aspartate (16), as well as decrease expression of the glutamate transporter-1 in the rat hippocampus with the net effect of increasing tissue GABA (12). It is not known to what extent these actions contribute to the clinical effect of VPA.
BIOTRANSFORMATION, PHARMACOKINETICS, AND INTERACTIONS
Biotransformation
Biotransformation of VPA in humans includes glucuronidation of VPA itself, beta-oxidation, hydroxylation, ketone formation, and desaturation (Figure 66.2). Both beta-oxidation and desaturation can result in the formation of double bonds. By far the most abundant metabolites are VPA glucuronide and 3-oxo-VPA that comprises about 40% and 33%, respectively, of the urinary excretion of a VPA dose (18). Two desaturated metabolites of VPA, 2-ene-VPA and 4-ene-VPA, have anticonvulsant activity that is similar in potency to that of the parent drug (19). Because there is delayed but significant accumulation of 2-ene-VPA in the brain and it is cleared more slowly than VPA itself (20), the formation of 2-ene-VPA may provide a possible explanation for the discrepancy between the time courses of VPA concentrations and the antiepileptic activity of VPA (21). It appears that 2-ene-VPA does not possess the pronounced embryotoxicity (22) and hepatotoxicity (23) of 4-ene-VPA, and it may thus represent a better AED than VPA itself. The strongly hepatotoxic 4-ene-VPA is produced by the action of cytochrome P450 enzymes. These enzymes are induced by other AEDs such as phenobarbital, phenytoin, and carbamazepine (18,24), and this may explain the increased risk of hepatotoxicity in patients receiving these drugs together with VPA (25). However, elevation of 4-ene-VPA levels has not yet been documented in patients with VPA hepatotoxicity or in conjunction with short-term adverse effects or hyperammonemia (26).
FIGURE 66.1 Structural formula of VPA (N-dipropylacetic acid).
FIGURE 66.2 Main biotransformation pathways of valproate.
Source: From Ref. (17). Sankar R, Teratogenicity of antiepileptic drugs: role of drug metabolism and pharmacogenomics. Acta Neurol Scand. 2007:116;65–71. doi:10.1111/j.1600-0404.2007.00830.x. Reprinted with permission of John Wiley & Sons.
Pharmacokinetics
The main pharmacokinetic parameters of VPA are summarized in Table 66.1.
The bioavailability of oral preparations of VPA is virtually complete when compared to the intravenous route (27). The purpose of the enteric coating of tablets is to prevent the gastric irritation associated with release of VPA in the stomach. When VPA syrup was administered rectally, it was found to have the same bioavailability as the oral preparation (28,29). Compared to oral syrup, the bioavailability of VPA suppositories was found to be 80% in volunteers (30). The time to peak level was longer for the suppositories than for the syrup (3.1 hours vs. 1.0 hour). In a study in patients treated chronically with VPA, administration of VPA suppositories was well tolerated for several days, and the bioavailability was the same as for the oral preparations (31). The available evidence suggests that there is no need for a dosage change or a change in regimen when oral VPA administration is transiently replaced by rectal administration. The bioavailability of the divalproex extended-release preparation is 8% to 20% lower than that of other divalproex preparations (32,33).
Absorption of VPA after oral administration is quite variable and depends on the formulation. Administration of syrup and uncoated regular tablets or capsules is followed by rapid absorption, and peak levels are achieved within 2 hours. Absorption from enteric-coated tablets is delayed, but once absorption begins, it is rapid. The onset of absorption varies as a function of the state of gastric emptying at the time of ingestion, and peak levels may be reached only 3 to 8 hours after oral ingestion of enteric-coated tablets (34–36). Figure 66.3 shows the serum levels of a patient who received a first oral dose of 500 mg of enteric-coated divalproex sodium. No VPA was detectable in serum until 8 hours after the oral intake, and this was followed by a rapid rise of serum levels (37).
TABLE 66.1
PHARMACOKINETIC PARAMETERS OF VALPROATE | |
Bioavailability | >90% |
Time to peak levela | 1–8 hours |
Volume of distribution | 0.16 L/kg |
Serum protein bindingb | 70%–93% |
Elimination half-lifec | 5–15 hours |
Therapeutic range | 50–100 (–150) mg/L |
| 350–700 (–1,000) mcmol/L |
aThe longer values are for enteric-coated and slow-release preparations.
bConcentration dependent; lower at higher total concentrations.
cShorter values are for comedication with inducing drugs.
