Invasive prenatal diagnosis allows vital details to be obtained regarding the fetus that can include genetic, biochemical, or physiologic data. This chapter describes amniocentesis, chorionic villus sampling (CVS), and fetal blood sampling (FBS), which are the most frequently used invasive techniques to obtain this information. Most perinatal centers have the technical expertise to perform all of these procedures and to select the best method for each clinical scenario. However, certain conditions meriting specialized techniques may require referral to a fetal center.
To complement diagnostic imaging and invasive fetal procedures, perinatal centers must provide detailed genetic counseling. With this, available clinical options are described, their risks are explained, and their consequences are communicated in a nondirective, supportive way.
Myriad tests for prenatal screening are available to the practicing obstetrician. The American College of Obstetricians and Gynecologists (2016b) recommends that all pregnant women regardless of age be offered aneuploidy screening before 20 weeks’ gestation. Diagnostic testing is then offered to women whose screening test results are positive. In that same publication, the College also endorsed that all pregnant women, regardless of age, should be counseled regarding the option of invasive or diagnostic fetal testing after a thorough explanation of the differences between screening and diagnostic tests.
Invasive prenatal tests yield diagnostic results but have the potential for procedure-related complications. Therefore, pretest counseling by the obstetrician or maternal-fetal medicine (MFM) specialist or by a genetic counselor or medical geneticist is recommended. Elements of counseling are listed in Table 13-1.
Indication for the test Risks/potential complications of procedure Alternative therapies or natural history of condition if expectant management elected Specific test(s) ordered Diagnostic accuracy and test limitations Gestational age range for which an invasive test can be safely performed Waiting interval before a final result is expected Postprocedural activity limitations |
Prior to the procedure, the patient is ideally instructed regarding suggested postprocedural activity restrictions. Further, a description of how complications will be evaluated is recommended.
Prior to any invasive test, several procedural steps are recommended. Needed specimen containers are assembled and are prelabeled to reduce errors. Laboratory requisitions are similarly premarked to reflect the procedure and type of specimen.
A “time out” is suggested, given the risk of pregnancy complications including fetal loss. Elements that are reviewed and confirmed include correct patient identity and medical record number, the planned procedure, tests to be performed from the samples, potential intraprocedural complications, correct specimen labeling, and pertinent maternal laboratory results. The latter may include maternal blood type, Rh status, and positive results from hepatitis B, hepatitis C, or human immunodeficiency (HIV) testing. Following amniocentesis, CVS, and FBS, all rhesus-negative women (without alloimmunization to D) should receive prophylactic administration of Rh(D) immune globulin.
Table 13-2 lists some of the common indications for genetic amniocentesis. The most frequent reason is identification of genetic defects. Other less often used purposes are evaluation of fetal anemia or pulmonary maturity and a search for infection.
Timing | Indication |
---|---|
2nd trimester (15–17 wks) | Obtain cells for karyotype Diagnose certain metabolic disorders Assay amnionic fluid AFP levela |
Late 2nd/early 3rd trimester | Determine alloimmunization severityb Diagnose intraamnionic infection/inflammation Insert dye to diagnose ruptured membranes Confirm fetal lung maturityc Amnioreduction in singleton or multifetal gestation |
Therapeutic | Treatment of hydramnios Fluid removal prior to cerclage placement |
In the United States, most providers offer prenatal genetic analysis of a fetal sample to all women who will be 35 years or older at their expected date of delivery. This approach is based on the increased risk of aneuploidy seen with advancing maternal age. Selection of this age as the threshold for offering invasive testing is arbitrary. It was chosen more than 30 years ago as a pragmatic balance between the available laboratory resources, the relatively limited information regarding the safety of the emerging technology of amniocentesis, and the fetal genetic risks. As seen in Table 13-3, this threshold still seems a reasonable balance between the 1:200 risk of a chromosomal abnormality and the known risks of the amniocentesis procedure (Hook, 1981, 1983). Presently, approximately 7.5 percent of pregnant women will be of advanced maternal age, and approximately 30 percent of Down syndrome births will occur in this population. Because most Down syndrome births occur in women younger than 35 years, maternal age alone is an inefficient screening criterion. As stated above, the American College of Obstetricians and Gynecologists (2016a) endorses offering diagnostic testing to all women regardless of age. Thus, appropriate risk/benefit counseling is indicated prior to testing.
