Developmental abnormalities that result in absence or narrowing of intracranial vascular structures are common. However, collateral pathways prevent functional brain alterations in most individuals with these anomalies. Agenesis refers to complete failure of embryological development. Aplasia indicates lack of development despite the presence of a precursor. Hypoplasia is incomplete development. Agenesis and aplasia of vascular structures usually cannot be differentiated radiographically or clinically, and the 2 terms are generally used interchangeably.
Agenesis or hypoplasia of an internal carotid artery occurs in less than 0.01% of the population. There is a 3:1 left-sided predominance. The anomaly is most often unilateral. Absence of the internal carotid artery is usually an isolated lesion, but can also occur in association with other cervicocerebral vascular anomalies, as in patients with PHACES (posterior fossa malformations, hemangiomas, arterial anomalies, cardiac anomalies and aortic coarctation, eye abnormalities, and a sternal cleft or suprapubic raphe) syndrome (see Figure 13-16). Most patients are asymptomatic or have nonspecific complaints, such as headache. Rare potential clinical manifestations include transient ischemic attacks, hemiplegia, and congenital Horner syndrome. Patients with absence of an internal carotid artery are at increased risk for cerebral aneurysms, although these are usually not evident until adult life.1,2
There are 3 basic patterns of collateralization in patients with congenital absence or substantial hypoplasia of a carotid artery; the developmental stage at which the insult occurs determines the specific pattern. (1) By far the most common pattern of collateralization is by way of the circle of Willis. Typically, the contralateral internal carotid artery provides flow to the anterior cerebral artery via a prominent anterior communicating artery. An enlarged posterior communicating artery supplies flow to the middle cerebral artery ipsilateral to the absent carotid artery. (2) If the embryonic insult occurred prior to completion of the circle of Willis, primitive pathways of collateral circulation prevail. This most often consists of an intercavernous communication between the ipsilateral carotid siphon and the cavernous segment of the contralateral internal carotid artery. (3) Rarely, collateral flow occurs into a reconstituted internal carotid artery via skull base collaterals from the external carotid artery system. Potential pathways for arterial supply to the ophthalmic artery in patients with absence of the internal carotid artery are external carotid branches, a residual hypoplastic segment of the internal carotid artery, and various anomalous origins of the ophthalmic artery (e.g., from the posterior communicating, middle meningeal, or anterior cerebral artery).3–6
Techniques for the diagnosis of congenital absence of the internal carotid artery include angiography, CT angiography (CTA), and MR angiography (MRA). The presence of the developing internal carotid artery is a prerequisite for formation of the carotid canal (5 to 6 weeks of gestation). Therefore, an absent carotid canal on CT or MR confirms the diagnosis of internal carotid artery agenesis, as opposed to an acquired occlusion. Likewise, a diminutive carotid canal is confirmatory of the diagnosis of hypoplasia rather than acquired narrowing.7
Agenesis, hypoplasia, and duplication anomalies of smaller intracranial arteries are common anomalies that usually are of no clinical significance other than potential limitations in collateral flow in patients with acquired pathology. Aplasia or hypoplasia of the A1 segment of the anterior cerebral artery is the most common anomaly, occurring in approximately 10% of the population. Duplication of the anterior communicating artery is also common. An azygous anterior cerebral artery refers to a single trunk supplied by the right and left A1 segments (Figure 20-1); this rare anomaly occurs in many children with holoprosencephaly. Hypoplasia of the distal aspect of the basilar artery occurs in some individuals with a fetal origin of the posterior cerebral artery.
Fetal origin of the posterior cerebral artery occurs in approximately 10% of the population. This is due to persistence of the embryonic pattern of circulation. Imaging studies show origin of the posterior cerebral artery from the internal carotid artery (Figure 20-2). The ipsilateral P1 segment is hypoplastic or absent. This anomaly is usually of no clinical significance except in adults with atherosclerotic disease.8
Persistent embryonic circulatory patterns can lead to various anomalous communications between the carotid arteries and the vertebral or basilar arteries. These occur in up to 1% of the population. Most common is a persistent primitive trigeminal artery, which connects the proximal intracavernous portion of the internal carotid artery to the distal third of the basilar artery. The ipsilateral vertebral and posterior communicating arteries are sometimes hypoplastic in individuals with this anomaly. There is a variant of this anomaly in which the persistent trigeminal artery connects with the superior or posterior inferior cerebellar arteries. Potential associated lesions include intracranial aneurysm (posterior communicating artery or the primitive trigeminal artery), arteriovenous malformation (AVM), moyamoya disease, and additional intracranial arterial anomalies.9,10
The second most common persistent carotid-vertebrobasilar anastomosis is the primitive hypoglossal artery. This vessel arises from the internal carotid artery at the C1-C3 level of the neck, courses through the hypoglossal canal, and connects to the basilar artery just beyond its origin. Potential associated conditions include aneurysm at the junction of the vessel with the basilar artery, persistent primitive trigeminal artery, glossopharyngeal neuralgia, and hypoglossal nerve paralysis.
A rare persistent carotid-vertebrobasilar anastomosis is the proatlantal intersegmental artery. This vessel originates from the internal carotid artery or external carotid artery, courses between the occiput and atlas, and connects to the horizontal portion of the vertebral artery near the foramen magnum.
The persistent otic artery arises from the petrous segment of the internal carotid artery, extends through the internal auditory canal, and joins the caudal aspect of the basilar artery. One or both vertebral arteries are hypoplastic or absent in some individuals with this very rare anomaly.
Asymmetry of the venous sinuses of the posterior fossa is common. An absent or hypoplastic transverse sinus is the most common venous sinus “anomaly.” The ipsilateral sigmoid sinus and internal jugular vein are usually small as well in these patients. A dominant right transverse sinus is most common. A small or absent transverse sinus can mimic the findings of venous sinus thrombosis on MR venography. Careful inspection of cross-sectional images is essential to differentiate a clot-filled sinus from a hypoplastic or absent sinus.11
The persistent occipital sinus is an uncommon anomaly that is due to persistence of a fetal venous pathway between the torcula and internal jugular veins. The occipital sinus is a midline vessel dorsal to the cerebellum, contained within the leaves of the dura. In some instances, there are 2 vessels or a network of vessels. The straight sinus and sagittal sinus drain into the persistent occipital sinus at the torcula. At the foramen magnum, the sinus either continues as a single vessel to 1 internal jugular vein or splits into 2 vessels that drain bilaterally. The transverse sinuses are usually small or absent. Recognition of this anomaly is important for patients who will be undergoing posterior fossa decompression surgery.12
Spontaneous, or nontraumatic, intracranial hemorrhage is in important cause of morbidity and mortality in children. The brain parenchyma is the most common site of spontaneous intracranial hemorrhage. Subarachnoid or intraventricular hemorrhage can occur by way of extension from a parenchymal focus or, less commonly, as the primary site of bleeding. Nontraumatic pediatric intraparenchymal hemorrhage occurs at a rate of approximately 0.7 per 100,000 children per year. Common nontraumatic causes of intraparenchymal hemorrhage in children are AVM, cavernous malformation, mycotic aneurysm, venous sinus thrombosis, neoplasm, vasculopathy, and coagulopathy. The major clinical manifestations of spontaneous intraparenchymal hemorrhage relate to elevated intracranial pressure: depressed level of consciousness, headache, irritability, and vomiting. Focal neurological symptoms and seizures are also common.13–17
Spontaneous hemorrhage into the subarachnoid space is rare in children. Greater than 97% of nontraumatic subarachnoid hemorrhages occur in patients older than 18 years. The most common causes are aneurysm (50%) and AVM (25%) (Table 20-1). Fewer than 2% of children with a known aneurysm suffer intracranial hemorrhage prior to the age of 19 years. The risk for hemorrhage is even lower for small lesions; the estimated rate of rupture of aneurysms smaller than 5 mm is 0.55% per year. Spontaneous hemorrhage into the subarachnoid space usually leads to a sudden onset of intense headache and neurological defects of variable severity.18–26
In term infants, the most common cause of spontaneous intracranial hemorrhage is sinovenous thrombosis. Straight sinus thrombosis is an important potential cause of thalamic hemorrhage in the term infant. Thrombosis of the superior sagittal sinus can lead to parasagittal hemorrhage. Spontaneous choroid plexus hemorrhage, usually minor, can lead to intraventricular clot. Hemorrhage due to an AVM or intracranial neoplasm is rare in this age group. In some patients with intracranial hemorrhage, there is no clinically or radiographically demonstrable cause.
