Invasive Prenatal Diagnostic Procedures
The overall objective of invasive prenatal diagnosis is to obtain genetic, biochemical, or physiologic information about the fetus. This chapter describes the most frequently used techniques of amniocentesis, chorionic villus sampling (CVS), and percutaneous umbilical blood sampling (PUBS). This armamentarium of techniques allows detection of an ever-expanding list of inherited conditions. Most perinatal centers have the technical expertise to perform all of these procedures and to select the best procedure for each clinical situation. They also must provide detailed genetic counseling in which available clinical options are described, the risks enumerated, and the consequences communicated in a nondirective, supportive way.
AMNIOCENTESIS
HISTORICAL PERSPECTIVE
Amniocentesis was first used in the 1880s for decompression of polyhydramnios (Lambl, 1881; Schatz, 1992). An additional use became available in 1930 when placental localization was achieved by x-ray analysis after the intra-amniotic injection of contrast medium (Menees and colleagues, 1930). In 1937, Aburel described the intra-amniotic injection of hypertonic saline for termination of pregnancy.
It was during the 1950s that amniocentesis became a significant diagnostic tool when measurement of amniotic fluid bilirubin concentration began to be utilized in monitoring fetal Rhesus disease (Bevis, 1950; Walker, 1957). In that same decade, amniocentesis for fetal chromosome analysis was initiated when laboratory techniques for cell culture and karyotype were developed. While the first reported applications were limited to fetal sex determination by Barr body analysis (Fuchs and Riis, 1956), the feasibility of culturing and karyotyping amniotic fluid cells was demonstrated by Steele and Breg in 1966, and the first prenatal diagnosis of an abnormal karyotype, a balanced translocation, was reported in 1967 (Jacobson and Barter, 1967). Trisomy 21 was first detected prenatally by Valenti and associates in 1968. During that same year the first diagnosis of the metabolic disorder galactosemia was reported (Nadler, 1968).
INDICATIONS FOR AMNIOCENTESIS
EVALUATION FOR FETAL GENETIC ABNORMALITIES.
In the United States, it is standard practice to offer prenatal cytogenetic analysis to all women who will be 35 years of age or older at their expected time of delivery. This approach is based on the increased risk of numerical chromosomal abnormalities (aneuploidy) seen with advancing maternal age, which occurs as a result of nondisjunction during maternal meiosis. The use of age 35 at expected date of conception (EDC) as a cut-off for offering invasive testing is arbitrary. It was chosen over 30 years ago as a pragmatic balance between the available laboratory resources, the relatively limited information about the safety of the emerging technology of amniocentesis, and the fetal genetic risks. As seen in Table 27-1 (Hook and colleagues, 1983; Hook, 1981), this cut-off still seems a reasonable balance between the 1:200 risk of a chromosome abnormality and the known risks of the amniocentesis procedure. Presently, approximately 7.5 percent of pregnant women will be of advanced maternal age, and about 30 percent of Down syndrome births will occur in this population. Because the majority of Down syndrome births are to women under 35, maternal age alone is an inefficient screening approach.
TABLE 27-1. Relationship Between Maternal Age and the Estimated Rate of Chromosomal Abnormalities*
Over the last decade, maternal serum screening utilizing α-fetoprotein (AFP), human chorionic gonadotrophin (HCG), and unconjugated estriol (μEol) levels have been developed to identify pregnancies at risk for Down syndrome and trisomy 18 in the general population (Haddow and colleagues, 1992). Women are considered “screen-positive” if their combined age and biochemical risk for Down syndrome exceeds the second-trimester risk of a 35-year-old woman (risk 1:270). In women under 35 years old, 60–70 percent of Down syndrome pregnancies will be identified with 5 percent of women being screen-positive (Haddow and colleagues, 1992). A positive maternal serum screen is now the second most frequent indication for amniocentesis behind maternal age.
Serum screening is also accurate in women over 35 years old and can identify 85–90 percent of Down syndrome pregnancies with a 25 percent screen-positive rate (Haddow and colleagues, 1994). While abandoning maternal age as an indication for amniocentesis would significantly decrease invasive testing, concerns about missing chromosomally abnormal pregnancies in these women has lead to the continued recommendation that advanced maternal age (AMA) remain an indication for testing. However, many AMA women now await their serum screen risk analysis before deciding on invasive testing.
Ultrasound identification of fetal structural anomalies is increasing in frequency as an indication for amniocentesis. In addition to major structural defects that have long been known to have a high association with fetal aneuploidy, more subtle markers, such as echogenic fetal bowel and mild proximal limb shortening, have been demonstrated to increase the risk of aneuploidy (Rotmensch, 1997; Nyberg, 1993; and their colleagues). In addition, ultrasound markers for fetal infection, anemia, or other disorders frequently require evaluation by amniocentesis.
Amniocentesis is indicated to obtain fetal material for biochemical or DNA studies. The molecular abnormalities responsible for many disorders are being identified at an increasingly rapid rate and any listing of these is soon outdated. A list of the more common genetic conditions for which DNA-based prenatal diagnosis is available is given in Table 27-2. A more detailed list, as well as a listing of the centers performing each test, can be found at www.genetests.org. Many of these conditions are rare and their diagnosis complex so that consultation with a genetics unit is encouraged prior to performing an invasive test.