Age | Risk of Liveborn Down Syndrome | Risk of Liveborn Chromosomal Abnormality |
---|---|---|
20 | 1/1667 | 1/526 |
25 | 1/1250 | 1/476 |
30 | 1/952 | 1/385 |
35 | 1/385 | 1/202 |
36 | 1/295 | 1/162 |
37 | 1/227 | 1/129 |
38 | 1/175 | 1/102 |
39 | 1/137 | 1/82 |
40 | 1/106 | 1/65 |
41 | 1/82 | 1/51 |
42 | 1/64 | 1/40 |
43 | 1/50 | 1/32 |
44 | 1/38 | 1/25 |
45 | 1/30 | 1/20 |
46 | 1/23 | 1/16 |
47 | 1/18 | 1/13 |
48 | 1/14 | 1/10 |
49 | 1/11 | 1/7 |
As a surrogate for amniocenteses, less-invasive screening tests that measure maternal serum analytes have been developed. These are often selected by gravidas at lower risk for genetic defects. Both first- and second-trimester screening algorithms yield a risk assessment for Down syndrome. For results that show an elevated aneuploidy risk, more invasive prenatal testing is then typically offered.
The first-trimester combined screen measures both nuchal translucency thickness and maternal serum analyte concentrations. Specific analytes are free β-human chorionic gonadotropin (hCG) and pregnancy-associated plasma protein A (PAPP-A). This combined screening approach has an 85-percent detection rate for Down syndrome and a 5-percent false-positive or screen-positive rate.
For second-trimester screening, the quadruple-marker test or “quad test” is the most often used serum screening test for aneuploidy. With this, the four measured maternal serum analytes are maternal serum alpha-fetoprotein (MSAFP), hCG, unconjugated estriol, and inhibin A. The quad test yields an 81-percent detection rate for Down syndrome with a 5-percent screen-positive rate (Dugoff, 2010). An abnormal screening test is defined as one in which the combined age and biochemical risk for Down syndrome exceeds the second-trimester risk of a 35-year-old woman, which is 1 affected neonate per 270 live births (1:270).
As described in the last section, several approaches for first- and second-trimester aneuploidy screening have been developed with the goal of offering a minimally invasive test with a high detection but low screen-positive rate.
In addition to these, technologic advancements in sonographic scanning now permit fetal structural anomalies to be found at an early gestational age. And, this is often the finding that prompts genetic amniocentesis or CVS. Specifically, Table 13-4 lists many major structural defects that have a high association with fetal aneuploidy. In addition, soft sonography markers can add predictive power. For example, nuchal thickening, hyperechoic bowel, short humerus or femur length, echogenic intracardiac focus, choroid plexus cysts, and pyelectasis increase the likelihood of aneuploidy (Nyberg, 1993, 2001; Rotmensch, 1997; Smith-Bindman 2001).
Abnormality | Birth Prevalence | Aneuploidy Risk (%) | Common Aneuploidiesa |
---|---|---|---|
Cystic hygroma | 1/5000 | 50–70 | 45X, 21, 18, 13, triploidy |
Nonimmune hydrops | 1/1500–4000 | 10–20 | 21, 18, 13, 45X, triploidy |
Ventriculomegaly | 1/1000–2000 | 5–25 | 13, 18, 21, triploidy |
Holoprosencephaly | 1/10,000–15,000 | 30–40 | 13, 18, 22, triploidy |
Dandy Walker malformation | 1/12,000 | 40 | 18, 13, 21, triploidy |
Cleft lip/palate | 1/1000 | 5–15 | 18, 13 |
Cardiac defects | 5–8/1000 | 10–30 | 21, 18, 13, 45X, 22q11.2 microdeletion |
Diaphragmatic hernia | 1/3000–4000 | 5–15 | 18, 13, 21 |
Esophageal atresia | 1/4000 | 10 | 18, 21 |
Duodenal atresia | 1/10,000 | 30 | 21 |
Gastroschisis | 1/2000–4000 | No increase | |
Omphalocele | 1/4000 | 30–50 | 18, 13, 21, triploidy |
Clubfoot | 1/1000 | 5–30 | 18, 13 |
For any of the indications above, genetic amniocentesis removes amnionic fluid and amniocytes for biochemical or DNA studies. Molecular abnormalities responsible for many disorders are being identified at a rapid rate, and thus any list is quickly rendered outdated. However, the more common genetic conditions for which DNA-based prenatal diagnosis is indicated are shown in Table 13-5. Many of these conditions are rare, and their diagnosis is so complex that consultation with a genetics unit is encouraged prior to performing an invasive test.