In the acute setting, CT is the imaging technique of choice for evaluation of the child with suspected intracranial hemorrhage. An underlying vascular malformation or aneurysm is occasionally visible; contrast-enhanced images or CT angiography may provide more specific information in these patients. MR and MRA are essential components for the evaluation of clinically stable patients with spontaneous intracranial hemorrhage. If these studies fail to reveal a source of hemorrhage and there is a strong clinical suspicion of an AVM or aneurysm, conventional catheter angiography is usually indicated.
An AVM is a congenital nonneoplastic lesion composed of dysplastic thin-walled vessels fed by dilated arteries and drained by enlarged veins. A normal intervening capillary network is lacking. Usually, the dysplastic vessels comprise a “mass”; this is the nidus of the lesion. The pathogenesis involves a localized failure of appropriate differentiation of primitive arteriovenous connections early in embryogenesis. Absence of normal capillaries between arteries and veins leads to high velocity blood flow through the nidus. Enlargement of a congenital arteriovenous malformation may occur by way of progressive dilation of the involved vessels, recruitment of collateral vessels, proliferation of new abnormal vessels, and destruction of adjacent parenchyma. The vascular supply of a cerebral arteriovenous malformation is through pial arteries, dural arteries, or both.27
The most common location of an intracranial arteriovenous malformation in a child is in the subpial space; dural-based lesions and deep cerebral lesions are less common. Approximately 90% of supratentorial arteriovenous malformations are superficial. Superficial lesions often have a triangular shape, with a peripheral base and a central apex. Deep lesions can arise in the thalami or basal ganglia. Eighty percent to 90% of intracranial arteriovenous malformations are supratentorial. Multifocal lesions are uncommon. The predominant blood supply of pediatric intracranial arteriovenous malformations is from the middle cerebral arteries in 55%, the posterior cerebral arteries in 29%, the anterior cerebral arteries in 8.5%, and the internal carotid arteries in 7.5%.26,28
Wyburn–Mason syndrome (Bonnet-Dechaume-Blanc syndrome) is an uncommon phacomatosis in which intracranial arteriovenous malformations (usually multifocal) occur in association with facial nevi and retinal vascular malformations. Multiple arteriovenous malformations of the central nervous system (CNS) can also occur in patients with hereditary hemorrhagic telangiectasia; most of these lesions are pial.29,30
Although these are congenital lesions, most (approximately 80%) arteriovenous malformations are not discovered until adult life. A neonate with a large arteriovenous malformation sometimes has manifestations of hemodynamic deterioration due to massive shunting. A high-flow lesion can also cause clinical manifestations of intracranial venous hypertension. Older children may suffer seizures (usually generalized). Seizures occur in up to 70% of patients with an intracranial arteriovenous malformation, and are the presenting manifestations in approximately 15%. Many patients are asymptomatic until suffering spontaneous hemorrhage; an arteriovenous malformation is the most common cause of spontaneous intracranial hemorrhage in children. Hemorrhage usually arises from the venous side of the lesion, and can occur into the cerebral parenchyma (most common), the subarachnoid space, or the ventricular system. After the initial presenting event, the annual rate of subsequent hemorrhage is approximately 2%.
Chronic or recurrent headache is another potential presenting manifestation intracranial vascular malformations, occurring in approximately 5% of cases. Uncommonly, chronic vascular steal and/or venous hypertension compromise brain development and function, leading to progressive neurological deficits; imaging studies may show encephalomalacia and calcifications. Some infants with an intracranial vascular malformation develop macrocrania, particularly if there is prominent dural venous drainage of the lesion. Occasionally, obstructive hydrocephalus occurs.22,23
On unenhanced CT, an intracranial arteriovenous malformation usually appears as a heterogeneous area of slightly increased attenuation within the brain parenchyma. Small dystrophic calcifications are sometimes present. There may be adjacent hypoattenuation due to encephalomalacia or gliosis. The nidus of the lesion enhances intensely with IV contrast. There are prominent contrast-enhancing arteries and veins adjacent to the nidus. Peripheral lesions often have a triangular or cone-shaped configuration, whereas deep malformations often are rounded. There is usually little mass effect. High-pressure flow through the lesion can lead to the formation of an aneurysm within the nidus, in a feeding artery, or in a draining vein.
Unenhanced CT after hemorrhage from an intracranial arteriovenous malformation most often shows a parenchymal hematoma. There may also be blood in the subarachnoid space or the ventricular system. The hemorrhage sometimes partially or completely obscures the underlying malformation. Spontaneous hemorrhage can also lead to thrombosis of the malformation. Sequential CT imaging performed after intracerebral hemorrhage shows progressive resolution of the hematoma and the development of localized encephalomalacia; dystrophic calcifications may develop.
The rapidly flowing blood within an arteriovenous malformation results in a hypointense appearance on MR (i.e., flow voids). Classically, the nidus appears as a tangle of serpiginous flow voids (Figure 20-3). Hypointense supplying arteries and draining veins extend from the periphery of the nidus. Subacute hemorrhage is hyperintense on T1-weighted images. Hemosiderin from prior hemorrhages may lead to foci within or adjacent to the malformation that are hypointense on T2- and T2*- weighted images. Gliosis is hyperintense on T2-weighted sequences and FLAIR (fluid attenuated inversion recovery) images.31,32
Figure 20–3
Cerebral arteriovenous malformation.
A. A T1-weighted MR image of a 14-year-old child with recent onset headaches shows a superficial “mass” (arrow) composed of multiple flow voids. The lesion has a triangular shape, with the peripheral aspect wider than the central aspect. B. A sagittal T1-weighted image demonstrates hyperintense blood products (arrow) at the inferior-lateral margin of the lesion. C. The nidus of the malformation enhances intensely with intravenous gadolinium. D, E. Anterior and lateral MRA images confirm that the nidus consists of a tangle of vessels with arterial flow (arrow). The predominant feeding arteries arise from the right middle cerebral and posterior cerebral arteries. There is also supply from the right pericallosal artery.