TABLE 27-2. List of Common Conditions in Which
The screening test for open spina bifida (OSB) is a second trimester measurement of maternal serum α-fetoprotein (MSAFP) levels. Although amniocentesis had been the definitive diagnostic test, it has been supplanted by ultrasound in many centers (UK Collaborative Study, 1979) because ultrasound diagnosis has been greatly enhanced by the recognition of associated abnormalities of the skull and brain. These abnormalities can include ventriculomegaly, microcephaly, frontal bone scalloping (lemon sign), and obliteration of the cisterna magna with either an “absent” cerebellum or abnormal anterior curvature of the cerebellar hemispheres (banana sign; (Nicolaides and colleagues, 1986a). Van den Hof and colleagues (1990) reported associated abnormalities of the skull or brain in 129 of 130 fetuses with OSB. This evidence, along with the improved ability of sonography to visualize the fetal spine, has led to a reevaluation of the need for amniocentesis in the work-up of an elevated MSAFP (Shields and colleagues, 1996).
Patients at increased risk for a neural tube defect because of an elevated MSAFP level, a history of a previous child with a neural tube defect, or who are taking antifolate medications, should be referred to centers with sonographers experienced in high-resolution fetal ultrasound. If ultrasound examination demonstrates a normal fetal spine, cranium, and cerebellum, the chance of an undetected spinal abnormality is low. Amniocentesis can be reserved for patients with suspicious ultrasound findings or large MSAFP elevations despite a normal scan, or when there is an inability to adequately visualize fetal anatomy. Some centers calculate a revised neural tube defect risk on the basis of the MSAFP value and a normal ultrasound examination (Nadel, 1990; Richards, 1988; and their colleagues). For example, with an OSB risk of 1 in 170, a normal ultrasound examination may reduce the risk of spina bifida by 90–95 percent, giving a revised risk of approximately 1 in 3400. However, the sensitivity of ultrasound is extremely dependent on the skill of the sonographer, and each center should be aware of its ability to reduce risk.
EVALUATION OF FETAL HEALTH STATUS
Assessment of the severity of fetal hemolytic disease and the need for fetal blood transfusion or early delivery has been traditionally based on amniocenteses and interpretation of the results of spectrophotometric estimation of amniotic fluid bilirubin (Liley, 1961). This evaluation is less accurate in the midtrimester, and direct measurement of the fetal hemoglobin concentration by PUBS may be required (Nicolaides, 1986b; Nicolaides, 1988; and their colleagues). Recently, ultrasound has been demonstrated to add additional information about the fetal status, which may result in a decrease in the need for invasive testing (Mari and colleagues, 2000).
Premature delivery is a leading cause of perinatal death and chronic handicap. An association between intrauterine infection, premature labor (in the presence or absence of ruptured membranes), and long-term sequelae has been demonstrated (Yoon and colleagues, 2000). Because of this, the use of amniocentesis to evaluate for subclinical infection is gaining acceptance as part of the evaluation of patients with preterm labor. However, until more definitive data is available this must be considered a research tool. However, amniocentesis is indicated in preterm labor and premature rupture of membranes (PROM) for those patients with clinical findings suspicious for infections.
Measurement of the amniotic fluid lecithin/sphingomyelin ratio (Gluck and colleagues, 1974) and phosphatidylglycerol level (Hallman and colleagues, 1976) is useful for the assessment of fetal lung maturity. However, the need for these tests has declined because of improved neonatal care and the introduction of better methods of ultrasound assessment of gestational age and fetal surveillance. Generally, elective preterm delivery should not be performed without a significant maternal or fetal indication; therefore, assessment of fetal lung maturity adds little to the decision-making process.
TECHNIQUE OF AMNIOCENTESIS
Midtrimester amniocentesis for genetic evaluation is most commonly performed between 15 and 18 weeks gestation. At this age, the amount of fluid is adequate (approximately 150 mL) and the ratio of viable to non-viable cells is greatest. Prior to the procedure, an ultrasound scan is performed to determine the number of fetuses, to confirm gestational age, to assure fetal viability, to document normal anatomy, and to locate the placenta and cord insertion. After an appropriate sampling path is chosen, the maternal abdomen is washed with antiseptic solution. Continuously guided by ultrasound, a 20–22-gauge needle is then introduced into a pocket of amniotic fluid free of fetal parts and umbilical cord (Fig. 27-1). The pocket should be large enough to allow advancement of the needle tip through the free-floating amniotic membrane that may occasionally obstruct the flow of fluid. The first 2 mL of aspirated amniotic fluid are discarded to prevent maternal cell contamination of the tissue culture, and then 20–30 cc of amniotic fluid is withdrawn. Fetal heart rate and activity are documented immediately following the procedure.
FIGURE 27-1. Representation of amniocentesis. Note sonographic direction to avoid placental puncture and to avoid direct fetal puncture. (Reprinted with permission from D’Alton and associates, 1994.)