Because of this delayed absorption, serum levels of VPA in patients taking VPA chronically (twice or thrice daily) continue to decrease for 2 or more hours after drug ingestion. Therefore, the lowest VPA levels of a 24-hour cycle are not the assumed “trough levels” before the morning dose, but actually occur in the late morning or early afternoon (31). When using the divalproex formulation, measurement of plasma a level must be obtained within 2 hours after taking the first dose of the day. For comparison, patients should have levels measured at approximately the same time of day with any subsequent assessment. The absorption of enteric-coated sprinkles was compared to the absorption of VPA syrup in 12 children, and no difference in overall bioavailability was found between the two formulations. However, the absorption of VPA was slower from the sprinkles, with an average time to maximal VPA concentrations of 4.2 hours after ingestion of sprinkles, as compared with 0.9 hours after ingestion of syrup (38).
FIGURE 66.3 Valproate serum concentrations after a 500-mg single first oral dose of enteric-coated divalproex sodium in a 60.4-kg woman.
Source: Reproduced with permission from Ref. (37). Bourgeois BFD. Pharmacokinetics and pharmacodynamics of antiepileptic drugs. In: Wyllie E, ed. The Treatment of Epilepsy: Principles and Practice. 4th ed. Philadelphia, PA: Lippincott Williams & Wilkins, 2006:655–664.
The volume of distribution of VPA is relatively small (0.13–0.19 L/kg in adults and 0.20–0.30 L/kg in children). This suggests that VPA has a relatively lower affinity for binding outside the blood compartment than for binding to serum proteins. Indeed, VPA is highly bound to serum proteins. This binding appears to be saturable at therapeutic concentrations, and the free fraction of VPA increases as the total concentration increases. For instance, Cramer et al (39) found that the average free serum fraction of VPA in adults was only 7% at 50 mg/L and 9% at 75 mg/L, increasing to 15% at 100 mg/L, 22% at 125 mg/L, and 30% at 150 mg/L. Based on these values, with a three-fold increase in the total concentration of VPA from 50 to 150 mg/L, the free level of VPA would increase more than 10 times from 3.5 mg/L to 45 mg/L. Because the clearance of VPA is related to the free concentration, there is a linear relationship between the daily maintenance dose of VPA and steady-state free levels. But, as expected on the basis of saturable binding, a curvilinear relationship between the VPA maintenance dose and total steady-state concentrations was found by Gram and coworkers (40), with relatively smaller increases in concentrations at higher doses.
The elimination half-life of VPA is influenced by comedication. In the absence of inducing drugs, the half-life in adults is 13 to 16 hours (35,41), whereas the half-life in adults receiving polytherapy with inducing drugs averaged 9 hours (27); half-lives in children are slightly shorter. Cloyd and coworkers (42) found an average half-life of 11.6 hours in children receiving monotherapy and 7.0 hours with polytherapy. Hall and coworkers (43) determined that VPA half-lives were significantly related to age, but volume of distribution and clearance were not. Newborns eliminate VPA slowly, with half-lives that are longer than 20 hours (44).
Interactions
Pharmacokinetic interactions involving VPA fall into three categories based on the fact that (a) the metabolism of VPA is sensitive to enzymatic induction, (b) VPA itself can inhibit the metabolism of other drugs, and (c) VPA has a high affinity for serum proteins and can displace other drugs. Pharmacokinetic interactions with VPA have been reviewed previously (45). Concomitant administration of enzyme-inducing AEDs such as carbamazepine, phenytoin, phenobarbital, and primidone has been shown repeatedly to result in lower VPA levels in relation to the maintenance dose (46). The addition of both carbamazepine (47,48) and phenytoin (48) significantly lowers VPA levels, usually by at least one-third to one-half. These interactions are particularly pronounced in children, resulting in VPA level reductions of 50% or more (49,50). When children receiving polytherapy had other drugs discontinued, VPA levels rose 122% after withdrawal of phenytoin, 67% after withdrawal of phenobarbital, and 50% after withdrawal of carbamazepine (51). The antibiotic meropenem can decrease VPA levels to a clinically relevant degree (52). In general, when an inducing drug is added or withdrawn in a patient taking VPA, the dose of VPA must be increased or decreased, respectively, by a factor of about 2, if the same level is to be maintained. Clobazam has been found to reduce the clearance and elevate the levels of VPA (53).