MRA and CT angiography provide supplemental information about the angioarchitecture of an intracranial arteriovenous malformation. Differentiation of arteries from veins is often difficult, as both have rapid flow. Conventional angiography provides the greatest anatomic detail and allows accurate characterization of flow dynamics through the lesion. Rapid sequence image acquisition is essential because of the rapid blood flow. Multiple projections or rotational angiography may be needed to accurately characterize the pathological anatomy. Arteriovenous shunting is a hallmark angiographic feature of an arteriovenous malformation. The supplying arteries and draining veins are dilated and tortuous. The nidus consists of a tangle of small irregular vessels. When present, an associated aneurysm appears as a contrast-opacified pouch. Occasionally, the aneurysm is remote to the actual malformation. Arteriovenous malformations of the dura usually do not have a discrete nidus, but rather consist of multiple microfistulae; that is, a developmental arteriovenous fistula (AVF).21,33,34
An AVF is a large-caliber arteriovenous connection without an intervening capillary bed. The distinguishing feature from an arteriovenous malformation is the lack of a vascular nidus. The connections of an AVF can be single or multiple. AVFs can be developmental (idiopathic) or acquired. The most common types of intracranial AVFs are dural, vertebral, and carotid-cavernous.
A dural AVF is a direct shunt located inside the dural layer. Some dural AVFs are acquired lesions that arise as a consequence of dural sinus thrombosis, trauma, or surgery. In children, however, the idiopathic variety is most common. Idiopathic congenital dural malformations tend to be large high-flow lesions that can clinically mimic vein of Galen malformation. Potential feeding arteries are meningeal vessels (most common), cerebral arteries, internal carotid arteries, and external carotid artery branches. The potential clinical manifestations of dural AVFs include headache, macrocephaly, distended scalp veins, pulsatile tinnitus, proptosis, chemosis, and manifestations related to intracranial hypertension. A large congenital lesion in an infant can lead to cardiac failure. Those AVFs with retrograde cortical venous drainage often cause neurological manifestations. Cortical venous drainage and aneurysmal venous dilation indicate either obstruction of the involved venous sinus or pressure elevation due to large volume blood flow.35–38
Dural AVFs can usually be detected with MRA. Potential findings include multiple curvilinear or nodular vascular structures adjacent to a sinus wall, as well as high signal intensity areas of turbulent high-velocity flow within the venous sinus. Contrast-enhanced digital subtraction MRA documents early filling of the involved venous sinus. Angiography usually demonstrates multiple enlarged meningeal arteries that converge on a venous sinus. Transcranial sonography of the neonate with a congenital dural AVF typically shows marked enlargement of the venous sinuses distal to the lesion. Unlike vein of Galen malformation, the cerebral vessels in these infants are often normal in size or minimally enlarged. Older children with a large dural AVF often have hydrocephalus and imaging manifestations of parenchymal ischemia, such as white matter thinning and gliosis.38
Vertebral AVFs can be congenital, iatrogenic, or posttraumatic. There is a connection, usually solitary, between the vertebral artery and markedly dilated draining veins in the paravertebral plexus. The most common clinical manifestations are neck pain and a bruit. Neurological findings occasionally occur due to vascular steal from the intracranial circulation. Imaging studies demonstrate enlargement of the vertebral artery proximal to the fistula and direct communication with multiple enlarged tortuous draining veins.39
An AVF between an internal carotid artery and the cavernous sinus is usually an acquired lesion due to trauma. Although quite rare, developmental dural AVFs from a meningeal artery can also occur at this site. Potential clinical manifestations of a carotid-cavernous fistula include headache, bruit, proptosis, and diplopia. Neuroimaging studies show 1 or more prominent supplying arteries and marked enlargement of the involved cavernous sinus. There is also dilation of veins that communicate with the involved venous sinus, including the superior ophthalmic vein. Shunting of blood through intercavernous connections often leads to concomitant enlargement of the contralateral cavernous sinus and its tributary veins.40
Vein of Galen malformation is a developmental cerebrovascular lesion in which midline arteriovenous communications feed enlarged deep venous structures of the galenic venous system. This term encompasses a spectrum of anomalies. Most often, the pathogenesis consists of developmental AVFs between deep choroidal arteries and the median prosencephalic vein of Markowski. The arteriovenous communications can be in the form of 1 or more large direct fistulae or multiple smaller connections.41–50
There are various classification schemes for vein of Galen malformations. The vein of Galen aneurysmal malformation refers to a direct AVF into the embryonic median prosencephalic vein. There is lack of embryonic development of a true vein of Galen. The choroidal type of this malformation is most common. This is a complex lesion, with arterial supply from choroidal, pericallosal, thalamoperforator, and transmesencephalic arteries that communicate with tributaries of the median prosencephalic vein. The clinical presentation of the choroidal type typically is in the newborn period and includes manifestations of congestive heart failure. The mural type of vein of Galen aneurysmal malformation involves fewer fistulae than with the choroidal type. Feeding vessels from the collicular arteries, the posterior choroidal arteries, or the pericallosal arcade drain into the wall of the dilated median prosencephalic vein. These patients usually present as infants with macrocephaly and failure to thrive, often accompanied by some degree of congestive heart failure. The vein of Galen aneurysmal dilation refers to a deep midline arteriovenous malformation that drains into the vein of Galen (Figure 20-4). Enlargement of the vein of Galen in these patients is in part due to restriction of outflow. Common supplying vessels include lenticulostriates, thalamoperforators, trans-sylvian branches of the middle cerebral arteries, and perforators from the anterior cerebral arteries. Patients with the vein of Galen aneurysmal dilation usually present beyond infancy with seizures, neurological deficits, or manifestations of intracranial hemorrhage; congestive heart failure is rare in these children.