Transplacental passage should be avoided when possible, but if unavoidable the thinnest portion should be perforated. If the placenta must be traversed, color Doppler is helpful in avoiding any large fetal vessels at the sampling site. The area close to the placental cord insertion should be avoided because it contains the largest vessels. Using this approach, transplacental amniocentesis does not appear to increase fetal loss rates in the hands of experienced operators (Bombard and colleagues, 1995).
Although blind insertion of the sampling needle was the standard in the past, current expertise has progressed to the point that amniocentesis should only be performed using continuous ultrasound guidance. Guidance should be maintained throughout the procedure to avoid inadvertent puncture of the fetus, and to identify uterine wall contractions that occasionally will retract the needle tip back into the myometrium. Ultrasound guidance may be performed with either sector or curved-linear-array transducers, and the procedure may be performed either free-hand or with needle guides (Lenke, 1985; Jeanty, 1990; and their colleagues).
The freehand technique allows easier manipulation of the needle if a uterine contraction or fetal movement abruptly alters the position of the target (Fig. 27-2). Alternatively, a needle guide allows more certain ascertainment of the needle entry point and a more precise preentry determination of the sampling path. A needle guide is especially helpful for relatively inexperienced operators or sonographers. Most guides now allow easy intraoperative removal of the needle from the guide, and quick adaptation to free-hand guidance once the uterus has been entered.
FIGURE 27-2. Ultrasound image of amniocers tesis showing the highly reflective needle and the very bright image created by the tip of the needle.
If the initial attempt to obtain fluid is unsuccessful, a second attempt in another location should be performed after reevaluation of the fetal and placental positions. Amniotic membrane tenting and the development of needle-induced uterine wall contractions are the most frequent cause of initial failure. No more than two attempts should be made at any single session, but the patient may be rescheduled in several days. Although studies have demonstrated that the fetal loss rate increases with the number of insertions, it does not increase with the number of separate procedures. In experienced centers, return visits are rarely required.
TECHNIQUE OF AMNIOCENTESIS IN MULTIFETAL GESTATIONS
Amniocentesis in multifetal pregnancy involves puncture of the first sac, withdrawal of amniotic fluid, injection of a dye, and then a new needle insertion to puncture the second sac (Elias, 1980; Filkins, 1984; and their colleagues). If the fluid aspirated after the second puncture is clear, this is confirmation that the first sac was not resampled (Fig. 27-3). If blue-tinged fluid is retrieved, the needle should be removed and another attempt at sampling the second sac should be made.
FIGURE 27-3. Representation of one method of performing amniocentesis in twins: Step 1. The fluid is aspirated from the first sac. Step 2. Indigo carmine is injected into the first sac. Step 3. Clear fluid is aspirated from the second sac.
History has shown us the possible toxic effects of dye instillation. Methylene blue is associated with small bowel atresia (Nicolini, 1990a; Van der Pol, 1992; and their colleagues), as well as an increased risk of fetal death (Kidd and colleagues, 1996). Its use is now contraindicated. Use of indigo carmine has been reviewed in large series by both Cragan and colleagues (1993) and Pruggmayer and colleagues (1992), and no increased risk for small-bowel atresia, nor any other congenital anomaly, has been found. However, because of the theoretical risk of intra-amniotic dye, instillation-free techniques have evolved (Jeanty, 1990; Sebire, 1996; and their colleagues).
A single-puncture method has been described in which the site of needle insertion is determined by the position of the dividing membrane (Jeanty and colleagues, 1990). After entry into the first sac and aspiration of amniotic fluid, the needle is advanced through the dividing membrane into the second sac. To avoid contamination of the second sample with fluid from the first, the first 1 mL of fluid from the second sample is discarded. This method may cause iatrogenic rupture of the dividing membrane with creation of a monoamniotic sac and its attendant risk of cord entanglement (Megory and colleagues, 1991). This appears to occur almost exclusively in monochorionic gestations.
Bahado-Singh and colleagues (1992) described a technique of twin amniocentesis that entails identifying the separating membranes with a curvilinear or linear transducer. The first needle is inserted, fluid retrieved, and the needle left in place, while a second needle is inserted into the coexisting sac. Visualization of the two needle tips on alternate sides of the membrane confirms sampling of both fetuses.
LABORATORY CONSIDERATIONS FOR AMNIOCENTESIS
The cells within the amniotic fluid emanate from a number of sources, including the fetal skin, respiratory tract, urinary tract, GI tract, and the amnionic placenta. After fluid retrieval, the cells are put into tissue culture, either in flasks or on coverslips. After 3–7 days of growth, sufficient mitoses are present for staining and karyotype analysis. Cells grown in flasks are harvested and analyzed together, whereas those grown on coverslips are analyzed in situ as individual colonies. Amniocyte culture is quite reliable with failure occurring in less than 1 percent of cases.