Disorder | Mode of Inheritance | Prenatal Diagnosis |
---|---|---|
α1-Antitrypsin deficiency | AR | Determine PiZZ allele. Not all homozygotes have liver involvement; preprocedural genetic counseling critical |
α-Thalassemia | AR | α-Hemoglobin gene |
Adult polycystic kidney | AD | PKD1 and PKD2 gene mutation. In large families, linkage is possible in >90%. Gene mutation identifiable in 50% of PKD1 and 75% of PKD2 |
β-Thalassemia | AR | β-Hemoglobin gene mutation |
Congenital adrenal hyperplasia | AR | CYP21A2 gene mutations/deletions. Nine common mutations/deletions detect 90 to 95% of carriers. Sequencing available |
Cystic fibrosis | AR | CFTR gene mutation |
Duchenne/Becker muscular dystrophy | XLR | Dystrophin gene mutation |
Fragile X syndrome | XLR | CGG repeat number |
Hemoglobinopathy (SS, SC) | AR | β-Chain gene mutation |
Hemophilia A | XLR | Factor VIII gene inversion 45%, other gene mutations 45% (not available in all labs), linkage analysis in appropriate families |
Huntington disease | AD | CAG repeat length (PGD and noninforming PND possible to avoid disclosing presymptomatic parents’ disease status |
Marfan syndrome | AD | Fibrillin (FBN-1) gene mutation. Linkage in large families. Approximately 70% have mutation identified |
Myotonic dystrophy | AD | CTG expansion in the DMPK gene |
Neurofibromatosis type 1 | AD | NF1 gene mutation identifiable in >95% of cases but requires sequencing. Linkage in appropriate families |
Phenylketonuria | AR | 4 to 15 common mutations, 40% to 50% detection. Further mutation analysis >99% detection |
Retinoblastoma | AD | Mutation in both copies of the gene RB1 |
Spinal muscular atrophy | AR/AD | |
Tay-Sachs disease | AR | Enzyme absence; gene mutation |
As an adjunct to standard karyotyping, chromosomal microarray (CMA) is a newer technique to detect chromosomal abnormalities (microdeletions and duplications) that are not diagnosable by traditional karyotyping.
The screening test for open spina bifida is a second-trimester measurement of MSAFP levels. In the past, amniocentesis was considered a good screening test. However, it currently has been supplanted by sonography because of the advances in image quality. In many centers, sonographic diagnosis of spina bifida has been greatly enhanced by the recognition of associated abnormalities of the skull and brain. These include ventriculomegaly, microcephaly, concave deformity of the frontal bones (lemon sign), and obliteration of the cisterna magna, which leads to an abnormal anterior curvature of the cerebellar hemispheres (banana sign) (Nicolaides, 1986a; UK Collaborative Study, 1979).
Patients at increased risk for a neural-tube defect include those with an elevated MSAFP level during screening or those with a previously affected child. These gravidas should be referred to centers experienced in high-resolution fetal sonography. If sonographic examination demonstrates a normal fetal spine, cranium, and cerebellum, the chance of an undetected open spinal abnormality is low. Amniocentesis can be reserved for patients with fetuses possessing suspicious sonography findings or with large MSAFP elevations despite a normal sonographic scan. Also, it is suitable for at-risk women in whom the ability to visualize fetal anatomy is inadequate. Importantly, in women without access to sonographic services, MSAFP level testing may still play a limited role to identify those at increased risk for a fetal open neural-tube defect.
In addition to genetic information, access to amnionic fluid can provide vital data regarding several conditions affecting the fetus. Of these, preterm birth is a leading cause of perinatal morbidity, mortality, and long-term adverse outcomes. An association between intrauterine infection/inflammation, preterm labor, and chronic sequelae has been demonstrated (Yoon, 2000). Because of this, amniocentesis for women with preterm labor or prior to rescue cerclage placement may be considered in some cases. Testing for subclinical infection/inflammation evaluates a Gram stain, amnionic fluid glucose level, and white blood cell count. In patients with more overt clinical findings of intraamnionic infection, amniocentesis may play a role because the results of the amnionic fluid culture can change antimicrobial selection. More recently, the advent of commercially available point-of-care testing of amnionic fluid provides reliable identification of intraamnionic inflammation at the bedside (Chaemsaithong, 2016; Nien, 2006). This may lead to an increased use of diagnostic amniocentesis in women with suspected preterm labor.
Because of the availability of sonography for early pregnancy dating, assessment of amnionic fluid for fetal lung maturity is infrequently needed. However, in indicated cases, contemporary methods use fluorescence polarization and lamellar body counts to assess fluid (Neerhof, 2001). Although previously used for this indication, lecithin/sphingomyelin ratio and phosphatidylglycerol level methods are less commonly selected (Gluck, 1974; Hallman, 1976).
Fetal hemolytic disease severity and need for fetal blood transfusion or early delivery were previously determined from amniocentesis. Fluid was then interpreted using spectrophotometric estimation of amnionic fluid bilirubin levels (Liley, 1961). This evaluation is less accurate in the midtrimester, and direct measurement of the fetal hemoglobin concentration by FBS was instead previously used in suspected cases (Nicolaides, 1986b, 1988).