More than 90% of vein of Galen malformations are detected prenatally or during the neonatal period. There are 3 clinical classifications of vein of Galen malformation: (1) the neonate with a prominent intracranial bruit and congestive heart failure (about one-third of patients); (2) an infant presenting with macrocephaly, prominent scalp veins, or seizures; and (3) an older child presenting with headaches or manifestations of subarachnoid hemorrhage. Occasionally, spontaneous thrombosis results in an acute presentation. With a large shunt, cerebral venous hypertension and ischemia lead to brain damage. Ventriculomegaly in children with vein of Galen malformation often has a multifactorial pathogenesis; potential causes include compression of the aqueduct, intracranial venous hypertension, and brain atrophy.42,45,51
The prenatal sonographic appearance of vein of Galen malformation is that of a “cyst” or prominent vascular structure at the midline dorsal to the thalamus and midbrain. The appearance can mimic that of other developmental cystic lesions such as porencephaly or arachnoid cyst. Doppler evaluation confirms blood flow through the lesion. The neck vessels may appear prominent. With a large lesion, there may be sonographic manifestations of heart failure. The dilated “vein of Galen” usually is not sonographically visible until the third trimester. Fetal MR can also be helpful to document the pathological vascular anatomy and associated alterations in the brain.52
CT angiography and MRA are the most useful noninvasive imaging techniques for demonstrating the morbid vascular anatomy of a vein of Galen malformation. Conventional transcatheter angiography is reserved for therapeutic embolization procedures. The most commonly utilized angiographic classification for vein of Galen malformation recognizes 4 types. The type 1 lesion involves 1 or a few feeding arteries from the pericallosal and posterior cerebral arteries. The type 2 lesion is somewhat more complex, with additional feeders from thalamoperforators. The type 3 lesion has feeding vessels from the pericallosal, posterior cerebral, thalamoperforator, and basilar arteries; lenticulostriate and sylvian arteries can also be involved. The type 4 lesion is an arteriovenous malformation of the thalamus or midbrain, with secondary dilation of an otherwise normal vein of Galen; that is, vein of Galen aneurysmal dilation. In some patients with vein of Galen malformation, there are abnormalities in the major draining veins, such as duplication of the straight sinus, an accessory straight sinus, or stenosis of the straight sinus. If there is hemodynamically significant resistance to drainage through the venous sinuses of the posterior fossa, redistribution into anterior vessels may lead to massive enlargement of facial veins.53
Standard CT and MR examinations demonstrate a vein of Galen malformation as a large mass at the midline in the region of the quadrigeminal plate cistern. The varix may have a spherical or tubular shape. On unenhanced CT, the blood-filled “aneurysm” usually appears slightly hyperattenuating to normal brain. Foci of thrombosis may alter the attenuation characteristics. There is intense contrast enhancement of the lesion, the feeding arteries, and the draining veins. Chronic ischemia may lead to parenchymal hypoattenuating foci of encephalomalacia. Occasionally, hemorrhage or dystrophic calcification results in foci of high attenuation.43,54,55
The rapidly flowing blood in the varix of a vein of Galen malformation is predominantly hypointense on MR sequences (Figure 20-5). A somewhat heterogeneous character is common, reflecting turbulent flow and/or intraluminal clot. There may be pulsation artifact associated with the mass. The feeding arteries appear as linear flow voids adjacent to the varix. The mass causes displacement of the tectum; hydrocephalus is common. There may also be prominence of subarachnoid fluid surrounding the cerebral convexities, due to elevated intracranial venous pressure and diminished cerebrospinal fluid (CSF) resorption. Parenchymal foci of acute ischemia are hyperintense on diffusion-weighted images; in the subacute phase, these become hyperintense on standard T2-weighted images and hypointense on T1-weighted images.
Figure 20–5
Vein of Galen aneurysmal malformation.
Rapidly flowing blood in the varix produces a flow void on this T1-weighted MR image. The posterior extension of the varix is more superior than expected for a dilated straight sinus. The anterior aspect of the varix compresses the tectum. The basilar artery is dilated and tortuous.

A venous angioma is a relatively common developmental lesion composed of radially oriented dilated veins that converge in a single enlarged transcortical draining vein. The brain tissue between the venous channels is normal. The term “angioma” is a misnomer, as this lesion is not a neoplasm. Developmental venous anomaly is a more accurate term for this localized venous malformation. The thin-walled anomalous veins are usually medullary or subcortical. There is no arteriovenous shunting. Most venous angiomas are asymptomatic. Potential clinical manifestations include headache, seizure, and focal neurological deficit. Hemorrhage is rare. Multiple venous angiomas can occur in patients with the blue rubber bleb nevus syndrome.27,56–58
A specific imaging diagnosis of venous angioma is usually possible with MR (preferably performed with IV contrast), contrast-enhanced CT, or cerebral angiography. The key feature is a tuft of small vessels that converge into a single enlarged transcortical draining vein (Figure 20-6). The collection of dilated anomalous veins has been likened to a medusa head (caput medusa). In most instances, the lesion is not visible on unenhanced CT; rarely there is a focus of dystrophic calcification (Figure 20-7). Identification of the draining vein is an important clue to the diagnosis on unenhanced MR images. Because the velocity of blood flow within the lesion is low, the signal intensity on unenhanced MR is variable (Figure 20-8).32,59,60
Figure 20–7
Venous angioma.
A. Unenhanced CT of a 17-year-old boy with headaches shows a small focus of calcification (arrow) in the right frontal lobe. The adjacent parenchyma appears normal. B. A contrast-enhanced T1-weighted MR image is diagnostic, demonstrating a tuft of radially oriented veins (large arrow) that converge into a single prominent draining vein (small arrow).

Venous angiomas occur throughout the brain; periventricular or deep locations are more common than superficial, and supratentorial locations are more common than infratentorial. Drainage can occur into superficial cortical veins or sinuses, into deep periventricular veins, or into both. The draining vein communicates with the superficial venous system in approximately 70% of cases. There is occasionally a stenosis of the draining vein as it enters the dural sinus. Stenosis can lead to localized venous hypertension, draining vein thrombosis, and hemorrhagic complications.
Cavernous malformation is a form of cerebral vascular malformation. As this is not a neoplasm, the synonymous terms cavernous angioma, cavernoma, and cavernous hemangioma are inaccurate. Pathologically, a cavernous malformation is a discrete well-circumscribed cerebral mass with distinct lobulations. Most are 0.5 to 3 cm in diameter. The supplying arteries are of normal size and there is no nidus. Blood flow through the lesion is relatively slow. The lesion consists of endothelial-lined, blood-filled sinusoidal chambers that are separated by fibrous or collagenous tissue; there is no intervening normal brain tissue. Repeated small hemorrhages lead to adjacent gliosis, microcalcification, and small cysts that contain blood breakdown products. Most cavernous malformations are supratentorial. Occasionally, multiple lesions are present; about half of patients with the autosomal dominant familial form of cavernous malformation have multiple foci. Approximately 75% of patients with familial cerebral cavernous malformation have mutations in the CCM2, KRIT1, or PDCD10 genes.27,61–65
Most clinical manifestations a cavernous malformation relate to acute hemorrhage or seizures. Occasionally, there are focal neurological deficits; these are relatively common presenting features in young children. Chronic or recurrent headache occurs in some patients. Some cavernous malformations are entirely asymptomatic; approximately 25% of individuals with this lesion experience no symptoms throughout their lifetimes. Even asymptomatic lesions have histological evidence of prior hemorrhagic episodes. The incidence of spontaneous clinically apparent hemorrhage of an untreated cavernous malformation is probably less than 1% per year. In general, deep (i.e., brainstem and central gray matter) lesions have a higher risk for hemorrhage and neurological deficits then do superficial supratentorial lesions. When feasible, surgical resection is the treatment of choice for cavernous malformation.62,63
Examination with unenhanced CT shows a cavernous malformation as a well-circumscribed irregular nodule of mixed attenuation. At least a portion of the mass is slightly hyperattenuating to brain. There is mild contrast enhancement. Intraparenchymal edema and hemorrhage sometimes obscure the lesion. One or more prior hemorrhagic events may lead to encephalomalacia, calcification, or cysts.