Chromosomal mosaicism—the presence of two or more cell lines with different karyotypes in a single person—occurs in approximately 0.1–0.3 percent of cases and occurs most frequently as a result of postzygotic nondisjunction (Hsu and Perlis, 1984). Mosaic results can also result from meiotic errors with trisomic rescue. The observation of multiple cell lines in a prenatal sample does not necessarily mean that the fetus is mosaic because the results are confirmed in the fetus in only 70 percent of cases (Bui and colleagues, 1984). The most common finding is pseudomosaicism (Hsu and Perlis, 1984), in which an abnormality is evident in only one of several flasks or confined to a single colony. In this case, the abnormal cells arise in vitro, are not present in the fetus, and are not clinically important. True mosaicism exists when the same abnormality is present on more than one cover slip or in more than one flask. While this is rare (0.25 percent of cases), it is clinically important (Hsu and Perlis, 1984). The question of whether amniotic fluid mosaicism also involves the fetus may be resolved by karyotyping fetal lymphocytes obtained by PUBS (Gosden and colleagues, 1988). However, this approach may not be valid in all cases because the mosaic cell line may involve other fetal tissues and have clinical implications, but be excluded from the fetal hematopoietic compartment and not be present in a fetal blood sample (Johnson and Wapner, 1997). Alternatively, some mosaic results (eg, trisomy 20) occur in the amniotic fluid relatively frequently but are rarely confirmed in the fetus (Johnson and Wapner, 1997). Further evaluation of amniotic fluid mosaic results should also include detailed ultrasound scanning to assess fetal growth and exclude structural anomalies. If both ultrasound and fetal sampling are normal, the parents can be reassured that in most cases major chromosomal abnormalities involving the fetus have been excluded (Gosden and colleagues, 1988). They should be reminded, however, that a small chance of fetal involvement still exists. Because of the complexity of interpreting mosaic amniotic fluid results, consultation with the cytogenetics laboratory and a clinical geneticist is recommended.
More than 100 abnormalities of lipid, mucopolysaccharide, amino acid, and carbohydrate metabolism are amenable to prenatal diagnosis from the study of cultured amniotic fluid cells. However, the earlier diagnosis and the substantially larger amount of tissue obtained by chorionic villus sampling may make it the preferred method of diagnosing this group of disorders.
USE OF FLUORESCENCE IN SITU HYBRIDIZATION IN PRENATAL DIAGNOSIS
Fluorescence in situ hybridization (FISH) permits determination of the number and location of specific DNA sequences. FISH probes are relatively short, fluorescently labeled DNA sequences that are hybridized to a known location on a specific chromosome. Interphase cells are then evaluated by counting the number of discrete fluorescent signals from each probe. A normal diploid cell queried with a probe for the centromere of chromosome 18 would have two signals, while a trisomy 18 cell would have three signals.
Prenatal interphase evaluation of uncultured amniotic fluid can detect aneuploidies caused by monosomies, free trisomies, trisomies associated with Robertsonian translocations, triploidy, and other numeric chromosomal abnormalities. In standard practice, probes involving chromosomes 13, 18, 21, X, and Y are utilized. This technology will not routinely detect cytogenetic abnormalities such as mosaics, translocations, or rare aneuploidies (Ward, 1993; Klinger, 1992; and their colleagues).
Ward and colleagues (1993) described the first large-scale application of interphase FISH for prenatal diagnosis. FISH was performed on physician request as an adjunct to routine karyotyping in 4500 patients. Region-specific DNA probes to chromosomes 13, 18, 21, X, and Y were used to determine ploidy by analysis of signal number in hybridized nuclei. A sample was considered to be euploid when autosomal probes generated two hybridization signals and a normal sex chromosome pattern in 80 percent or more of the hybridized nuclei. A sample was considered to be aneuploid when 70 percent or more of hybridized nuclei displayed the same abnormal hybridization pattern for a specific probe. Of the attempted analyses, 90 percent met these criteria and were reported as “informative” to referring physicians within 48 hours.
The overall detection rate for aneuploidy was 73 percent (107/146). The accuracy of informative results for aneuploidies was 93.9 percent (107/114). There was one false-positive sex chromosome result and seven false-negative autosomal aneuploidies. The strict criteria used to define results in the pilot study and to report samples as abnormal, led to a detection rate of only 73 percent of all aneuploidies. If the criteria for reporting a sample as abnormal were changed to include all samples in which greater than 50 percent of hybridized nuclei were trisomic, the aneuploidy detection rate would improve to 83 percent (107 + 14/146). In a recent abstract, Carelli and colleagues (1995) updated this information by presenting data on 13,883 clinical specimens. They found interphase FISH to be informative in 89.2 percent of cases with a false-positive rate of 0.008 percent and a false-negative rate of 1.5 percent. Technologic refinements and increased experience increased their accuracy to 100 percent in their most recent 2906 patients with a detection rate of 93 percent. Even though Bryndorf and colleagues (1997) used the same probe set, they were unable to reproduce these results. Most recent studies support that FISH can routinely diagnose the common trisomies with close to 100 percent sensitivity; however, bloody samples and samples from pregnancies beyond 25 weeks may be less-efficiently evaluated.