Valproate affects the kinetics of other drugs with the interaction that are either enzymatic inhibition or a displacement from serum proteins. Levels of phenobarbital have been found to increase by 57% (54) to 81% (55) after the addition of VPA. Levels of ethosuximide also can be raised by the addition of VPA, mostly in the presence of additional AEDs (56). Although VPA does not raise levels of carbamazepine itself, levels of the active metabolite, carbamazepine-10,11-epoxide, may double (57,58). Elimination of lamotrigine is markedly inhibited by VPA, with a two-fold to three-fold prolongation of the lamotrigine half-life (59). This interaction is competitive and rapidly reversible, but it seems to persist at low VPA concentrations (60,61). Lamotrigine must be introduced at lower doses in patients taking VPA and a low dose ascension must be followed with the expectation that the target dose or plasma level will be one-half that of a patient treated with a drug other than VPA.
A pharmacokinetic interaction occurs between VPA and phenytoin because both drugs have a high affinity for serum proteins. VPA increases the free fraction of phenytoin (62,63). Because the free serum level of phenytoin determines the brain concentration, total phenytoin concentrations in the usual therapeutic range may, in the presence of VPA, be associated with clinical toxicity. In contrast to inducing AEDs, VPA is not associated with failure of oral contraceptives (64). Acetylsalicylic acid can both displace VPA from serum proteins and alter its metabolism (65). It appears that this interaction can be sufficient to cause clinical VPA toxicity (66).
CLINICAL EFFICACY
Absence Seizures
Valproate is a broad-spectrum AED with at least some degree of efficacy against most seizure types (67). It is a highly effective drug in the treatment of primarily generalized idiopathic seizures, such as absence seizures, generalized tonic–clonic seizures, and myoclonic seizures (68). The primary indication for VPA when it was first released in North America in 1978 was the treatment of absence seizures. When VPA was administered to patients with typical and atypical absence seizures, a reduction of spike-and-wave discharges was repeatedly demonstrated (69–74). Comparison of the efficacy of VPA and ethosuximide in the treatment of absence seizures revealed equal efficacy in at least two studies (75,76). Sixteen patients not previously treated for absence seizures and 29 refractory patients were enrolled in a double-blind crossover study of VPA and ethosuximide, in which the measure of efficacy was the frequency and duration of generalized spike-wave bursts on electroencephalogram (EEG) telemetry (76). Ethosuximide and VPA were equally effective in previously untreated patients. An open randomized comparison of VPA and lamotrigine revealed that VPA achieved seizure control much faster than lamotrigine, but the overall seizure freedom at 1 year did not differ (77). According to other reports, simple absence seizures could be completely controlled by VPA monotherapy in 82% to 95% of patients (51,78–80). Overall review of available evidence did not demonstrate a difference in efficacy between VPA, lamotrigine, and ethosuximide against absence seizures, possibly because of the methodologic quality or statistical power of the studies (81). It appears that absence seizures are more likely to be fully controlled if they occur alone than if they are mixed with another seizure type (51,80). Overall, treatment with VPA appears to be less effective against atypical or “complex” absences than against simple absences (80,82). VPA can be used effectively in patients with recurrent absence status (83). The largest double-blind, randomized controlled clinical trial ever conducted in a pediatric epilepsy cohort compared the efficacy, tolerability, and neuropsychologic effects of ethosuximide, VPA, and lamotrigine in children with newly diagnosed childhood absence epilepsy (84). VPA and ethosuximide were significantly more effective than lamotrigine in controlling seizures, and ethosuximide was better tolerated than VPA (VPA had higher rates of attentional problems). The mean daily dose of VPA in this trial was 35 mg/kg/day with a steady-state serum concentration of 94 mcg/mL.
Generalized Tonic–Clonic Seizures
Valproate was found to be highly effective in the treatment of certain types of generalized convulsive seizures (85–88). In 36 patients with primarily generalized tonic–clonic seizures, of whom 24 had been treated previously with other AEDs, VPA was used in monotherapy (78). In 33 of these patients, seizures were fully controlled. Among 42 patients with intractable seizures, the generalized tonic–clonic seizures were fully controlled by add-on VPA in 14 patients (7). VPA was compared to phenytoin in 61 previously untreated patients with generalized tonic–clonic, clonic, or tonic seizures (89). The seizures came under control during the time of observation in 73% of patients receiving VPA and in 47% of patients treated with phenytoin. Discounting seizures that occurred before therapeutic plasma drug levels had been reached, this response increased to 82% for VPA and 76% for phenytoin. In another randomized study comparing valproate and phenytoin in patients with previously untreated tonic–clonic seizures, a 2-year remission was achieved in 27 of 37 patients with VPA and in 22 of 39 patients with phenytoin (86). Monotherapy with VPA was assessed in two studies of patients with primary (or idiopathic) generalized epilepsies (80,81). Among patients who had only generalized tonic–clonic seizures, complete seizure control was achieved in 51 of 70 patients (81) and in 39 of 44 patients (80), respectively. Monotherapy with VPA in children with generalized tonic–clonic seizures was found to be highly effective (90).