On MR, an uncomplicated cavernous malformation appears as a small mass with well-defined, lobulated borders. Varying signal characteristics are typical. Blood products may result in hyperintensity within portions of the lesion on T1-weighted images (Figure 20-9). There is usually gadolinium contrast enhancement. On T2-weighted or T2*-weighted sequences, hemosiderin within glial cells and macrophages at the periphery of the lesion causes marked hypointensity (Figure 20-10). The remainder of the lesion and any surrounding gliosis are hyperintense on T2-weighted and FLAIR images (Figure 20-11). Enlarged feeding arteries and draining veins are lacking. Circulation through a cavernous malformation is quite slow, and arteriovenous shunting does not occur. Cavernous malformations are often not visible on conventional angiography. Occasionally, there is a faint capillary blush or sedimentation of contrast within the lesion.66–68
Figure 20–10
Familial cavernous malformations.
This asymptomatic 5-year-old girl was evaluated due to a history of cavernous malformations in her mother and maternal aunt. A. There are innumerable hypointense foci in the brain on this T2*-weighted gradient echo sequence. B. The lesions are only faintly visible on a spin echo T2-weighted image. The larger left frontal lobe focus (arrow) has a mixed character on this sequence.

A capillary telangiectasia is a collection of dilated capillaries intermixed with normal brain parenchyma. Hemorrhage from these lesions is rare. Many are asymptomatic. The most common location is the pons. There are often coexistent cavernous angiomas in patients with capillary telangiectasias. Most capillary telangiectasias of the brain are small and solitary; there are rare large or diffuse forms.
On MR evaluation, capillary telangiectasias are small, homogeneously enhancing, hypointense-to-isointense on T1-weighted images, and isointense-to-slightly hyperintense on proton density and T2-weighted images. There is marked signal loss on gradient echo sequences, apparently due to deoxygenated blood within the dilated capillaries. The hemosiderin rim that is typical of cavernous angioma is lacking. There is usually mild-to-moderate contrast enhancement, often with slightly irregular borders.69,70
Posterior fossa vascular abnormalities are occasionally implicated in trigeminal neuralgia. Trigeminal neuralgia is a craniofacial pain syndrome that rarely occurs in children. Approximately 1% of instances of trigeminal neuralgia have onset in patients younger than 20 years. These patients experience episodic unilateral facial pain in the distribution of the trigeminal nerve, most commonly in the maxillary and mandibular distributions. Grimacing during episodes of pain gives rise to the term tic douloureux that describes this condition.71
Trigeminal neuralgia occurs in idiopathic and secondary forms. Deficits of trigeminal function are sometimes identifiable clinically in patients with the secondary form. Some instances of idiopathic trigeminal neuralgia are caused by compression of the nerve near its origin from the brainstem by an anomalous loop of the anterior inferior cerebellar artery. Other potential causes include multiple sclerosis, neoplasm, vascular malformation, and petrous sinusitis. Children presenting with this disorder generally should be evaluated with MRI. Careful evaluation of the course of the trigeminal nerve for a tumor or anomalous vessel is essential. Potential sites of nerve impingement include the posterior fossa, Meckel cave, the cavernous sinus, the skull base, and the pterygopalatine fossa. Symptoms due to trigeminal nerve damage can also occur following head or facial trauma, surgery, or a dental procedure.72,73
Cerebral aneurysms are rare in children. The 3 major types of cerebral aneurysms are congenital, mycotic, and traumatic. The morphological classification of aneurysms is into saccular, fusiform, and dissecting forms. Most congenital intracranial aneurysms are isolated. Cerebral aneurysms occasionally occur in association with a predisposing systemic disease, such as autosomal dominant polycystic kidney disease, coarctation of aorta, fibromuscular dysplasia, Ehlers–Danlos syndrome, Marfan syndrome, or tuberous sclerosis. Aneurysms sometimes occur due to local hemodynamic alterations caused by a vascular anomaly, such as persistent fetal patterns or vestigial remnants. Other associations include moyamoya, HIV infection, and hematological disorders (e.g., sickle cell disease).
Between 1.3% and 1.9% of cerebral aneurysms are diagnosed in patients less than 20 years of age. There is a male predilection. Approximately 40% of pediatric aneurysms are located in a middle cerebral artery and 25% in the posterior circulation. Many congenital cerebral aneurysms in the pediatric age group (particularly in infancy) are giant, that is, greater than 2.5 cm in size.
Most pediatric aneurysms are symptomatic at the time of diagnosis. There is a bimodal distribution of presenting age: The first peak is in infants less than 6 months of age and a second peak occurs from 8 years of age to adolescence. The most common clinical presentation of all types of intracranial aneurysms is due to hemorrhage; in decreasing order of frequency, hemorrhage is subarachnoid (>80%), intraparenchymal, and/or intraventricular. Other common presenting features relate to mass effect, hydrocephalus, or seizures (seizures are uncommon, however, with an unruptured aneurysm). Enlargement of an aneurysm can produce focal neurological findings by way of cranial nerve compression or parenchymal compression. Hydrocephalus or other manifestations of mass effect are relatively common in association with a giant aneurysm.20,74,75
The most common type of intracranial aneurysm in childhood is a congenital saccular aneurysm. The lesion is usually due to a developmental defect in the tunica media and tunica elastica of the arterial wall. The intima bulges through the defect; only adventitia covers the aneurysm peripherally. The imaging features of cerebral aneurysms that occur in association with a predisposing systemic condition are similar to those of true congenital aneurysms.
The most common site of a pediatric congenital intracranial aneurysm is the middle cerebral artery. Sites that are more common in children than in adults include the proximal aspects of the anterior and middle cerebral arteries and the vertebrobasilar system. The circle of Willis is a less common site of origin in children than in adults. Approximately 30% to 35% of congenital aneurysms arise at the internal carotid bifurcations; this site is most common in older children and adolescents. In infants and young children, 30% to 40% of cerebral aneurysms occur in the posterior circulation. Only approximately 5% of children with a congenital intracranial aneurysm have multiple lesions. Aneurysms larger than 2.5 cm account for 16% to 29% of pediatric cases; this is a much higher frequency than in adults. Forty to fifty percent of pediatric congenital intracranial aneurysms are in the 1 to 2.5 cm size range. About half of giant aneurysms in children occur in the anterior circulation and half in the posterior circulation.76
Most congenital intracranial aneurysms are clinically silent until the lesion ruptures. Rupture of a congenital intracranial aneurysm accounts for approximately 50% of atraumatic subarachnoid hemorrhages (Table 20-1). An unruptured aneurysm occasionally produces neurological symptoms due to mechanisms such as mass effect on the second and third cranial nerves or cerebral ischemia resulting from aneurysm thrombosis and embolization. Brain compression by a giant aneurysm can cause focal neurological signs. Common clinical manifestations of aneurysm-related subarachnoid hemorrhage include sudden onset of headache, vomiting, seizures, coma, paresis, cranial nerve palsy, fever, and meningismus. Occasionally, headache occurs as a warning sign of impending major hemorrhage. Headache is uncommon in young children and infants with an aneurysm.77,78
The size and location of a congenital intracranial aneurysm affect the risk for rupture. Smaller lesions and those located in the anterior circulation are less likely to rupture than larger aneurysms and those in the posterior circulation. Management options of an unruptured aneurysm include surgical clipping, endovascular coiling, and observation without intervention (for a small lesion).79
Most infectious (mycotic) aneurysms in children are due to bacterial rather than fungal or protozoan infections. The most common underlying pathology is embolization from bacterial endocarditis. A second type of infectious aneurysm involves direct extension from an adjacent focus, such as meningitis, osteomyelitis, paranasal sinus infection, or cavernous sinus septic thrombophlebitis. This type of aneurysm most often arises from a large artery at the base of the brain. A third form of infectious aneurysm occurs in the absence of a detectable inflammatory lesion elsewhere in the body. The most common organisms involved in bacterial intracerebral aneurysms in children are alpha streptococcus, staphylococcus, Pseudomonas, and Haemophilus species.80,81
Subarachnoid hemorrhage is the most common presenting symptomatic feature of a mycotic aneurysm in childhood. Seizures, intraparenchymal hemorrhage, or neurological alterations related to mass effect or ischemia can also occur. A mycotic aneurysm within the cavernous sinus typically causes various orbital manifestations, such as proptosis, ophthalmoplegia, and venous engorgement.