The question of which pregnancies should be studied with FISH is debatable. Presently, it is suggested that FISH analysis not be used as a primary screening test on all genetic amniocenteses because of its inability to detect many structural rearrangements. Evans and colleagues (1999) surveyed the results of almost 73,000 prenatal cases from seven centers and reported that routine FISH would have detected only 67 percent of abnormalities. However, it should be noted that this interpretation may be somewhat misleading in that some of the missed abnormalities would not have had an impact on fetal development. All abnormalities would be detectable by subsequent tissue culture, raising the question of whether the FISH analysis is cost effective. Presently, most labs use FISH to offer quicker reassurance to patients with an unusually high degree of anxiety, or to test fetuses at the highest risk such as those with ultrasound anomalies. It is also beneficial in cases where rapid results are crucial to subsequent management such as advanced gestational age.
COMPLICATIONS OF AMNIOCENTESIS
A common finding following amniocentesis is cramping lasting for 1–2 hours. Lower abdominal discomfort may occur for up to 48 hours after the procedure, but rarely is severe. Fortunately, serious maternal complications such as septic shock are rare following amniocentesis. Amnionitis occurs in 0.1 percent of cases (Turnbull and MacKenzie, 1983) and can occur from contamination of the amniotic fluid with skin flora or from inadvertent puncture of the maternal bowel. It may also follow procedure-induced amnion rupture. Postamniocentesis chorioamnionitis can have an insidious onset, and frequently presents with flu-like symptoms with few early localizing signs. This can evolve into a serious maternal systemic infection unless early aggressive treatment is undertaken. Therefore, a high index of suspicion is necessary.
The development of rhesus isoimmunization will occur in approximately 1 percent of Rh-negative women undergoing amniocentesis (Tabor, 1986; Golbus, 1979; Hill, 1980; and their colleagues), but can be avoided by prophylactic administration of anti-D immunoglobulin following the procedure.
Amniotic fluid leakage or vaginal bleeding is noted by 2–3 percent of patients after amniocentesis, and is usually self-limited. Unlike spontaneous second trimester amnion rupture, which has a dismal prognosis, fluid leakage following amniocentesis usually resolves after a few days of bedrest. Successful pregnancy outcome after such an event is common (Kishida and colleagues, 1994). Occasionally, leakage of amniotic fluid will persist throughout pregnancy (NICHD Amniocentesis Registry, 1976; Simpson and colleagues, 1981), but if the amniotic fluid volume remains adequate, a good outcome may be anticipated.
PREGNANCY LOSS FOLLOWING MIDTRIMESTER AMNIOCENTESIS
The safety and accuracy of midtrimester amniocentesis was documented in the mid-1970s by three collaborative studies performed in the United Kingdom, United States, and Canada. These studies were not randomized; instead, the studies included unsampled matched control groups. The United States and Canadian studies showed similar loss rates (spontaneous abortions, stillbirths, and neonatal deaths) between the two groups. A greater risk of loss occurred with needles of 19 gauge or larger, and with more than two needle insertions per procedure. Both studies reported total post-procedure loss rates of 3–4 percent. In contrast to these studies, the British Collaborative Study found an excess of 1–1.5 percent in fetal loss in the amniocentesis group as compared to controls. This study has been criticized for a number of concerns, including a significant proportion of sampled patients with elevated MSAFP levels, an unusually low complication rate in the control group, and a change in the matching criteria during the study (Medical Research Council, 1977; Working Party on Amniocentesis, 1978; NICHD Amniocentesis Registry, 1978).
The only prospective, randomized, controlled trial evaluating the safety of second trimester amniocentesis is a Danish study, which reported on 4606 low-risk, healthy women, 25–34 years old, who were randomly allocated to either amniocentesis or to ultrasound examination (Tabor and colleagues, 1986). The total fetal loss rate in the amniocentesis group was 1.7 percent and in the controls 0.7 percent (p < 0.01). The observed difference of 1 percent gave a relative risk of 2.3 percent. The conclusions of this study were initially criticized because the original report stated that an 18-gauge needle (which is associated with higher risks than smaller needles) was used. Tabor and colleagues (1988) subsequently reported that they had, in fact, used a 20-gauge needle for most of the procedures. This study also demonstrated significant associations between pregnancy loss and puncture of the placenta, high MSAFP, and discolored amniotic fluid (Tabor and colleagues, 1986). The postprocedure loss rates of 3–4 percent seen in early studies are higher than those presently seen because they represent the experience when amniocentesis was a relatively new procedure. Additionally, ultrasound was not routinely utilized. More contemporary studies report total postprocedure loss rates to 28 weeks between 1 and 2 percent (Canadian Early and Mid-Trimester Amniocentesis Trial [CEMAT] Group, 1998).
Both the UK and Danish studies (Working Party on Amniocentesis, 1978; Tabor and colleagues, 1986) found an increase in respiratory distress syndrome and pneumonia in neonates from the amniocentesis groups. Other studies have not found this association. The UK study also showed an increased incidence of talipes and dislocation of the hip in the amniocentesis group (Working Party on Amniocentesis, 1978). This finding has not been confirmed in several other studies.