However, in contemporary practice, sonographic estimation of peak systolic blood flow velocity through the fetal brain’s middle cerebral artery yields diagnostic information regarding the degree of fetal anemia. This noninvasive assessment is now a validated and widely used modality (Moise, 2012). Thus, cases requiring amniocentesis, which is more invasive, are rapidly declining in number (Mari, 2000).
Many abnormalities of lipid, mucopolysaccharide, amino acid, and carbohydrate metabolism are amenable to prenatal diagnosis from the study of cultured amnionic fluid cells. However, the earlier diagnosis and the substantially larger amount of tissue obtained by CVS make it the preferred method of diagnosing this group of disorders.
Genetic amniocentesis is commonly performed in the midtrimester between 15 and 18 weeks’ gestation. At this gestational age, the amount of fluid is adequate (approximately 150 mL), the ratio of viable to nonviable amniocytes is greatest, and in most cases, the amnion has fused with the chorion. This last attribute more easily permits successful cavity entry and thereby reduces the risk for premature rupture of membranes.
Prior to the procedure, a sonographic examination is performed to determine fetal number, confirm gestational age, assure fetal viability, document fetal anatomy, and locate the placenta and placental cord insertion.
The maternal abdomen is cleansed with an antiseptic solution (povidone-iodine or chlorhexidine) (Figs. 13-1 and 13-2). Antibiotic prophylaxis prior to amniocentesis is not recommended based on evidence. One trial evaluated the fetal loss rate within the 22nd week of pregnancy in untreated women versus those who received prophylactic agents. Compared with untreated women, those who received antibiotics had a lower loss rate, but the difference did not reach statistical significance (Gramellini, 2007).
Usually, 20- or 22-gauge spinal needles in differing lengths are assembled, and the needle should be sufficiently long to reach the target pocket (Fig. 13-3). Selection accounts for placental location, maternal abdominal wall thickness, and possible uterine contraction in response to needle insertion, which temporarily widens the myometrial wall. Local anesthesia is generally not necessary and does not diminish discomfort caused by uterine penetration.
Under direct sonographic guidance, the needle is introduced with a quick thrust into a pocket of amnionic fluid that is free of fetal parts and umbilical cord (Fig. 13-4). Once the target pocket is reached, the first 2 mL of aspirated amnionic fluid is discarded to prevent maternal cell contamination. Subsequently, 20 to 30 mL of amnionic fluid is withdrawn into a sterile syringe. This fluid is then transferred into sterile tubes and sent for testing. Fetal heart rate is documented before and after the procedure.
Although blind insertion of the sampling needle was the standard in years past, current practice employs continuous sonographic guidance. Visualization should be maintained throughout the procedure to avoid inadvertent fetal puncture and to identify uterine wall contractions, which occasionally will retract the needle tip back into the myometrium (Jeanty, 1990; Lenke, 1985). Direct real-time sonographic guidance allows easier manipulation of the needle if a uterine contraction or fetal movement is encountered (Fig. 13-5).
Operators are cautioned to avoid needle insertion through the maternal bowel to prevent contaminating the uterine cavity. Transplacental passage is also avoided when possible. But if unavoidable, the thinnest portion should be traversed. In these instances, color Doppler is helpful to identify and avoid umbilical vessels at the sampling site. Moreover, the placental cord insertion site contains the largest vessels, and a location away from this area is desirable.
If the initial effort to obtain fluid is unsuccessful, a second attempt in another location can be performed after reevaluation of fetal and placental positions. Amnionic membrane tenting and the development of needle-induced uterine wall contractions are the most frequent cause of initial failure. If the amnion is not yet fused to the chorion at the initial evaluation for amniocentesis, the procedure is best postponed for a few days to a week to improve the success rate of obtaining fluid on the first attempt. Although studies have demonstrated that the fetal loss rate increases with the number of needle insertions, it does not rise with the number of separate procedures. With experienced operators, return visits are rarely needed.
Bed rest is not required after genetic amniocentesis, but the woman should be instructed to avoid heavy lifting and vigorous exercise for 24 hours. Complications after most procedures are uncommon, but women are cautioned regarding potential warning signs. These include fever, fluid leakage, bleeding, or regular contractions. Management of these complications is found on page 210.
In multifetal pregnancy, amnionic fluid from each sac must be sampled individually to provide accurate data for each fetus. Suitable methods employ a multineedle method or a single needle approach. The multineedle technique involves puncture of the first sac and withdrawal of amnionic fluid. Before the needle is removed from the sac, a dilute dye is injected. A new needle insertion then punctures the second twin’s sac (Elias, 1980; Filkins, 1984; Vink, 2012). Retrieval of clear fluid from the second puncture confirms that the first sac was not resampled (Fig. 13-6). If blue-tinged fluid is retrieved, the needle is removed. Another attempt at sampling the second sac is then made.