A traumatic intracranial aneurysm can result from a direct penetrating injury or a closed head injury in which there is acceleration/deceleration-induced shear. The former can be located anywhere along the direct path of the penetrating injury. Aneurysms related to closed head injury tend to occur in the vascular suspensory regions of the brain or areas of vascular contiguity with dural margins. Examples include the anterior cerebral arteries along the falx, the posterior cerebral arteries adjacent to the free edge of the tentorium, and the middle cerebral arteries adjacent to the sphenoid ridges. Peripheral cortical vessels can be damaged at the edge of a skull fracture. The most common traumatic intracranial aneurysms involve the internal carotid arteries; these are classified as cavernous, supraclinoid (rare), and petrous types. Traumatic aneurysms of the anterior cerebral arteries are most often distal to the A1 segments. Traumatic aneurysms of the middle cerebral arteries typically occur at or just distal to a bifurcation. In the posterior circulation, the most common location of a traumatic aneurysm is adjacent to the vertebrobasilar confluence.82,83
Traumatic intracranial aneurysms are true or false. A true aneurysm has an intact layer of adventitia in the wall. A false traumatic aneurysm (i.e., pseudoaneurysm) represents fibrous organization around a communicating perivascular hematoma. Direct penetrating injuries or neurosurgical procedures are the most common causes of false aneurysms. Blunt head trauma and penetrating injuries can also lead to a traumatic aneurysmal AVF. A dissecting aneurysm is a form of true aneurysm; this represents an outpouching beyond the vessel lumen due to a tear in the intima and internal elastic lamina, with dissection of blood subintimally.84
Intracranial hemorrhage is the most common presenting feature of a traumatic cerebral aneurysm. Less common findings in these patients include recurrent epistaxis, cranial nerve palsy, and manifestations of hydrocephalus. Some patients are asymptomatic. Occasionally, there is a substantial delay between the initiating traumatic event and the onset of clinical manifestations of a traumatic cerebral aneurysm.85
Because subarachnoid hemorrhage is the most common presenting feature of an intracranial aneurysm, CT is usually the initial imaging study performed. If large, the aneurysm itself may be visible as a mass, and occasionally mimics the appearance of a neoplasm. In the situation of acute intracranial hemorrhage, the lesion is often a least partially filled with clotted blood. The patent portion of the aneurysm enhances intensely with IV contrast (Figure 20-12). Other potential CT findings include an intracerebral hematoma, intraventricular or subarachnoid hemorrhage, and hydrocephalus. The hemorrhage associated with a cerebral aneurysm sometimes localizes to the basilar cisterns. A partially thrombosed giant aneurysm may appear as a ring-enhancing mass. CT angiography generally provides excellent depiction of the morbid anatomy of a patent cerebral aneurysm. In neonates and young infants, a large cerebral aneurysm is often demonstrable with sonography.25,86
Figure 20–12
Carotid siphon aneurysm in a child with Marfan syndrome.
A. Contrast-enhanced CT shows the lesion as a homogenously enhancing mass (arrow). B. There is enhancement of the aneurysm wall (arrows) on this T1-weighted gadolinium-enhanced MR image. The patent potion of the lesion enhances with contrast, whereas the clot-filled inferior aspect is relatively hypointense. The aneurysm displaces the adjacent left temporal lobe.

On standard MR images, a patent aneurysm appears as an oval or round flow void adjacent to a vessel. Thrombus within the lumen produces greater signal intensity; the specific character varies with the age of the clot. Artifacts from turbulent flow are sometimes present within a giant aneurysm. There is usually intense homogeneous contrast enhancement of a patent cerebral aneurysm. The enhancement pattern typically is ringlike when the aneurysm is thrombosed. MRA provides supplemental information and is an important screening tool for high-risk patients. Contrast-enhanced MRA may improve sensitivity for the detection of a small lesion or an aneurysm with slow flow. A thrombosed aneurysm usually is not visible on MRA.87
Conventional catheter cerebral angiography remains the gold standard for the detection and characterization of intracranial aneurysms. The typical angiographic appearance of an intracranial aneurysm is a saccular outpouching from an arterial wall, often at the bifurcation of a vessel (Figure 20-13). Accurate demonstration of the anatomy at the neck of the lesion often requires multiple projections. Giant aneurysms can be fusiform or saccular; accurate depiction of the communication with the source artery is essential for therapeutic planning. A dissecting aneurysm appears as an elongated, ovoid, or saccular contrast collection that extends beyond the vessel lumen.88
Figure 20–13
Middle cerebral artery aneurysm.
A. Liquid blood within the lesion (arrow) is slightly hyperattenuating relative to brain on this unenhanced CT image. There is a small focus of hyperattenuating clot in the posterior aspect of the aneurysm. B. Because of slow flow within the aneurysm cavity, it is only faintly visible (arrow) on this time-of-flight MRA image. C. The aneurysm (arrows) fills with contrast on this angled AP image from a conventional cerebral angiogram.


Focal arterial spasm or aneurysm thrombosis sometimes prevents contrast opacification of a ruptured aneurysm. Angiography in this situation is often normal or demonstrates subtle irregularity of the vessel wall. These hemodynamic alterations also interfere with visualization on MRA and CT angiography. Follow-up evaluation in a few weeks may allow detection of the aneurysm due to recanalization of the lumen. When multiple aneurysms are present, angiographic clues to the source of subarachnoid hemorrhage include large aneurysm size, a small diverticulum near the apex of the aneurysm, and an irregular contour of the aneurysm wall.89
Arterial vasospasm is a potential sequela of aneurysmrelated subarachnoid hemorrhage that can lead to cerebral ischemia (Figure 20-14), although overt stroke due to vasospasm in children is uncommon. The severity of vasospasm roughly correlates with the volume of blood in the basal cisterns. Typically, vasospasm related to subarachnoid hemorrhage peaks a few-to-several days after the initial event. Transcranial Doppler of children with vasospasm shows elevation of middle cerebral artery velocities; this technique allows sequential monitoring of these patients. Central cerebral arterial narrowing and irregularity due to spasm can be demonstrated with MRA, CTA, or conventional angiography.
Figure 20–14
Ruptured congenital saccular aneurysm.