In early experience with amniocentesis, needle puncture of the fetus was reported in 0.1–3.0 percent of cases (NICHD Amniocentesis Registry, 1978; Karp and Hayden, 1977), and was associated with fetal exsanguination (Young and colleagues, 1977); intestinal atresia (Therkelsen and Rehder, 1981; Swift and colleagues, 1979); ileocutaneous fistula (Rickwood, 1977); gangrene of a fetal limb (Lamb, 1975); uniocular blindness (Merin and Beyth, 1980); porencephalic cysts (Youroukos and colleagues, 1980); patellar tendon disruption (Epley and colleagues, 1979); skin dimples (Broome and colleagues, 1976); and peripheral nerve damage (Karp and Hayden, 1977). Continuous use of ultrasound to guide the needle minimizes needle puncture of the fetus, and in experienced centers, this is an exceedingly rare complication.
No long-term adverse effects have been demonstrated in children undergoing amniocentesis. Baird and colleagues (1994) compared 1296 liveborn children whose mothers had a midtrimester amniocentesis to unsampled controls. With the exception of hemolytic disease due to isoimmunization, the offspring of women who had amniocentesis were no more likely than controls to have a disability during childhood and adolescence. Finegan and colleagues (1990) reported an increased incidence of middle-ear abnormalities in children whose mothers had amniocentesis.
COMPLICATIONS OF AMNIOCENTESIS IN MULTIPLE GESTATION
Multiple studies have evaluated the risks of second trimester amniocentesis in twins. Compared to singletons, in which loss rates are approximately 1.0–1.7 percent before 28 weeks, the loss rates for twins are somewhat higher. Most series report postprocedure loss rates between 2 and 5 percent (Table 27-3). The largest single-center experience is that of Anderson and colleagues (1991), who collected data on over 300 twin sets over a 20-year period. The pregnancy loss rate for twins following amniocentesis was 3.6 percent, as compared to a 0.6 percent rate for singletons sampled over the same period. A 2.3 percent loss rate before 20 weeks and 3.7 percent before 28 weeks was reported by Pruggmayer and colleagues (1992) from a collective European experience of 529 sampled twin sets. Although the higher loss rates could demonstrate an increased danger of amniocentesis in twins as compared to singletons, it more likely is a manifestation of the inherent risk of twin gestations.
TABLE 27-3. Pregnancy Outcomes Following Twin Amniocentesis
Literature evaluating the risk of pregnancy loss prior to 28 weeks in unsampled twins with a normal second trimester ultrasound demonstrates rates of 4.5 percent, 5.8 percent, and 7.2 percent, which helps put the increase in postprocedure rates into perspective (Prompelan, 1989; Pretorious, 1993; and their colleagues). However, the ideal way to evaluate procedure-induced loss rates is to compare similar cohorts of sampled and unsampled twins. Ghidini and colleagues (1993) reported on 101 twins undergoing amniocentesis and compared them to a contemporaneously collected group of unsampled twins recruited in the second trimester. Each group had a loss rate of 2 percent to 25 weeks, and the total fetal loss rate in the sampled group was 3.5 percent as compared to 3.2 percent in the unsampled group. Unfortunately, this evaluation had insufficient power to demonstrate whether the identified difference in fetal loss was significant. No study presently exists with sufficient numbers of patients and controls to better evaluate the procedure-related risks.
Patients with twins that choose to undergo amniocentesis should be counseled prior to sampling about the rate of postprocedure loss, and should be reassured that in most cases this does not appear to be related to the procedure. In this manner, when background losses do occur, the natural tendency of the patient to feel guilty is minimized. Presently, the best estimate of procedure-induced loss from a second-trimester amniocentesis performed in an experienced center is approximately 1 percent.
Patients with twins must also be counseled about the risk of finding a karyotypically abnormal child, which, because of the presence of two fetuses, is approximately twice that following a singleton procedure (Rodis and colleagues, 1990). Amniocentesis in twins does raise some very painful questions. Families need to consider the possibility of a test showing that one of the twins is normal and the other has an abnormality. Selective termination of the affected fetus is now a routine procedure and can be accomplished in 100 percent of cases with a postprocedure loss rate of 5–10 percent (Evans and colleagues, 1999). However, this approach is also associated with an increased risk of preterm birth; especially when performed after 20 weeks gestation, or if the lower fetus is terminated (Lynch and colleagues, 1996).
EARLY AMNIOCENTESIS
The desire for a first trimester diagnosis stimulated interest in the feasibility of performing amniocentesis earlier in gestation. The technique varies somewhat from conventional amniocentesis in that less fluid is available and incomplete fusion of the amnion and chorion is common before 14 weeks’ gestation (Canadian Early and Mid-Trimester Amniocentesis Trial Group, 1998). Therefore, tenting of the free-floating membranes may hamper aspiration of fluid. Penetration can usually be completed by a sharp thrust of the needle but failure of retrieval occurs in approximately 2–3 percent of cases.