Myoclonic Seizures
VPA is currently a drug of first choice for most myoclonic seizures, particularly those occurring in patients with primary or idiopathic generalized epilepsies (78–80). In a group of 23 patients with myoclonic epilepsy of adolescence, 16 came under complete control with VPA monotherapy (78). In the same study, in 22 patients with myoclonic epilepsy of adolescence and abnormality on intermittent photic stimulation, of whom 17 had failed to respond to previous medications, full seizure control was achieved in 17 patients. Photosensitivity on the EEG is easily suppressed by VPA, regardless of the associated clinical seizure type (91). Among a group of patients with primary generalized epilepsies treated with VPA monotherapy, 22 patients had myoclonic seizures and 20 of those had at least one other seizure type, either absence or tonic–clonic seizures. The myoclonic seizures were controlled by VPA monotherapy in 18 of these 22 patients (79). Patients with juvenile myoclonic epilepsy have an excellent response to VPA (92), which still remains a drug of first choice for this condition in most patients, at least in terms of efficacy (93,94). Benign myoclonic epilepsy of infancy, which belongs to the group of primary or idiopathic generalized epilepsies, also responds well to treatment with VPA (90). Postanoxic intention myoclonus is usually refractory to treatment, but some success has been achieved with VPA (95–97). A combination of VPA and clonazepam was advocated in the treatment of the myoclonic and tonic–clonic seizures in patients with severe progressive myoclonus epilepsy (98).
Infantile Spasms and Lennox–Gastaut Syndrome
The available information on the use of VPA in the treatment of generalized encephalopathic epilepsies of infancy and childhood, such as West syndrome and Lennox–Gastaut syndrome, is much less extensive than for the more benign idiopathic primary generalized epilepsies. Like all other antiepileptic medications, VPA is less effective in the treatment of these severe forms of generalized epilepsy. In a larger series of patients treated with VPA, 38 had myoclonic astatic epilepsy, a term used by the authors synonymously with Lennox–Gastaut syndrome (78). Of these patients, seven became and remained seizure-free with VPA. In addition, a 50% to 80% improvement was achieved after the addition of VPA in one-third of these patients, and other AEDs were withdrawn or reduced. In the same series, seizures were fully controlled in three of six patients with myoclonic absence epilepsy, all of them on combination therapy. In another series involving 100 children treated with VPA (51), seizure control was achieved in 12 of 27 children with “absences and other seizures” and in 9 of 39 children with atonic seizures.
The majority of reports on the use of VPA for the treatment of infantile spasms include small numbers of patients (99–101), or they include patients receiving corticotropin and VPA simultaneously (102,103). VPA was used without corticotropin in one series of 19 infants with infantile spasms (104). With VPA as their first drug, 8 of these 19 infants experienced good seizure control and did not require corticotropin. These patients received VPA doses ranging from 20 to 60 mg/kg/day. The patients who experienced an initial failure with either VPA or corticotropin were subsequently switched to the other drug. Comparison of the two groups revealed a tendency toward a better response to corticotropin, but the incidence and severity of side effects was lower with VPA. A low dose of 20 mg/kg/day of VPA was used in a series of 18 infants with infantile spasms not previously treated with corticotropin (105). In 12 of these patients, the short-term results were described as good to excellent. On follow-up, 7 patients had residual seizure activity, and moderate to severe intellectual disability was diagnosed in 16. The authors concluded that the efficacy of VPA was similar to the efficacy of corticotropin and that VPA was associated with fewer side effects.