Of marker dyes, methylene blue is contraindicated because of associated skin staining, small bowel atresia in some studies, and methemoglobinemia risks (Nicolini, 1990a; Van der Pol, 1992). Indigo carmine use has been reviewed in large series by both Cragan (1993) and Pruggmayer (1992) and their colleagues. No increased risk for small-bowel atresia, or any other congenital anomaly, has been found. However, because of theoretic risks posed by an intraamnionic dye, instillation-free techniques have evolved (Jeanty, 1990; Sebire, 1996). This is especially true given the current national shortage of indigo carmine.
Of the instillation-free options, some operators prefer a single-insertion technique. With sonographic guidance, the site of needle insertion is determined by the position of the dividing membrane, and the proximal sac is sampled first (Jeanty, 1990; Vink, 2012). After entry into the first sac and aspiration of amnionic fluid, the needle is advanced through the dividing membrane into the second sac. To avoid contamination of the second sample with fluid from the first, the first 2 mL of fluid from the second sample is discarded. This method may cause iatrogenic rupture of the dividing membrane with creation of a monoamnionic sac and its attendant risk of cord entanglement (Megory, 1991). This complication appears to occur almost exclusively in monochorionic gestations.
Bahado-Singh and associates (1992) described a technique of twin amniocentesis that entails identifying the separating membranes using a curvilinear or linear sonographic transducer. The first needle is inserted, fluid retrieved, and the needle left in place. A second needle is inserted on the other side of the membrane and into the second sac. Visualization of the two needle tips on opposite sides of the membrane confirms sampling from each sac.
Cells within the amnionic fluid are shed from numerous fetal sources, including the lower urinary tract, skin, respiratory tract, gastrointestinal tract, and amnion. After fluid retrieval, cells are placed into tissue culture, either in flasks or on coverslips. After 3 to 7 days of growth, sufficient mitoses are present for staining and karyotype analysis. Cells grown in flasks are harvested and analyzed together by specialized techniques. Cells grown on coverslips are analyzed in situ as individual colonies. Amniocyte culture is reliable, and cells fail to grow in <1 percent of cases.
This newer technique identifies microdeletions and duplications that are not detectable by traditional karyotyping and is used in some cases (Fig. 13-7). In fetuses with structural anomalies identified by sonography, CMA can increase the detection rate of chromosomal anomalies by 6 percent compared with conventional karyotyping. Further, the detection rate of chromosomal anomalies rises by 1.7 percent in women whose indication for testing was advanced maternal age or positive screening results (Wapner, 2012). CMA does not identify balanced translocations and triploidies and thus is best used to complement karyotyping. At our center, we employ karyotyping and CMA when fetal structural anomalies are found.
FIGURE 13-7
Chromosomal microarray analysis. A. Actual microarray chip size. B. Each chip contains thousands of cells (squares). C & D. Each cell contains thousands of identical oligonucleotides on its surface, and each cell is unique in its nucleotide content. E. During genetic analysis, a mixture containing tagged fetal DNA is presented to the chip. Complementary DNA sequences bind. F. If a laser is shined on the chip, DNA sequences that have bound will glow. This identifies a matching sequence. (Reproduced with permission from Doody KJ: Treatment of the infertile couple. In Hoffman BL, Schorge JO, Schaffer JI, et al (eds): Williams Gynecology, 2nd ed. New York, McGraw-Hill, 2012.)

As another option, whole-exome sequencing has the potential to isolate chromosomal anomalies not identifiable by either conventional karyotyping or CMA. However, at the moment, whole-exome sequencing is too expensive to be implemented in routine clinical practice.
Two or more cell lines with different karyotypes in a single person are found in approximately 0.1 to 0.3 percent of cases. This chromosomal mosaicism most often results from postzygotic nondisjunction (Hsu, 1984). It can also result from meiotic errors that lead to trisomic rescue. Both are described more fully on page 216. Notably, the observation of multiple cell lines in a prenatal sample does not necessarily mean that the fetus is mosaic, because such results are confirmed in the fetus in only 70 percent of cases (Bui, 1984). One common explanation is pseudomosaicism, in which an abnormality is evident in only one of several flasks or confined to a single colony (Hsu, 1984). In this case, the abnormal cells arise in vitro, are not present in the fetus, and are not clinically important. True mosaicism exists when the same abnormality is present on more than one coverslip colony or in more than one cell culture flask.