While initial experience with early amniocentesis (EA) was reassuring (Hanson, 1992; Assel, 1992; Penso, 1990; Henry, 1992; and their colleagues), subsequent studies raised serious concerns about its safety. When performed under 13 weeks of gestation there is an increased frequency of culture failure, ruptured membranes, and pregnancy loss as compared to later procedures (Canadian Early and Mid-Trimester Amniocentesis Trial Group, 1998; Sundberg, 1997; Nicolaides, 1994; and their colleagues). Of most concern, however, is that in approximately 1–2 percent of cases, severe fetal talipes equinovarus has occurred. This is a rate 10-fold higher than the expected rate of 1 per 1000 births. These clubfoot anomalies are most likely secondary to procedure-induced fluid leakage because there is a 15 percent incidence of talipes when leakage occurs. For these reasons, amniocentesis should rarely, if ever, be performed before the thirteenth week of gestation. The safety of amniocentesis in weeks 13 and 14 is uncertain and is presently being evaluated in a large international trial. Until its safety can be assured, it is best to delay routine sampling until week 15 or 16 of pregnancy.
CHORIONIC VILLUS SAMPLING
The major drawback of conventional second trimester genetic amniocentesis is the delayed availability of the karyotype, and the increased medical risks of a dilatation and evacuation procedure late in the pregnancy. Furthermore, delaying the procedure until after fetal movement is felt inflicts a severe emotional burden on the patient. Because of these concerns, attempts have been made to move prenatal diagnosis into the first trimester. CVS, which samples the developing placenta rather than penetrating the amniotic membrane, is the most successful approach to date for moving prenatal diagnosis into the first trimester.
HISTORY OF CHORIONIC VILLUS SAMPLING
First trimester prenatal diagnosis is not a new concept. The ability to sample and analyze villus tissue was demonstrated more than 25 years ago by the Chinese, who, in an attempt to develop a technique for fetal sex determination, inserted a thin catheter into the uterus guided only by tactile sensation (Department of Obstetrics and Gynecology, Tietung Hospital of Anshan Iron and Steel Co., 1975). When resistance from the gestational sac was felt, suction was applied and small pieces of villi aspirated. While this approach seems relatively crude by today’s standards of ultrasonically guided invasive procedures, the diagnostic accuracy and low miscarriage rate demonstrated the feasibility of first trimester sampling.
In 1968, Hahnemann and Mohr attempted blind transcervical trophoblast biopsy in 12 patients using a 6-mm-diameter instrument. While successful tissue culture was possible, half of these subjects subsequently aborted. In 1973, Kullander and Sandahl used a 5-mm–diameter fiberoptic endocervoscope with biopsy forceps to perform transcervical CVS in patients requesting pregnancy termination. While tissue culture was successful in approximately half of the cases, two of the subjects subsequently became septic.
In 1974, Hahnemann described further experience with first trimester prenatal diagnosis using a 2.5-mm hysteroscope and a cylindrical biopsy knife. Again, significant complications, including inadvertent rupture of the amniotic sac, were encountered. By this time, the safety of midtrimester genetic amniocentesis was well established and further attempts at first trimester prenatal diagnosis were temporarily abandoned in the Western Hemisphere.
Two technologic advances occurred in the early 1980s that allowed reintroduction of CVS. The first advance was the development of real-time sonography, making continuous guidance possible. At the same time, sampling instruments were miniaturized and refined. In 1982, Kazy and associates reported the first transcervical CVS performed with real-time sonographic guidance. That same year, Old (1983) reported the first trimester diagnosis of β-thalassemia major using DNA from chorionic villi obtained by sonographically guided transcervical aspiration with a 1.5-mm–diameter polyethylene catheter. Using a similar sampling technique, Brambati and Simoni (1983) diagnosed trisomy 21 at 11 weeks’ gestation.
Following these preliminary reports, several CVS programs were established in Europe and the United States, with the outcomes informally reported to a WHO-sponsored registry maintained at Jefferson Medical College. This registry, along with single-center reports, were used to estimate the safety of CVS until 1989, when two prospective multicentered studies, one from Canada (Canadian Collaborative CVS/Amniocentesis Clinical Trial Group, 1989), and one from the United States (Rhoads and colleagues, 1989) were published and confirmed the safety of the procedure.
PROCEDURE-RELATED ANATOMY
Between 9 and 12 weeks after the last menstrual period, the developing gestation does not yet fill the uterine cavity (Fig. 27-4). The sac is surrounded by the thick, leathery chorionic membranes within which is both the amniotic cavity and the extraembryonic coelom. The amniotic cavity contains the embryo and is enclosed by the thin, wispy, freely mobile amniotic membrane. The extraembryonic coelom is located between the amniotic and chorionic membranes, contains a tenacious mucoid-like substance, and disappears as the amniotic sac grows toward the chorion and the two membranes become juxtaposed.
Prior to 9 weeks, chorionic villi cover the entire outer surface of the gestational sac. As growth continues, the developing sac begins to fill the uterine cavity and the majority of the villi regress except at the implantation site where they are associated with the decidua basalis (Fig. 27-4). Villi in this area rapidly proliferate to form the chorion frondosum, or fetal component of the placenta. Between 9 and 12 weeks gestation, they float freely within the blood of the intervillus space, and are only loosely anchored to the underlying decidua basalis.