Partial Seizures
Systematic assessment of the efficacy of VPA against partial seizures began only after its role in the treatment of generalized seizures had been established. Preliminary information had been obtained from subgroups of patients in studies not dealing primarily with partial seizures, all of which suggested some benefit (51,78,106). The first direct comparison of VPA with carbamazepine in the treatment of partial seizures was an open study in 31 previously untreated adults (107). Eleven patients receiving VPA and eight patients receiving carbamazepine were controlled, but follow-up was less than 1 year in 12 of the 31 patients. Comparison between carbamazepine, phenytoin, and VPA in monotherapy in a prospective study of 79 patients with previously untreated simple partial or complex partial seizures revealed no difference in efficacy among the three drugs (108). A group of 140 adults with previously untreated seizures were randomized to monotherapy with phenytoin or VPA (109). The seizures were tonic–clonic in 76 patients and predominantly complex partial in 64. Determination of this 2-year remission rate or time to first seizure revealed no difference between the two drugs in either subgroup. A retrospective study of VPA monotherapy in 30 patients with simple partial and complex partial seizures in whom previous drugs had failed revealed a remarkable response (110). Seizure control was achieved in 12 patients, a more than 50% seizure reduction occurred in 10 patients, and only 9 patients were not improved.
Mattson and coworkers (111) conducted a controlled comparison of VPA and carbamazepine monotherapy in the treatment of partial and secondarily generalized seizures. This multicenter, double-blind, randomized trial included 480 adult patients in whom several seizure indicators, as well as neurotoxicity and systemic toxicity, were assessed quantitatively. Four of five efficacy indicators were significantly in favor of carbamazepine against complex partial seizures, and a combined composite score for efficacy and toxicity was higher for carbamazepine than for VPA at 12 months, but not at 24 months. Outcomes for secondarily generalized seizures did not differ between the two drugs. A double-blind, placebo-controlled add-on to phenytoin and to carbamazepine in 137 patients showed a responder rate of 38% with VPA compared to 19% with placebo (112).
Two comparative studies of VPA were carried out specifically in children (113,114). A total of 260 children with newly diagnosed primary generalized or partial epilepsy were randomized to VPA or carbamazepine and followed for 3 years (113). The doses were titrated as needed and as tolerated according to clinical response. Equal efficacy was found for the two drugs against generalized and partial seizures, and adverse events were mostly mild for both drugs. The four drugs, phenobarbital, phenytoin, carbamazepine, and VPA, were compared in 167 children with untreated tonic–clonic or partial seizures entered into a randomized, unblinded study (114). Based on time to first seizure and to 1-year remission, there was no difference in efficacy at 1, 2, or 3 years. Unacceptable side effects necessitating withdrawal occurred in 6 of 10 patients on phenobarbital, which was prematurely eliminated from the study; in 9% of children taking phenytoin; and in 4% each of children taking carbamazepine or VPA. When trials comparing VPA and carbamazepine were subjected to a meta-analysis, carbamazepine was more effective than VPA for time to first seizure and for time to 12 months of remission (115). Valproate therapy was evaluated in 143 adult patients with poorly controlled partial epilepsy randomized to monotherapy with VPA at low levels (25–50 mg/L) or high levels (80–150 mg/L) (116). The reduction in the frequency of both complex partial and secondarily generalized tonic–clonic seizures was significantly higher among patients in the high-level group.
Other Uses of Valproate in the Treatment of Seizures
Several studies have assessed the efficacy of VPA in the prevention of febrile seizures (117–123). In some studies, VPA was found to be as effective as phenobarbital, and in other studies it was more effective than phenobarbital, placebo, or no treatment in reducing seizure recurrence. Nevertheless, based on risk-versus-benefit considerations, VPA cannot be recommended for the prophylaxis of febrile seizures. Treatment with intermittent diazepam during febrile episodes was as effective as prophylactic VPA in children with a high risk of recurrence of febrile seizures (124). A small number of newborns with seizures have been treated with VPA either rectally (125) or orally (44). Overall results were favorable. A loading dose of 20 to 25 mg/kg was followed by a maintenance dose of 5 to 10 mg/kg every 12 hours (44). Newborns treated with VPA were found to have a longer elimination half-life (26.4 hours) and higher levels of ammonia.
ADVERSE EFFECTS
Although adverse effects of AEDs are commonly divided into those that are dose related and those that are idiosyncratic, the distinction is not always apparent, particularly with VPA. Some reactions, such as tremor, are fairly predictable and dose related. However, certain adverse events that only occur in a small fraction of patients, and therefore appear to be idiosyncratic, may be more likely to occur at high doses or levels of VPA, such as thrombocytopenia, certain cases of confusion or stupor, and neural tube defects. Finally, certain side effects that are too common to be considered idiosyncratic could never be shown clearly to be dose related, such as hair changes and weight gain. The main adverse effects of VPA are summarized in Table 66.2.