Once confirmed as true, the question of whether amnionic fluid mosaicism also involves the fetus may be resolved by karyotyping fetal lymphocytes obtained by FBS (Gosden, 1988). However, this approach may not be valid in all cases. Namely, the mosaic cell line may involve other fetal tissues and have clinical implications, but be excluded from the fetal hematopoietic compartment and not be present in a fetal blood sample (Johnson, 1997). Alternatively, some mosaic results, for example, trisomy 20, occur in the amnionic fluid relatively frequently but are rarely confirmed in the fetus (Johnson, 1997).
Further evaluation of amnionic fluid mosaic results should also include detailed sonographic scanning to assess fetal growth and exclude structural anomalies. If both sonography and fetal blood sampling results are normal, the parents can be reassured that in most cases major chromosomal abnormalities involving the fetus have been excluded (Gosden, 1988).
Genetic counseling is helpful in explaining such technical details to the patient, but parents should be reminded that a small chance of fetal involvement still exists. Because of the complexity of interpreting mosaic amnionic fluid results, consultation with the cytogenetics laboratory and a clinical geneticist is recommended.
Fluorescence in situ hybridization (FISH) permits determination of the number and location of specific DNA sequences. FISH probes are short, fluorescent-labeled DNA sequences that are hybridized to a known location on a specific chromosome. Interphase cells are then evaluated by counting the number of discrete fluorescent signals from each probe. A normal diploid cell queried with a probe for the centromere of chromosome 18 would have two signals, while a trisomy 18 cell would have three signals.
Interphase FISH is beneficial in cases in which rapid results are crucial to subsequent obstetric management. One example is a case of advanced gestational age, in which the decision to intervene for fetal indications would be affected by results. Speed derives from the facts that amnionic fluid does not require culture and that FISH can detect aneuploidies caused by monosomies, some trisomies, certain trisomies associated with robertsonian translocations, triploidy, and other numerical chromosomal abnormalities. In standard practice, probes involving chromosomes 13, 18, 21, X, and Y are used as these are the commonest causes of aneuploidy in liveborn neonates. However, this technology will not routinely detect cytogenetic abnormalities such as mosaics, translocations, or rare aneuploidies (Klinger, 1992; Ward, 1993). FISH probes are also used to evaluate for microdeletions and duplications within specific regions of the genome known to be associated with disease. Examples are DiGeorge/velocardiofacial syndrome, cri-du-chat syndrome, and Angelman disease.
Cramping from uterine contraction is common following amniocentesis and typically lasts 1 to 2 hours. Lower abdominal discomfort may persist for up to 48 hours after the procedure but is seldom severe. Serious complications are rare following amniocentesis. Of these, associated fetal loss is discussed in the next section.
Amnionitis develops in less than 0.1 percent of cases, and septic shock is rare. Etiologies are contamination of amnionic fluid with skin flora or from inadvertent puncture of the maternal bowel (Turnbull, 1983). It may also follow procedure-induced amnion rupture. Postamniocentesis chorioamnionitis can have an insidious onset and frequently presents with flulike symptoms with few early localizing signs. This can evolve into a serious maternal systemic infection unless early aggressive treatment is undertaken. Therefore, a high index of suspicion is necessary.
Treatment is similar to that for other serious obstetric infections. Intravenous broad-spectrum antibiotics are continued until the woman is afebrile for more than 24 hours. At this point, antibiotics may be discontinued.
Another complication, rhesus alloimmunization, develops in approximately 1 percent of Rh-negative women undergoing amniocentesis. As noted on page 202, prophylactic administration of anti-D immunoglobulin following the procedure can help avoided this problem (Golbus, 1979; Hill, 1980; Tabor, 1986).
Amnionic fluid leakage or vaginal bleeding is noted by 2 to 3 percent of women after amniocentesis and is usually self-limited. Unlike spontaneous second-trimester amnion rupture, fluid leakage following amniocentesis usually resolves after a few days of modified activities and pelvic rest. Successful pregnancy outcome after such an event is common (Kishida, 1994). Occasionally, leakage of amnionic fluid will persist throughout pregnancy, but if the amnionic fluid volume remains adequate, a good outcome may be anticipated (NICHD Amniocentesis Registry, 1976; Simpson, 1981).