FIGURE 27-4. Diagram of first trimester pregnancy illustrating relevant anatomic landmarks.
TECHNIQUE OF TRANSCERVICAL CVS
Ultrasound examination immediately before the procedure confirms fetal heart activity, appropriate growth, and location of the placenta (Fig. 27-5
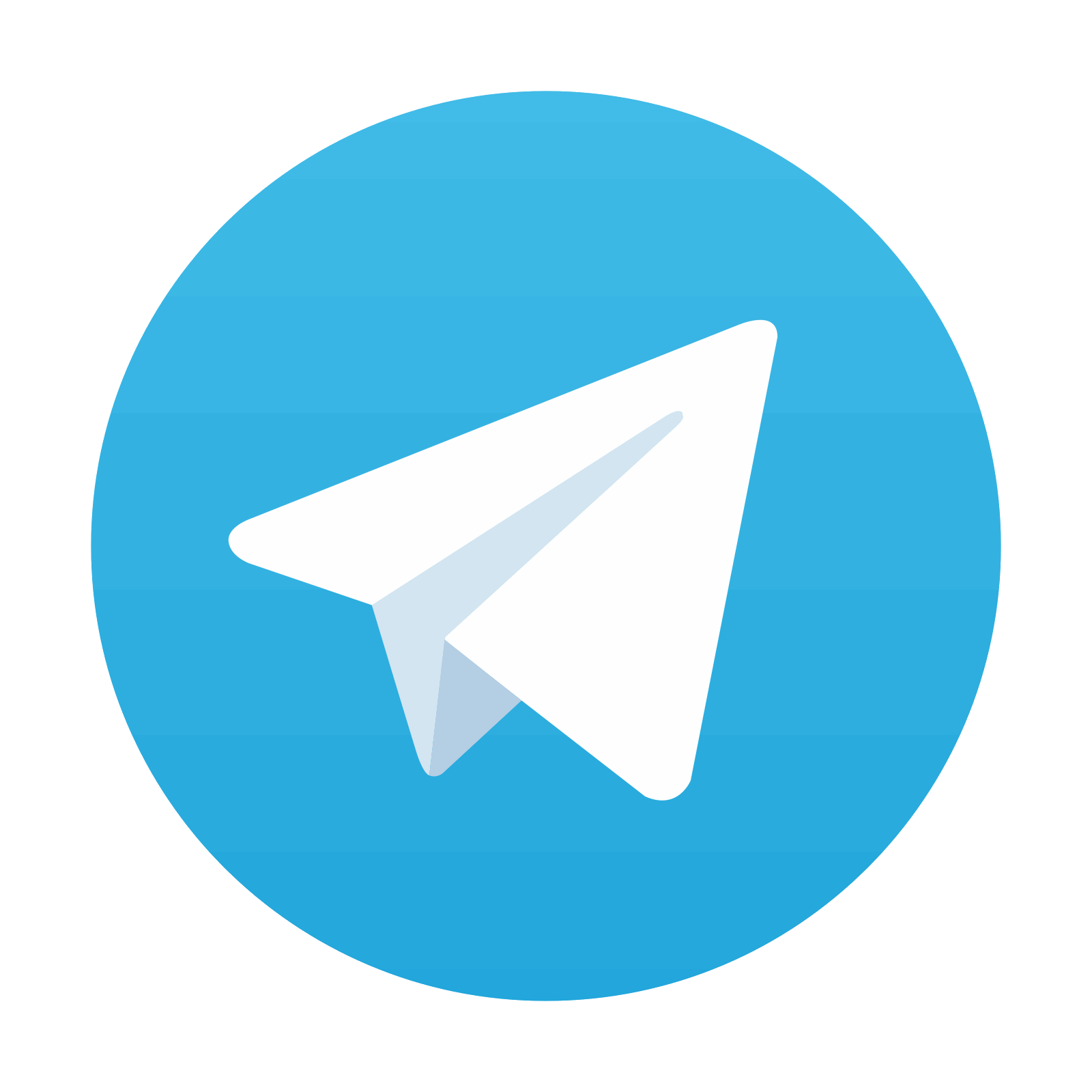
Stay updated, free articles. Join our Telegram channel

Full access? Get Clinical Tree
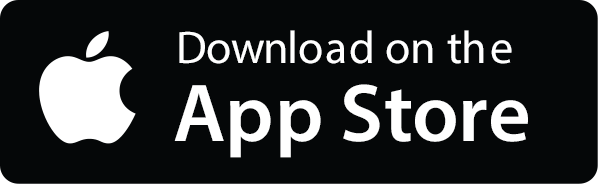
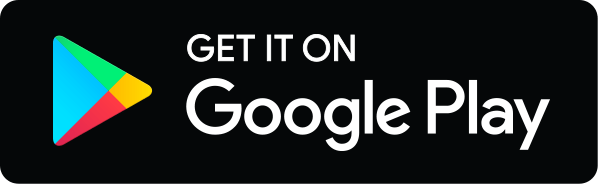