Neurologic Adverse Effects
A tremor with the characteristics of an essential tremor is relatively common with VPA (126,127). It is dose related and occurs in about 10% of patients. If it does not improve sufficiently with dose reduction, propranolol may be effective (127). Asterixis (128) and reversible parkinsonism (129–131) have been described. Drowsiness, lethargy, and confusional states are uncommon with VPA, but may occur in some patients, usually at levels greater than 100 mg/L. There have been well-documented cases of reversible dementia (129,132,133) and pseudoatrophy of the brain (133–135). Treatment with VPA has been associated with a rather specific and unique adverse effect characterized by an acute mental change that can progress to stupor or coma (136,137). It is usually associated with generalized delta slowing of the EEG tracing. The mechanism is not known with certainty, and hyperammonemia or carnitine deficiency can be associated (138); occasionally, patients who develop severe acidosis are found to have multiple Co-enzyme A (CoA) dehydrogenation defect, with precipitation of symptoms because of the tendency of VPA to sequester CoA, forming valproyl-CoA (139,140). This encephalopathic picture is more likely to occur when VPA is added to another AED, and it is usually reversible within 2 to 3 days upon discontinuation of VPA or of the other AED. Overall, VPA does not appear to be associated with a high incidence of dose-related effects on cognition or behavior (141–145).
TABLE 66.2
ADVERSE EFFECTS OF VALPROATE |
Neurologic |
Tremor, drowsiness, lethargy, confusion, reversible dementia, brain atrophy, encephalopathy |
Gastrointestinal |
Nausea, vomiting, anorexia, gastrointestinal distress, hepatic failure, pancreatitis |
Hematologic |
Thrombocytopenia, decreased platelet aggregation, fibrinogen depletion |
Metabolic/Endocrinologic |
Hyperammonemia, hypocarnitinemia, hyperinsulinism, menstrual irregularities, polycystic ovaries |
Teratogenicity |
Major malformations, including neural tube defect and possible developmental delay in offspring, facial dysmorphism |
Miscellaneous |
Hair loss, edema, nocturnal enuresis, decreased bone mineral density |
Gastrointestinal Adverse Effects
The most frequent gastrointestinal adverse effects of VPA are nausea, vomiting, gastrointestinal distress, and anorexia. They may be due, in part, to direct gastric irritation by VPA, and the incidence is lower with enteric-coated tablets. Excessive weight gain is another common problem (146–149). It is not due entirely to increased appetite, and decreased beta-oxidation of fatty acids has been postulated as a mechanism (150). More recently, obese VPA-treated men were found to have higher serum insulin levels than obese control subjects (151,152). The mechanism for this may be interference with hepatic insulin metabolism rather than increased insulin secretion or insulin resistance (153). In addition, patients who developed obesity while taking VPA had higher leptin levels and lower ghrelin and adiponectin levels (152). Despite recommendations for diet and exercise, weight gain tends to be a bothersome side effect, especially in young women. Excessive weight gain seems to be less of a problem in children, and one study suggests that VPA does not cause more weight gain than carbamazepine in children (154).
Hepatotoxicity remains the most feared adverse effect of VPA (155). Two prominent risk factors for fatal hepatotoxicity are young age and treatment with polytherapy (24,156,157). The risk of fatal hepatotoxicity while receiving polytherapy with VPA was found to be approximately 1 in 600 younger than the age of 3 years; 1 in 8,000 from 3 to 10 years; 1 in 10,000 from 11 to 20 years; 1 in 31,000 between 21 and 40 years; and 1 in 107,000 older than the age of 41 years (24). The risk is much lower for monotherapy and varies between 1 in 16,000 (3–10 years old) and 1 in 230,000 (21–40 years old). When initiating treatment with VPA, it is important to obtain baseline hepatic studies along with CBC and platelet count. Because a benign elevation of liver enzymes is common with VPA, and the severe hepatotoxicity usually is not preceded by a progressive elevation of liver enzymes, laboratory monitoring is of little value, although it is commonly done routinely (158). The diagnosis of hepatic failure due to VPA depends mostly on recognition of the clinical features that include nausea, vomiting, anorexia, lethargy, jaundice, edema, and at times loss of seizure control. Although increased production of toxic metabolites has been considered as a cause of VPA hepatotoxicity, this has not yet been documented (159,160). There has been evidence of a protective effect of carnitine administration (especially intravenously) in cases of severe VPA hepatotoxicity (161). A panel of pediatric neurologists concluded that daily L-carnitine supplementation is strongly suggested in several situations, including certain secondary carnitine deficiency syndromes, symptomatic VPA-associated hyperammonemia, multiple risk factors for VPA hepatotoxicity or renal-associated syndromes, infants and young children taking VPA, patients with epilepsy using the ketogenic diet who have hypocarnitinemia, patients undergoing dialysis, and premature infants who are receiving total parenteral nutrition (162). Recently, a direct relationship between DNA polymerase gamma (POLG)-mutations and valproate-induced hepatotoxicity has been ascertained, leading some to suggest this mutation be screened for in high-risk infants (or in those who develop hepatic problems) prior to starting valproate (163).