The safety and accuracy of midtrimester amniocentesis has been documented in numerous studies. Contemporary studies report total postprocedural loss rates up to 28 weeks that range from 1 to 2 percent (Canadian Early and Mid-Trimester Amniocentesis Trial [CEMAT] Group, 1998). Concurrent high-resolution sonographic guidance of the procedure lowers the risk associated with amniocentesis and raises the probability of obtaining a sample. Of other associations, a greater risk of loss is found if 19-gauge or larger needles are used or if the needle is inserted more than twice per procedure. Other factors are puncture of the placenta, a high index MSAFP level, and discolored amnionic fluid (Tabor, 1986). Tabor and associates (2009) also reported that the number of procedures performed by a department had a significant effect on the risk of miscarriage. In departments performing fewer than 500 amniocenteses during the 11-year period, the odds ratio for fetal loss was 2.2 compared with departments performing more than 1500 procedures in the same period. In sum, the American College of Obstetricians and Gynecologists (2016a) cite a procedure-related loss rate after traditional genetic amniocentesis to be as low as 1 in 370 to 900.
Of other potential fetal harms, some early studies found elevated rates of respiratory distress syndrome, neonatal pneumonia, hip dislocation, and talipes equinovarus, that is, clubfoot, in amniocentesis groups (Tabor, 1986; Working Party on Amniocentesis, 1978). However, other studies have not confirmed any of these associations.
In early experiences with amniocentesis, needle puncture of the fetus was reported in 0.1 to 3.0 percent of cases (Karp, 1977; NICHD Amniocentesis Registry, 1978). Moreover, needle puncture has been associated with fetal exsanguination, intestinal atresia, ileocutaneous fistula, fetal limb gangrene, uniocular blindness, porencephalic cysts, patellar tendon disruption, skin dimples, and peripheral nerve damage (Broome, 1976; Epley, 1979; Karp, 1977; Lamb, 1975; Merin, 1980; Rickwood, 1977; Swift, 1979; Therkelsen, 1981; Young, 1977; Youroukos, 1980). Continuous use of sonography to guide the needle minimizes risk of fetal puncture. In experienced centers, this is a rare complication.
No long-term adverse effects have been demonstrated in children undergoing amniocentesis. Baird and coworkers (1994) compared 1296 liveborn children whose mothers had a midtrimester amniocentesis with unsampled controls. With the exception of hemolytic disease due to alloimmunization, the offspring of women who had amniocentesis were no more likely than controls to have a disability during childhood and adolescence.
Women interested in genetic amniocentesis known to be infected with hepatitis B, hepatitis C, or HIV need additional counseling regarding the risk of vertical transmission. With chronic hepatitis B, genetic amniocentesis does not appear to increase the risk of neonatal infection with hepatitis B. These neonates generally receive the hepatitis B vaccine and immunoprophylaxis (HBIG) after delivery. Women with HIV who are not taking highly active antiretroviral therapy (HAART) and women with high viral loads of hepatitis C are counseled on noninvasive screening methods. This is because data on the risk of vertical transmission, especially if the procedure is transplacental, are limited.
Numerous studies have evaluated the risks of second-trimester amniocentesis in twins. Compared with singletons, in which loss rates are approximately 1.0 to 1.7 percent before 28 weeks, the loss rates for twins are somewhat higher. Most series report postprocedural rates between 2 and 5 percent (Table 13-6).
Years of Procedures | Continuous Guidance | No. | Loss to 20 wk (%) | Loss to 28 wk (%) | |
---|---|---|---|---|---|
Pijpers, 1988 | 1980–1985 | N | 83 | 1.2 | 4.8 |
Anderson, 1991 | 1969–1990 | N | 330 | — | 3.6 |
Antsaklis, 1991 | 1978–1988 | N | 53 | 0.0 | 1.9 |
Pruggmayer, 1991 | 1982–1989 | Y | 98 | 6.1 | 8.1 |
Pruggmayer, 1992 | 1981–1990 | Y | 529 | 2.3 | 3.7 |
Wapner, 1993 | 1984–1990 | Y | 73 | 1.4 | 2.9 |
Ghidini, 1993 | 1987–1992 | Y | 101 | 0.0 | 3.0 |
Ko, 1998 | 1986–1997 | Y | 128 | — | 4.5 |
Yukobowich, 2001 | 1990–1997 | Y | 476 | 2.7 | — |
Cahill, 2009 | 1990–2006 | Y | 311 | — | 3.2a |
Although the higher loss rates could demonstrate an increased danger of amniocentesis in twins compared with singletons, it more likely reflects the inherent risks associated with twin gestations. This helps put the increase in postprocedural loss rates into perspective (Pretorious, 1993; Prompelan, 1989). Further support comes from Ghidini and colleagues (1993), who analyzed 101 twins undergoing amniocentesis and compared them with similar unsampled twins recruited in the second trimester. Each group had a loss rate of 2 percent to 25 weeks’ gestation. The total fetal loss rate in the sampled group was 3.5 percent compared with 3.2 percent in the unsampled group.