A. An unenhanced CT image of a 5-week-old infant shows intraparenchymal and subarachnoid hemorrhage on the right. The oval blood-filled aneurysm (arrow) adjacent to the hematoma is isoattenuating to brain. B. Flowing blood in the aneurysm (arrow) causes elevated signal intensity on this T1-weighted image. Blood is present in the dependent portion of the dilated left lateral ventricle. C. A diffusion-weighted image demonstrates manifestations of ischemia throughout a large portion of the right cerebral hemisphere. D. An anterior-superior view of a CTA image shows the bilobed enhancing aneurysm to be located adjacent to the right middle cerebral artery (RMCA), which was the vessel of origin. ACA = anterior cerebral artery.


The term stroke refers to acute occlusion or rupture of a cerebral artery or vein, resulting in focal cerebral damage and neurological deficits that persist for at least 24 hours. Strokes are ischemic, hemorrhagic, or both. Ischemic strokes, or brain infarctions, are arterial or venous. Thromboembolic occlusion of a cerebral artery is the usual cause of an arterial infarction. Venous strokes are due to sinovenous thrombosis. The 2 major mechanisms of hemorrhagic strokes are bleeding into acutely ischemic brain tissue and rupture of an intracranial artery.90
Each year, over half a million individuals in United States suffer a stroke. The incidence of stroke is approximately 3 per 100,000 children per year. About half of pediatric strokes are hemorrhagic. About three-quarters of pediatric strokes are arterial and one-quarter venous. Potential predisposing conditions for pediatric stroke include sickle cell disease, cardiac lesions, cerebral vascular malformations, hematological disorders, neurocutaneous syndromes (e.g., neurofibromatosis), various chromosomal abnormalities (e.g., Down syndrome), primary vasculopathies (e.g., moyamoya), trauma (e.g., carotid or vertebral dissection), and head/neck infections. Up to half of children with stroke have no known underlying condition.91–94
Most strokes cause localized brain ischemia. Multiple factors influence the clinical and neuroimaging manifestations of the insult. These include the volume and location of brain tissue involved, the rapidity of onset of ischemia, the severity of ischemia, the time course, the age of the patient, the adequacy of collateral circulation, the presence of preexisting brain pathology, and the institution of therapeutic intervention. Brain hypoxia/ischemia can be focal or global, acute or chronic, and episodic or recurrent.
The typical clinical presentation of stroke varies with patient age. Infants who have suffered a stroke in utero may present with early onset of hand dominance or other signs of unilateral weakness. Strokes in the perinatal period often lead to seizures, with subacute development of hemiparesis. Older children with stroke usually have similar presentations as do adults, with an acute onset of a focal neurological deficit, dysarthria, and/or aphasia; seizures may or may not occur. In addition to stroke, the differential diagnosis of acute hemiplegia in a child includes Todd paralysis (transient hemiparesis following a seizure) and complicated migraine. Over half of children with stroke suffer lifelong cognitive or motor disabilities.95,96
Hemorrhagic stroke often has a precipitous clinical presentation, with neurological alterations due to localized brain compression and elevated intracranial pressure. Common manifestations include impaired mental status, severe headache, bradycardia, and emesis. In general, the survival rate is worse with hemorrhagic strokes than with ischemic strokes.
Arterial infarction of the brain is an important cause of CNS damage in children of all ages. Major cerebral infarction in the newborn occurs with an estimated prevalence of 1 in 4000 births. The clinical manifestations of perinatal or neonatal brain infarction are often nonspecific. Common findings include seizures, hypotonia, and lethargy. Some neonatal strokes are clinically silent. Fetal stroke may lead to early hand preference in infancy or unilateral weakness. Older infants and children with arterial infarction usually experience an acute neurological event, similar to the adult presentation. The key factors in the long-term prognosis are the volume of brain involved, the location in the brain, the adequacy of collateral circulation, and the age of the patient at the time of the event. Other factors being equal, functional recovery is much better in young infants following a stroke than in older children.97–99
Potential causes of cerebral infarction include emboli (often cardiogenic), sickle cell disease, prior varicella infection, coagulation disorders, congenital vascular malformations, aneurysm, vasculopathy (e.g., moyamoya, vascular fibrous dysplasia, and PHACES syndrome), metabolic disorders (e.g., MELAS syndrome, organic aciduria, and lysosomal storage disorders), trauma (e.g., child abuse, penetrating neck injuries, and vascular dissection), vasculitis, maternal drug use (fetal stroke), meningitis, and intracranial or neck neoplasm. Many infarcts are idiopathic. Perivascular space inflammation in patients with meningitis can cause a vasculitis that progresses to venous thrombosis or arterial thrombosis. The most common causes of ischemic stroke in the pediatric population as a whole are sickle cell disease and vasculopathy. An epidemiological study in the central Maryland and Washington, DC area reported an incidence of 0.6 per 100,000 children per year for ischemic stroke in individuals less than 15 years of age. For individuals with sickle cell disease, the incidence was 280 per 100,000 children per year.16,93,100
Ischemic vascular accidents most often occur in the middle cerebral artery territory. This is particularly true for children who suffer strokes due to thromboembolism, such as those with right-to-left shunts or thrombogenic pathology in the left heart. The basal ganglia are also susceptible to ischemic injury because the blood supply derives from small end arteries. The blood supply of the basal ganglia consists of the medial lenticulostriate arteries (which supply the head of the caudate nucleus and a portion of the anterior limb of the internal capsule) and the lateral lenticulostriate arteries (which supply the lentiform nucleus and parts of the caudate nucleus and internal capsule). The medial lenticulostriate arteries arise from the A1 segment of the anterior cerebral artery. The recurrent artery of Heubner is an important branch of the medial lenticulostriate arteries; this vessel can arise from the A1 or A2 segments of the anterior cerebral artery. The lateral lenticulostriate arteries arise from the superior portion of the M1 segment of the middle cerebral artery.
Unlike most tissues that can mobilize energy stores from fat and glycogen, the brain does not have an energy buffer for protection when challenged with ischemia. Ischemia causes cell membrane pump failure and a resultant cascade of fluid shifts that ultimately leads to cell death. The ischemic threshold occurs at about one-fourth of normal blood flow. An orderly sequence of events occurs at the tissue level of the brain in response to ischemia. The initial consequence is cytotoxic edema due to cell membrane pump failure. Vasogenic edema develops subsequently. By approximately 5 to 7 days after the initial insult, there is loss of integrity of the blood-brain barrier due to injury of vessels. Petechial hemorrhage, laminar necrosis, and increased contrast enhancement on neuroimaging studies may be present at this stage. In the subacute phase, edema diminishes and macrophages process the necrotic tissue, resulting in encephalomalacia and gliosis. Eventually, there is restoration of the blood-brain barrier.
During the first several hours after the onset of symptoms from an acute infarction, CT is usually normal. Occasionally, there is abnormal increased attenuation within an intracranial artery, usually the proximal segment of the middle cerebral artery, due to slow blood flow or the presence of a thrombus; this is termed the “hyperdense vessel sign.” The “MCA dot sign” refers to hyperattenuating thromboembolism within a branch of the middle cerebral artery in the sylvian fissure.