Another serious complication of VPA treatment is the development of acute hemorrhagic pancreatitis (164–169). Suspicion should be raised by the occurrence of vomiting and abdominal pain. Serum amylase and lipase are the most helpful diagnostic tests, and abdominal ultrasound may be considered. However, amylase may be elevated in 20% of asymptomatic patients taking VPA (170), and pancreatitis has been described in a patient with normal amylase but elevated lipase (171).
Hematologic Adverse Effects
Hematologic alterations are relatively common with VPA therapy, but they seldom lead to discontinuation of the drug (172–174). By far, the most frequently diagnosed hematologic adverse event is thrombocytopenia (175,176), which tends to improve with dosage reductions. The thrombocytopenia, in conjunction with other VPA-mediated disturbances of hemostasis, such as impaired platelet function, fibrinogen depletion, and coagulation factor deficiencies (177–180), may cause excessive bleeding. The common practice of discontinuing VPA about 1 month before elective surgery is therefore recommended, especially for operative procedures considered to be associated with higher blood losses, although several reports have found no objective evidence of excessive operative bleeding during neurosurgic procedures in patients maintained on VPA (181–183). In addition to the described hematologic changes, VPA can occasionally cause neutropenia (184), bone marrow suppression (185), and systemic lupus erythematosus (186).
Metabolic and Endocrinologic Changes, Effect on Offspring
Hyperammonemia is a common finding in asymptomatic patients receiving chronic VPA therapy, and routine monitoring of ammonia is not warranted. Because ammonia levels were initially measured in symptomatic patients, their elevation was thought to be the cause of the symptoms (187–189). It was later found that hyperammonemia is common in asymptomatic patients, particularly in those taking VPA together with an enzyme-inducing AED (190,191). The origin of the excessive ammonium may be renal (192). Although hyperammonemia can be reduced by L-carnitine supplementation (193), there is no documentation that this is necessary or clinically beneficial (194). Chronic treatment with VPA, especially in polytherapy, tends to lower carnitine levels (195,196). A role for carnitine deficiency in the development of severe adverse effects of VPA has never been established. One patient who developed an acute encephalopathy and cerebral edema after acute administration of VPA was found to have low carnitine levels (197). A beneficial role of L-carnitine supplementation in acute VPA overdoses has been suggested (198,199).
VPA can cause menstrual irregularities (200), hormonal changes such as hyperandrogenism and hyperinsulinism (201–205), and pubertal arrest in women (206). Another concern has been the association between VPA therapy and polycystic ovaries (201–203,207). To what extent these observations are significant and reproducible is an open and debated issue (208–215).
When taken during pregnancy, VPA is associated with an increase of major malformations and of developmental delay in the offspring (216). Treatment with VPA during the first trimester of pregnancy is associated with an estimated 1% to 2% risk of neural tube defect in the newborn (217–219). This risk appears to be greater at higher doses of VPA (217). Finally, a more recent concern has been that developmental delay may result from exposure to VPA in utero (220). Valproate should be avoided in females with childbearing potential unless seizure control cannot be achieved by another medication.
Miscellaneous Adverse Effects
Excessive hair loss may be seen early during treatment with VPA, and, although the hair tends to grow back, it may become different in texture (221) or color (222). Facial or limb edema can occur in the absence of VPA-induced hepatic injury (223). Children may develop secondary nocturnal enuresis during VPA therapy (224). Hyponatremia (225) was reported in one patient. The occurrence of rashes with VPA is extremely rare (226). Bone mineral density may be decreased by VPA, with increased risk for fractures (227–231). The precise mechanism has not been elucidated, and VPA, unlike enzyme-inducing drugs, does not cause bone loss through hypovitaminosis D. In an analysis of reports, carbamazepine and VPA have a limited decrease in bone mineral density, but pooled data on bone growth is limited (232). This review suggested that lack of deficiency of vitamin D was of importance.