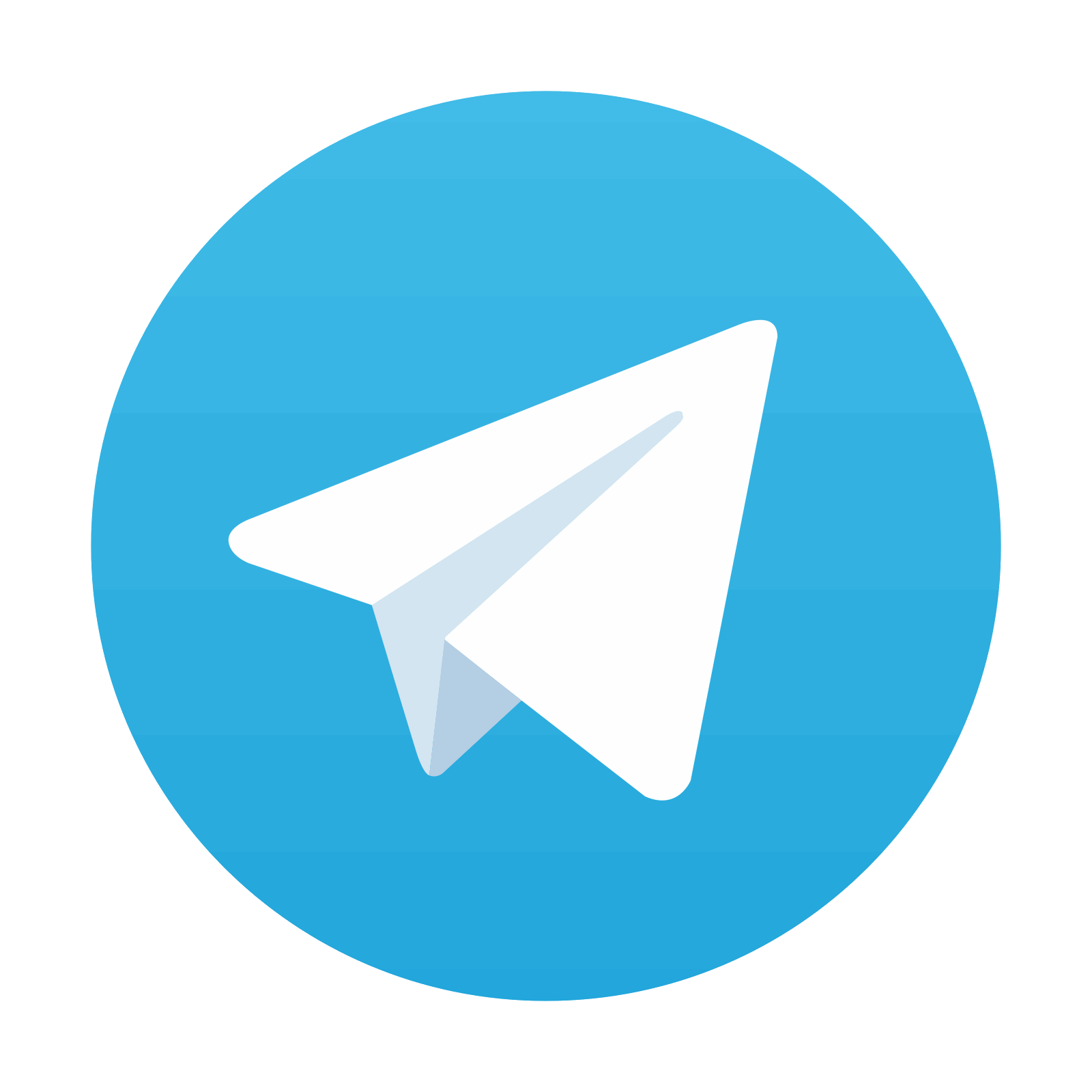
Stay updated, free articles. Join our Telegram channel

Full access? Get Clinical Tree
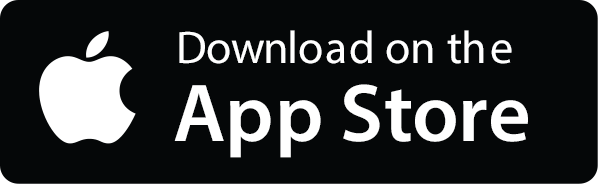
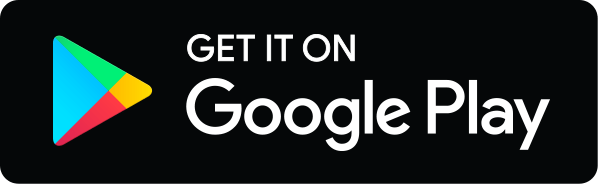