Between 6 and 12 hours after the event, there is usually sufficient edema to produce alterations in the attenuation characteristics of the ischemic brain on CT. Subtle findings that reflect the presence of cytotoxic edema during the early phase of an ischemic stroke include indistinctness of the gray matter-white matter junction, loss of the usual slightly higher attenuation of the insular cortex just superficial to the external and extreme capsules (the “insular ribbon sign”), and loss of the normal attenuation differences between the globus pallidus and putamen with respect to adjacent white matter. With ischemia in the distribution of the middle cerebral artery, local mass effect may produce effacement of sulci or narrowing of the sylvian fissure. Loss of the normal high attenuation appearance of the basal ganglia (due to cytotoxic edema) as an early CT manifestation of cerebral infarction is termed the “disappearing basal ganglia sign.” (Changes in basal ganglia attenuation, usually bilateral, can also occur with a variety of other insults, including global brain hypoperfusion, poisoning [e.g., methanol, cyanide], severe hypoglycemia, lactic acidosis, Leigh disease, and mitochondrial myopathy.) Patients with identifiable parenchymal brain abnormalities on CT within 3 hours of the onset of symptoms tend to have more severe strokes and worse outcomes than those with normal CT examinations during this early period.101–103
In the subacute phase of an arterial infarct, the involved portion of the brain is hypoattenuating on CT. Classic cortical infarcts are often wedge-shaped and involve both cortical gray matter and the underlying white matter. Mass effect from edema usually diminishes in severity after 5 to 7 days. Within several days of the injury, petechial hemorrhage or microcalcification in the cortex may develop, resulting in a laminar necrosis pattern (high attenuation along the cortical surface). There is also prominent contrast enhancement at the site of an arterial infarct during the subacute phase. The greatest risk for frank hemorrhagic transformation of an infarct is between the fourth and seventh days after the stroke.
MRI provides greater sensitivity and specificity than CT for the imaging evaluation of acute brain infarction. Diffusion-weighted MR images reflect manifestations of ischemia as early as 20 to 30 minutes after the insult. An early physiological consequence of ischemia is failure of cell membrane sodium-potassium pumps. This leads to influx of water into cells from the interstitium; that is, cytotoxic edema. This intracellular water has diminished diffusion in comparison to normal extracellular interstitial water. This is predominantly due to interaction of the water molecules with intracellular structures. Additional factors that serve to restrict water molecule motion are compression and increased tortuosity of the extracellular spaces (due to cellular swelling), as well as elevated intracellular viscosity. Because of the relatively high water content of brain in young infants, careful inspection of apparent diffusion coefficient (ADC) map images is essential to detect subtle or early edema. “Pseudonormalization” of diffusion-weighted images often occurs between 7 and 14 days after the insult, as the cell walls in the area of infarction break down and diffusion increases. However, standard T2-weighted and FLAIR images are usually abnormal by this time.104,105
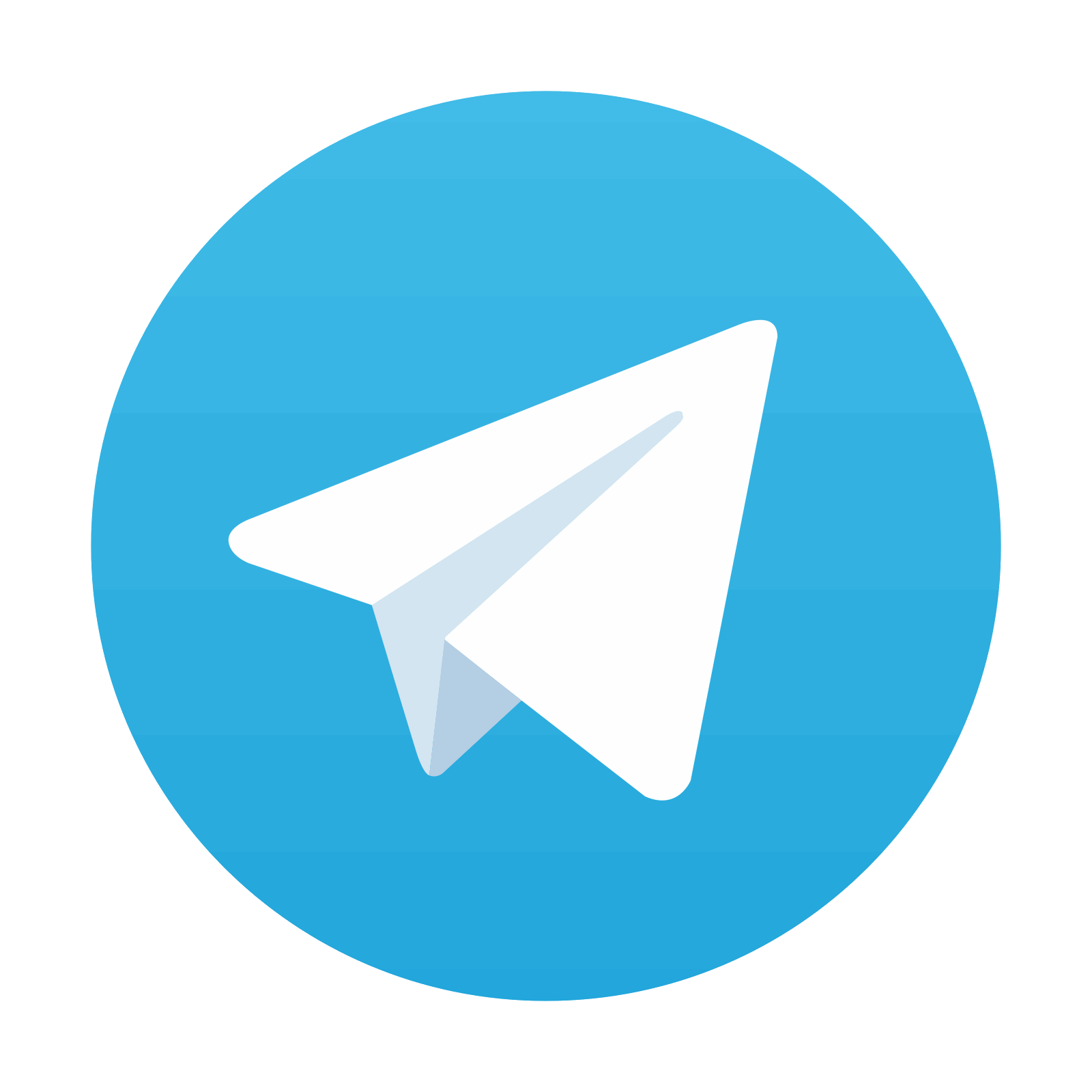
Stay updated, free articles. Join our Telegram channel

Full access? Get Clinical Tree
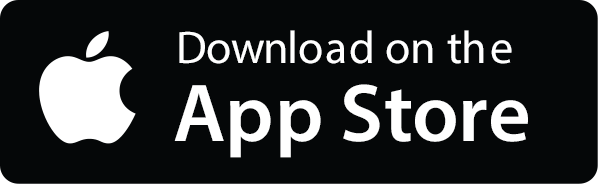
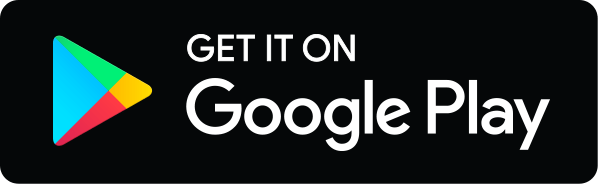