CLINICAL USE
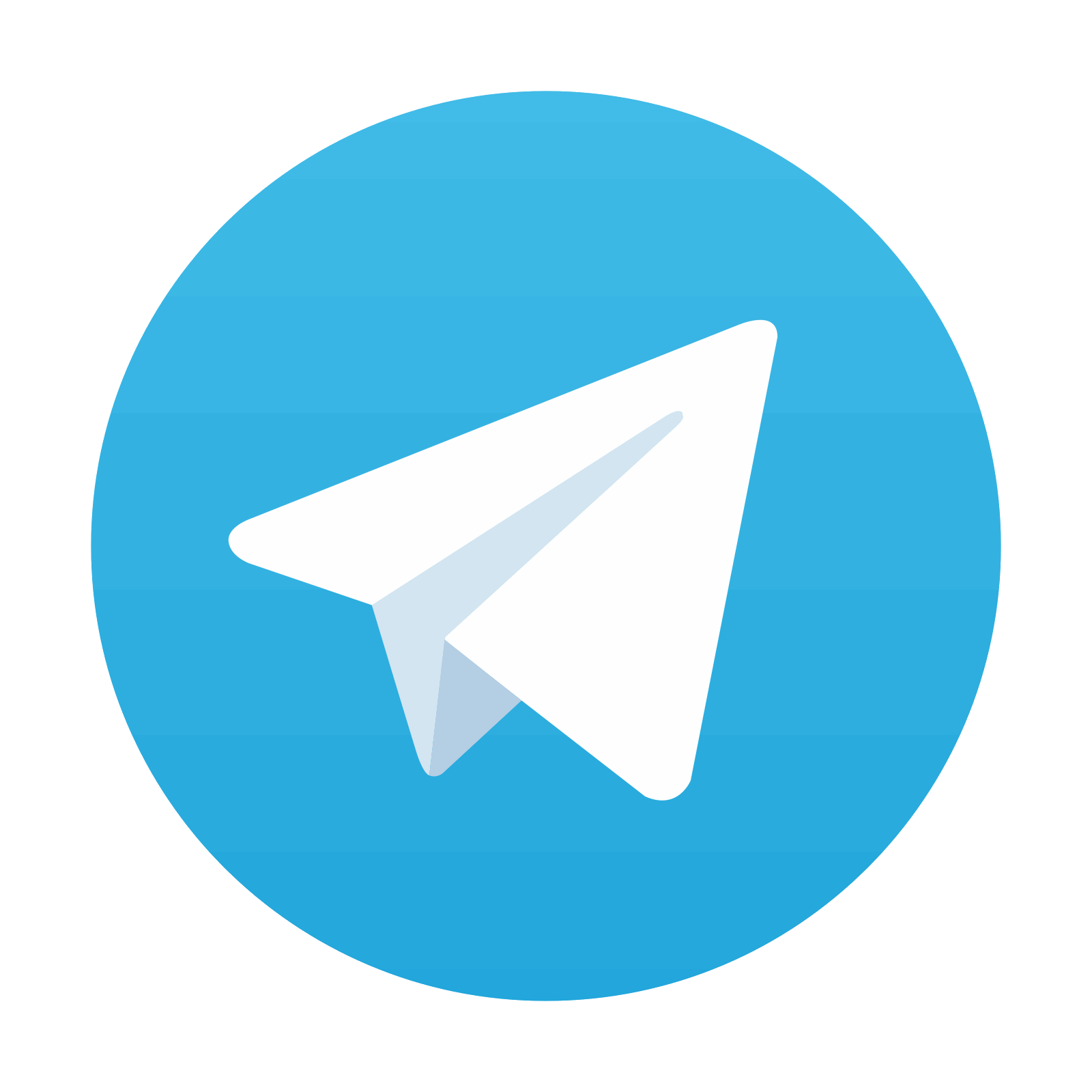
Stay updated, free articles. Join our Telegram channel

Full access? Get Clinical Tree
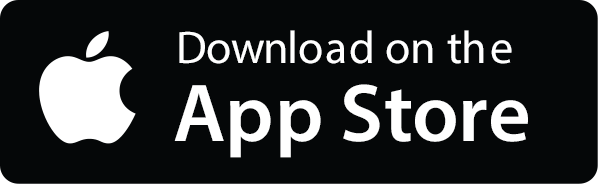
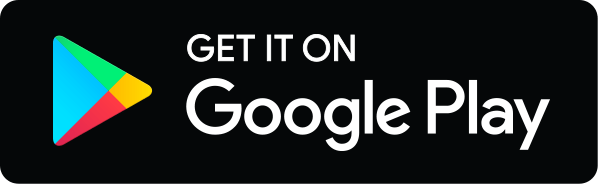